Cell Biology Lecture Notes PDF
Document Details
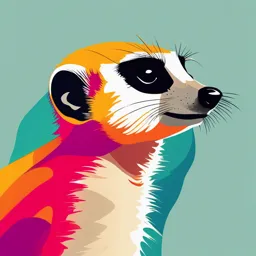
Uploaded by SpiritualHonor
College of Medicine
Tags
Summary
These lecture notes discuss cell membrane structure and functions, including vesicular transport mechanisms like endocytosis and exocytosis. They cover the fluid mosaic model and the role of phospholipids, proteins, and carbohydrates in cell membranes. The notes also briefly introduce different types of endocytosis.
Full Transcript
1 Cell membrane& vesicular Transport ILOs By the end of this lecture, students will be able to 1. Relate the molecular structure of membrane lipids to its different membrane functions. 2. Correlate the variable peripheral & integral membrane molecules to membrane function. 3. Int...
1 Cell membrane& vesicular Transport ILOs By the end of this lecture, students will be able to 1. Relate the molecular structure of membrane lipids to its different membrane functions. 2. Correlate the variable peripheral & integral membrane molecules to membrane function. 3. Interpret the diagrammatic presentation of the fluid mosaic model 4. Interpret endocytosis & exocytosis as vital processes for transport across the cell membrane. 5. Differentiate between types of endocytosis in normal and pathological conditions. Cell Components The cell is composed of two basic parts: cytoplasm (Gr. kytos, cell, + plasma, thing formed) and nucleus. Individual cytoplasmic components are usually not clearly distinguishable in common hematoxylin and eosin-stained preparations; the nucleus, however, appears intensely stained dark blue or black. (Why?) Cytoplasm The outermost component of the cell, separating the cytoplasm from its extracellular environment, is the plasma membrane (plasmalemma). However, even if the plasma membrane is the external limit of the cell, there is a continuum between the interior of the cell and extracellular macromolecules. The cytoplasm is composed of a matrix, or cytosol, in which are embedded the organelles, the cytoskeleton, and deposits of carbohydrates, lipids, and pigments. Higher levels of organization Plasma Membrane All eukaryotic cells are enveloped by a limiting membrane composed of phospholipids, cholesterol, proteins, and chains of oligosaccharides covalently linked to phospholipids and protein molecules. The cell, or plasma, membrane functions as a selective barrier that Page 1 of 5 regulates the passage of certain materials into and out of the cell and facilitates the transport of specific molecules. One important role of the cell membrane is to keep constant the intracellular milieu, which is different from the extracellular fluid. Membranes also carry out a number of specific recognition and regulatory functions (to be discussed later), playing an important role in the interactions of the cell with its environment. Membranes range from 7.5 to 10 nm in thickness and consequently are visible only in the electron microscope (so, what is visible on the light microscope?) Molecular structure of the cell membrane (Figure 2) 1- Membrane phospholipids. Structure: two long, nonpolar (hydrophobic) hydrocarbon chains linked to a charged (hydrophilic) head group. (Why it appears black in electron micrograph? Is it a single line?) o Organization: the hydrophobic (nonpolar) chains directed toward the centre of the membrane and the hydrophilic (charged) heads directed outward. o Cholesterol is also a constituent of cell membranes. Role of cholesterol; (It will be discussed later with fluidity of the membrane). Note: ✔ This represents the fluid mosaic model of the cell membrane. ✔ The lipid composition of each half of the bilayer could be different according to its functional role. ✔ The trilaminar appearance are characteristic of all the internal cellular membranes (eg. Nuclear, mitochondrial, and endoplasmic reticulum) as well as the plasma membrane. ✔ Some of the lipids, known as glycolipids, possess oligosaccharide chains that extend outward from the surface of the cell membrane and thus contribute to lipid asymmetry. (Figure 2) 2- Proteins, About 50% of the plasma membrane components Types: Page 2 of 5 o Integral proteins are directly incorporated within the lipid bilayer. Some integral proteins span the membrane one or more times, from one side to the other. Other proteins are large enough to extend across the two lipid layers and protrude from both membrane surfaces (transmembrane proteins). Integrins are transmembrane proteins that are linked to cytoplasmic cytoskeletal filaments and to extracellular molecules. Through these linkages there is a constant exchange of influence, in both ways, between the extracellular matrix and the cytoplasm. o Peripheral proteins exhibit a looser association with membrane surfaces. They may protrude from either the outer or inner surface. o Organelle-specific membranes proteins confer unique functions on certain organelles. Correlate the functional forms of integral membrane protein in Fig. 2 with its type. 3- The carbohydrate moieties of glycoproteins and glycolipids exoplasmic domain; they are important components of specific molecules called receptors that participate in important interactions such as cell adhesion, recognition, and response to protein hormones. As with lipids, the distribution of membrane proteins is different in the two surfaces of the cell membranes. Therefore, all membranes in the cell are asymmetric. Glycocalyx (cell coat) In the electron microscope the external surface of the cell shows a fuzzy carbohydrate-rich region called the glycocalyx. This layer is composed of carbohydrate chains linked to membrane proteins and lipids and of cell-secreted glycoproteins and proteoglycans. Functional significance of glycocalyx The glycocalyx has a role in cell recognition and attachment to other cells and to extracellular molecules. Transport across the cell membrane Movement of molecules and ions across membranes occurs by different mechanisms including: a. Passive transport (simple and facilitated diffusion). b. Active transport (pump and cotransport carrier). (Fig 3) c. Bulk movement of materials into and out of the cells (endocytosis & exocytosis) which will be discussed in this section. Mass transfer of material also occurs through the plasma membrane. This bulk uptake of material is known as endocytosis. The corresponding name for release of material in bulk is exocytosis. However, at the molecular level, exocytosis and endocytosis are different processes that utilize different protein molecules. Page 3 of 5 Endocytosis: (Gr. endon, within, + kytos, cell) The process whereby a cell ingests macromolecules, particulate matter, and other substances from the extracellular space. Mechanisms of endocytosis: (Figure 3) 1. Phagocytosis (cell eating) It is a nonselective process of engulfing larger particulate matter, such as microorganisms, cell fragments, and degenerated cells (e.g., defunct red blood cells), by specialized cells known as phagocytes. The engulfed material is enclosed in a vesicle called the phagosome. 2. Pinocytosis Nonselective process where small invaginations of the cell membrane form and entrap extracellular fluid forming a pinocytotic vesicle that pinch off from the cell surface and may fuse with lysosomes (see the section on Lysosomes later in this chapter). Receptor-Mediated Endocytosis It is a selective process that involves engulfment of macromolecules. This process depends on interaction between two structures: ⮚ Receptor proteins (cargo receptors) in the cell membrane. Cargo receptors are transmembrane proteins. ⮚ Macromolecules (ligands), (What is ligand?) such as low-density lipoproteins and protein hormones that bind with its receptor on the cell membrane. The receptors are either originally widely dispersed over the surface or aggregated in special regions called coated pits. Binding of the ligand to its receptor causes widely dispersed receptors to accumulate in coated pits. The coated pit invaginates and pinches off from the cell membrane, forming a coated vesicle that carries both the ligand and its receptor into the cell. Page 4 of 5 Note: Caveolae is a special form of endocytosis where the coating protein is caveolin (will be discussed later) Fate of the endocytotic vesicle (Figure 4) ⮚ The coated vesicles soon lose their clathrin coat and fuse with early endosomes near the periphery of the cell, a system of vesicles and tubules located in the cytosol near the cell surface. The clathrin molecules separated from the coated vesicles are moved back to the cell membrane to participate in the formation of new coated pits. ⮚ If some contents of the early endosome require degradation, they are transferred deeper in the cytoplasm into the late endosome. This similar set of tubules and vesicles, located deeper in the cytoplasm near the Golgi apparatus, helps to prepare its contents for eventual destruction by lysosomes. ⮚ The membranes of all endosomes contain ATP-linked H+ pumps that acidify the interior of the endosomes by actively pumping H+ ions into the interior of the endosome. Fate of the Endosome contents ⮚ Receptors that are separated from their ligand by the acidic pH of the early endosomes may return to the cell membrane to be reused. For example, low-density lipoprotein receptors are recycled several times. ⮚ The ligands typically are transferred to late endosomes. However, some ligands are returned to the extracellular milieu to be used again. An example of this activity is the iron-transporting protein transferrin. Occasionally, both the receptor and the ligand (e.g., epidermal growth factor and its receptor) are transferred to the late endosome, and then to a lysosome, for eventual degradation. (Fig 4) Fig. 4 Formation of early & late endosomes Page 5 of 5 2 Lipid Structure & functions in bio-membranes: ILOs By the end of this lecture, students will be able to 1. Describe structure-function relationship of phospholipids 2. Describe the structure-function relationship of cholesterol 3. Interpret their importance in controlling cell membrane function Membranes can be regarded as a “fluid mosaic model” the fluid nature of the membranes allows greater flexibility to the cell than it would if the membranes were rigid. It also allows the motion of membrane components, required for some types of membrane transport as membranes are dynamic structures. The cell membrane is an asymmetric structure with the two sides of membrane being structurally and functionally different. This difference is due to the difference in composition and orientation of lipids, proteins and carbohydrates. ❖ How can this membranes’ structure serve its function? o Phospholipids: Phospholipids are ionic compounds. Like fatty acids (FA), phospholipids are amphipathic in nature. That is, each has a hydrophilic head, which is the phosphate group plus whatever alcohol is attached to it and a long, hydrophobic tail containing FA. There are two classes of phospholipids, those that have glycerol as a backbone (GLYCEROPHOSPHLIPIDS or PHOSPHOGLYCERIDES ) and those that have sphingosine as a backbone (SPHINGOPHOSPHOLIPIDS). ✓ Glycerophosphlipids (Phosphoglycerides): Of the two major phospholipid classes present in membranes, phosphoglycerides are more common and consist of a glycerol backbone to which are attached two fatty acids in ester linkage and a phosphorylated alcohol. Phosphoglycerides structurally similar to Figure (10-1): Triglycerides Vs Phospholipids triglycerides except that on the third carbon of glycerol instead of a fatty acid there is a phosphate attached to a polar head group. The fatty acid constituents are usually even-numbered carbon molecules, most commonly containing 16 or 18 carbons. They can be saturated or unsaturated with one or more cis double bonds. Page 1 of 5 The simplest phosphoglyceride is Phosphatidic acid, which is 1,2- diacylglycerol 3-phosphate, a key intermediate in the formation of other phosphoglycerides In most phosphoglycerides present in membranes, the phosphate is esterified to an alcohol such as ethanolamine and choline or glycerol. Lecithin (phosphatidyl choline): - It is the most abundant phosphoglyceride. The phosphorylated alcohol here is choline. - It represents a large proportion of the body’s store of choline. - Choline is important in nervous transmission. - Lecithin is also a major constituent of the surfactant preventing adherence, due to surface tension of the inner surfaces of the lungs. - Its absence from the lungs of premature infants causes respiratory distress syndrome. - There is a certain enzyme called lecithinase enzyme present in the venum of cobra. It splits the unsaturated FA from lecithin in cell membrane giving rise to lysolecithin. This substance can produce lysis of red cell membrane and haemolysis. Cephalin (phosphatidyl ethanolamine): It is another abundant phosphoglycerols (phosphoglycerides) which is also found in cell membranes. The base is ethanolamine. Cephalin is one of the important blood clotting factors. ✓ Sphingophospholipids: The second major class of phospholipids is composed of Sphingomyelin, which contains a sphingosine backbone rather than glycerol. A fatty acid (usually longer and less saturated than that of phophoglycerides) is attached by an amide linkage to the amino group of sphingosine, forming ceramide. The primary hydroxyl group of sphingosine is esterified to phosphorylcholine, forming sphingomyelin. As the name implies, sphingomyelin is prominent in myelin sheaths. They play a major role in signal transmission and cell recognition. Figure(10-2): Structure of Sphingomyein Page 2 of 5 ❖ What is the Structure-Function Relationship of phospholipids? Phospholipids are the predominant lipids of cell membranes. In membranes, the hydrophilic (polar) head of the phospholipid extends outward, interacting with the intracellular or extracellular aqueous environment. While, the hydrophobic portion of a phospholipid molecule is associated with the nonpolar portions of other membrane constituents, such as glycolipids, proteins, and cholesterol. The hydrophobic tail containing the fatty acids plays a major role in maintaining the fluidity of the Figure (10-3): Structure of membrane membrane, unsaturated fatty acids add to membrane fluidity. These unsaturated fatty acids form kinks that increase the space between the phospholipids increasing the membrane’s fluidity. In addition, the increased space allows certain small molecules to cross the membrane quickly and easily. In the same time, they form weak non-covalent bonds with each other, holding the bilayer together. Therefore, phospholipid molecules are responsible for the semi-permeability of the cell membrane. They prevent passage of water soluble substances and ions; however they allow passage of small non-polar and fat soluble substances. The amounts and fatty acid compositions of the various phospholipids vary among the different cellular membranes. Clinical Implications: There are many diseases associated with problems in the ability of the phospholipid bilayer to perform these functions. One of these is Alzheimer’s disease, characterized by brain shrinkage and memory loss. One idea explaining why Alzheimer’s disease occurs is the formation of plaque sticking to the phospholipid bilayer of the brain neurons. Interference with the membrane’s phospholipids block communication between the brain neurons, eventually leading to neuron death and in turn causing the symptoms of Alzheimer’s, such as poor short-term memory. One of the facts about the Fluid-Mosaic membrane model is that the components of the bilayers are free to move. Phospholipids have several types of movements, rotational where phospholipid rotates on its axis to interact with its immediate neighbors. lateral, where the phospholipid moves around in one leaflet. Finally, it is possible for phospholipids to Page 3 of 5 move between both leaflets of the bilayer in transverse movement, in a “flip-flop” manner, these movements are important for cell signalling. o Cholesterol: Compounds containing 27 carbon structures with four rings. A Cholesterol is a very important steroid to the body. When cholesterol binds to a fatty acid it forms a Cholesteryl ester. Cholesteryl esters have a lower solubility in water due to their increased hydrophobicity. In the memebrane cholesterol can be present on it’s own or combined B with proteins Figure (10-4): A: Cholesterol Cholesterol Helps control the Fluidity of Cell Membranes: B:Cholesteryl - Cholesterol is an amphipathic molecule, meaning, like Ester phospholipids, it contains a hydrophilic and a hydrophobic portion. - The cholesterol molecules are randomly distributed across the phospholipid bilayer. - Cholesterol's hydroxyl (OH) group aligns with the phosphate heads of the phospholipids. The remaining portion of it tucks into the fatty acid portion of the membrane. - Because of the way cholesterol is shaped, part of the steroid ring is closely attracted to part of the fatty acid chain on the nearest phospholipid. This helps slightly immobilize the membrane (decrease the fluidity) and make it less soluble to very small water- soluble molecules that could otherwise pass through more easily. Figure (10-5): Cholesterol in - Without cholesterol, cell membranes would membrane be too fluid, not firm enough, and too permeable to some molecules. - However, in cold temperature Cholesterol increases the fluidity as it helps separate the hydrophobic tails of phospholipids so that the fatty acid chains can't come together and cyrstallize. Cholesterol Helps Secure Important Proteins in the Membrane: - The plasma membrane contains many proteins that perform important functions, because certain proteins' size or shape requires a thicker phospholipid bed to sit in, and because certain proteins need to stick together to function properly, the fluidity of the cell membrane where the molecules are constantly moving randomly, could pose a problem. Page 4 of 5 - In these areas the plasma membrane contains high concentrations of cholesterol and glycosphingolipids which aggregate more making these parts of the membrane thicker, and making it ideal for accommodating certain proteins. - The previous association of cholesterol and glycosphingolipids together with proteins form “lipid rafts” which are microdomains of the plasma membrane that function to organize and regulate membrane signalling. - A special type of lipid rafts is the “Caveolae” which are flask like invaginations in the plasma membrane (while lipid rafts are generally flat regions) they are mainly present in fat cells and muscle cells. - Caveolae plays an important role in cell signalling, they can bud from the plasma membrane to form a vesicle for endocytosis (cellular process in which substances are brought into the cell) or can flatten into the membrane to help cells with stand mechanical stress. Page 5 of 5 3 Transport across the cell membrane ILOs By the end of this lecture, students will be able to 1. Demonstrate the different transport mechanisms across the cell membrane 2. Compare movement through channels to movement by simple or facilitated diffusion 3. Differentiate between primary and secondary active transport Substances generally move across cellular membranes via transport processes that can be classified as passive or active, depending on whether they require cellular energy. In passive process, a substance moves across the cell membrane along any type of gradient without expenditure of energy. On the other hand, in active transport, the transport of substances takes place against any type of gradient by the utilization of energy. Passive transport The transport of the substances along the concentration or electrochemical gradient or both is called passive transport or diffusion. The substances are transported from region of higher concentration to a lower concentration. This type of transport doesn’t require energy but it may needs the help of carrier proteins of the cell membrane. 1)-Simple Diffusion Simple diffusion is a passive process in which substances move freely through the lipid bilayer of the plasma membranes of cells without the help of membrane transport proteins. The lipid layer is only permeable to lipid- soluble substances such as oxygen, carbon dioxide, and nitrogen gases; fatty acids; steroids; and fat-soluble vitamins (A, D, E, and K). Small, uncharged polar molecules such as water, urea, and small alcohols also pass through the lipid bilayer by simple diffusion. 2)-Facilitated Diffusion a)-Channel-mediated facilitated diffusion: A solute moves down its concentration gradient across the lipid bilayer through a membrane channel. Most membrane channels are ion channels, integral transmembrane proteins that allow passage of small, inorganic ions. Water soluble substances can diffuse through protein channels. The characteristic feature of these protein channels is the selective permeability. That is, each channel can permit only one type of ion to pass through it such as sodium channels, potassium channels, etc… Diffusion of ions through channels is generally slower than free diffusion through the lipid bilayer because channels occupy a smaller fraction of the membrane’s total surface area than lipids. Some of protein channels are continuously opened (leak channels) and most of channels are always closed (gated channels). The gated channels are opened only when required and it is of 2 types: Page 1 of 4 Voltage gated channels: these channels open when there is a change in the electrical potential. Ligand gated channels: are opened by binding to a ligand as some hormonal substances. b)-Carrier-mediated facilitated diffusion: The water soluble substances having larger molecules cannot diffuse through the protein channels. They diffuse through cell membrane with the help of carrier proteins (transporters). These transporters are proteins that are part of the cell membrane. In carrier-mediated facilitated diffusion, a carrier moves a solute down its concentration gradient across the plasma membrane. The solute binds to a specific carrier on one side of the membrane and is released on the other side. Binding of the solute with the carrier leads to changes in the shape of the carrier. This physical change propels the solute into the interior of the cell. Substances that move across the plasma membrane by carrier mediated facilitated diffusion include glucose, fructose, galactose, amino acids and some vitamins. Figure 1: The difference between simple and facilitated diffusion. 3)-Osmosis Osmosis is a type of diffusion in which there is net movement of water or any other solvent through a semipermeable membrane. Osmosis occurs only when a membrane is permeable to water but is not permeable to certain solutes. Like the other types of diffusion, osmosis is a passive process. In living systems, the solvent is water, which moves by osmosis across plasma membranes from an area of higher water concentration to an area of lower water concentration. Another way to understand this idea is to consider the solute concentration: In osmosis, water moves through a selectively permeable membrane from an area of lower solute concentration to an area of higher solute concentration. During osmosis, water molecules pass through a plasma membrane in two ways: (1) by moving between neighboring phospholipid molecules in the lipid bilayer via simple Page 2 of 4 diffusion, and (2) by moving through aquaporins, integral membrane proteins that function as water channels. Active transport It is the movement of substances against the chemical or electrical or electrochemical gradient. Active transport requires energy and a carrier protein. Active transport is considered an active process because energy is required for carrier proteins to move solutes across the membrane against a concentration gradient. Two sources of cellular energy can be used to drive active transport: (1) Energy obtained from hydrolysis of adenosine triphosphate (ATP) is the source in primary active transport; (2) energy stored in an ionic concentration gradient is the source in secondary active transport. Figure 2: The difference between passive and active transport 1)-Primary Active Transport This is the type of active transport in which the energy is liberated directly from hydrolysis of ATP. The carrier protein has ATPase activity which causes breakdown of ATP. The energy derived from hydrolysis of ATP changes the shape of a carrier protein, which “pumps” a substance across a plasma membrane against its concentration gradient. Indeed, carrier proteins that mediate primary active transport are often called pumps. The most prevalent primary active transport mechanism expels sodium ions (Na") from cells and brings potassium ions (K") in. Because of the specific ions it moves, Page 3 of 4 this carrier is called the sodium–potassium pump. Because a part of the sodium-potassium pump acts as an ATPase, an enzyme that hydrolyzes ATP, another name for this pump is Na!–K! ATPase. All cells have thousands of sodium–potassium pumps in their plasma membranes. 2)-Secondary Active Transport This is the type of active transport in which the substance moves against its electrochemical gradient. However the energy supplied for this transport doesn’t come directly from ATP, but it comes from the movement of another substance along its electrochemical gradient. When Na+ is transported by a carrier protein passively, another substance is also transported by the same protein simultaneously, either in the same direction of Na+ movement (co-transport) or in the opposite direction (counter- transport). The energy stored in a Na+ concentration gradient is used to drive other substances across the membrane against their own concentration gradients such as glucose and amino acids. A carrier protein (transporter) simultaneously binds to Na" and another substance and then changes its shape so that both substances cross the membrane at the same time. Types of carrier: Symporters: the carriers move two substances in the same direction at the same time as Na+ and glucose. Antiporters: the carriers move two substances in opposite directions across the membrane at the same time as Na+ and H+ in renal epithelial cells. Figure 3: Types of active transport Page 4 of 4 4 Cellular Homeostasis-Homeostatic control system ILOs By the end of this lecture, students will be able to 1. Relate Feedback /response loop to body homeostasis 2. Differentiate between +ve and –ve feedback mechanisms 3. Apply the process of -ve feedback loop on some homeostatic regulatory mechanisms as blood glucose level& body temperature 4. Correlate the distribution of body water and electrolytes among different compartments to the health and disease 5. Detect the different factors involved in body homeostasis 6. Integrate functions of different body systems serving the body homeostasis 7. Distinguish between osmolarity , osmolality , osmotic pressure and tonicity 8. Analyze the effect of different solutions on cell membrane 9. Locate the different ions in both ECF and ICF compartments 10. Relate the cell permeability and transport mechanisms to the cell membrane potential Homeostasis Homeostasis means the maintenance of constant internal environment. Control of Homeostasis Homeostasis in the human body is continually being disturbed. Some disruptions come from the external environment in the form of physical insults such as the intense heat day or a lack of enough oxygen. Other disruptions originate in the internal environment, such as change of blood glucose level. Fortunately, the body has many regulating systems that can usually bring the internal environment back into balance. Most often, the nervous system and the endocrine system, working together or independently, provide the needed corrective measures. Both means of regulation, however, work toward the same end, usually through negative feedback systems. Feedback Systems The body can regulate its internal environment through many feedback systems. A feedback system or feedback loop is a cycle of events in which the status of a body condition is monitored, evaluated, changed, re-monitored, reevaluated, and so on. Each monitored variable, such as body temperature, blood pressure, or blood glucose level, is termed a controlled condition. Any disruption that changes a controlled condition is called a stimulus. A feedback system includes three basic components: a receptor, a control center, and an effector (figure 1). 1. A receptor is a body structure that monitors changes in a controlled condition and sends input (nerve impulse or chemical signals) to a control center. This pathway is called an afferent pathway. 2. A control center in the body, for example, the brain, sets the range of values within which a controlled condition should be maintained (set point), evaluates the input it receives from receptors, and generates output commands when they are needed. Output from the control center typically occurs as nerve impulses, or hormones or other chemical signals. This pathway is called an efferent pathway. Page 1 of 9 3. An effector is a body structure that receives output from the control center and produces a response or effect that changes the controlled condition. In a feedback system, the response of the system “feeds backward to change the controlled condition in some way, either negating it (negative feedback) or enhancing it (positive feedback). Negative Feedback Systems A negative feedback system reverses a change in a controlled condition. If the activity of a particular system is increases, the regulatory mechanism will immediately reduce the activity. For example, consider the regulation of blood pressure. If the blood pressure (controlled condition) rises due to Figure (1): The feedback loop of homeostasis any stimulus, the following sequence of events occurs. Baroreceptors (the receptors), pressure- sensitive nerve cells located in the walls of certain blood vessels, detect the higher pressure. The baroreceptors send nerve impulses (input) to the brain (control center), which interprets the impulses and responds by sending nerve impulses (output) to the heart and blood vessels (the effectors). Heart rate decreases and blood vessels dilate (widen), which cause BP to decrease (response). This sequence of events quickly returns the controlled condition—blood pressure—to normal, and homeostasis is restored. Notice that the activity of the effector causes BP to drop, a result that negates the original stimulus (an increase in BP). This is why it is called a negative feedback system. Page 2 of 9 Example of negative feedback mechanism: Regulation of body temperature: Body temperature is regulated by negative feedback system (figure 2). The stimulus is when the body temperature exceeds or decreases below 37°C (set point). The receptors are the nerve cells with endings in the skin and brain, the control center is the temperature regulatory center in the brain, and the effector is the sweat glands and blood vessels throughout the body. If the body temperature rises above 37.0 ∘C, a negative feedback loop will act to bring it back down towards the set point. High temperature will be detected by receptors in the skin and brain. These receptors send signals to a temperature-regulatory control center in the hypothalamus. The control center checks our current temperature and compares it with the set point. Then it sends signals to the effectors (sweat glands and blood vessels). Blood vessels dilate with increase blood flow to skin which speed up heat loss into surroundings. Increase sweating will also help to decrease temperature through evaporation of sweat from skin. Heavy breathing can also increase heat loss. On the other hand, if body temperature decreases below its set point, low temperature will be detected by receptors in the skin and brain which send signals to the control center in the hypothalamus. This center sends signals to the effectors (sweat glands and blood vessels). Blood vessels constricts with decrease blood flow to skin which decrease heat loss into surroundings. Decrease sweating will also help to conserve body heat. The nervous system also sends signals to muscles to contract involuntarily (shivering) to generate heat. Figure (2) : Regulation of temperature by the negative feedback loop Page 3 of 9 Positive Feedback Systems Unlike a negative feedback system, a positive feedback system tends to strengthen or reinforce a change in one of the body’s-controlled conditions. The control center still provides commands to an effector, but this time the effector produces a physiological response that adds to or reinforces the initial change in the controlled condition. The action of a positive feedback system continues until it is interrupted by some mechanism. Normal childbirth (figure 3) and Suckling reflex provide good examples of positive feedback systems. These examples suggest some important differences between positive and negative feedback systems. Because a positive feedback system continually reinforces a change in a controlled condition, some event outside the system must shut it off. If the action of a positive feedback system is not stopped, it can “run-away” and may even produce life- threatening conditions in the body. The action of a negative feedback system, by contrast, slows and then stops as the controlled condition returns to its normal state. Usually, positive feedback systems reinforce conditions that do not happen very often, and negative feedback systems regulate conditions in the body that remain fairly stable over long periods. Figure (3): childbirth positive feedback loop Factors involved in homeostasis: 1. Maintenance of pH 2. Regulation of temperature 3. Maintenance of water balance 4. Maintenance of electrolyte balance 5. Supply of nutrients, oxygen, enzymes and hormones 6. Removal of metabolic and other waste products Page 4 of 9 Body Fluids compartments The cells of the human body live in a carefully regulated fluid environment. The fluid inside the cells, the intracellular fluid (ICF), occupies what is called the intracellular compartment, and the fluid outside the cells, the extracellular fluid (ECF), occupies the extracellular compartment. The barriers that separate these two compartments are the cell membranes. For life to be sustained, the body must rigorously maintain the volume and composition of the intracellular and extracellular compartments. To a large extent, such regulation is the result of transport across the cell membrane. The intracellular and extracellular fluids Total body water is the sum of the intracellular and extracellular fluid volumes. In a normal young adult male, it is about 60% of total body weight and in a young adult human female, it is about 50% of total body weight. Total body water accounts for a lower percentage of weight in females because they typically have more adipose tissue, and fat cells have a lower water content than muscle does. In thin persons, water content is more than in obese persons. In old age, water content is decreased due to increase in adipose tissue. The total quantity of body water in an average human being weighting about 70 kg is about 40 liters (60%). It is distributed into two major compartments namely: 1) - Intracellular fluid (ICF) forming 55% of total body water (22 liters) 2) - Extracellular fluid (ECF) forming 45% of the total body water (18 liters). Extracellular fluid is divided into: Intravascular fluid (inside the blood vessels). It is the blood plasma (3 L) Interstitial fluid (between cells) in the tissue spaces (12 L) Transcellular fluid: that includes cerebrospinal fluid, intraocular fluid, intraplerual fluid, peritoneal fluid, pericardial fluid (200-500ml) Others: as fluid in bones and joints and dense connective tissue. The “internal environment” is the ECF in which the cells live. The ECF that fills the narrow spaces between cells of tissues is known as interstitial fluid. ECF contains substances necessary for the survival of the cells. Hence, it is termed as “internal environment”. ECF serves as a transition between the external environment and ICF inside the cells. Therefore, homeostasis is maintaining a constant ECF compartment. External environment ECF ICF (cells) Figure (4): Body fluid movement between compartments. Body Fluids composition There are important differences in the solute composition of the various compartments of body fluid, and these have major implication for normal cell metabolism and function. The main distinction between ICF and ECF is that the dominant cation in ECF is Na+ while in ICF is K+. Cl- and HCO3- make up most of the balancing anions in ECF, while in ICF the principal negative charges are carried by phosphates and proteins (Table 1). There is normally zero net flux of water across the cell membrane, i.e ECF and ICF are in the osmotic equilibrium. Page 5 of 9 Another important distinction between the composition of plasma and interstitial fluid is that the plasma, but not the interstitial fluid, contains a substantial concentration of proteins. The mechanism for maintain this protein difference is the presence of a permeability barrier at the capillary wall which largely prevents the movement of capillaries under normal circumstances. The significance of this protein concentration gradient is that it makes an important contribution to the balance of forces across the capillary wall (colloid osmotic pressure of plasma). Na+ is the dominant cation in ECF, together with its accompanying anions account for 95% of the solutes present in this fluid compartment. Thus Na+ is responsible for nearly all of osmotic activity in ECF. So factors which deplete Na+ (hyponatremia) will be associated with a low ECF volume (hypovolemia), whereas Na+ retention (hypernatremia) is associated with expansion of ECF volume (hypervolemia). Osmosis and tonicity: The movement of water across a membrane in response to a solute concentration gradient is called osmosis. In osmosis water moves to dilute the more concentrated solution. Once concentrations are equal, movement of water stops. The concentration of solutes in a fluid creates the osmotic pressure of the solution, which in turn determines the movement of water through membranes Osmotic pressure: it is the mechanical pressure needed in the concentrated solution to prevent water movement from the diluted side. The movement of water can be prevented by applying some pressure in the solution with high solute concentration. This pressure is exerted by the solutes dissolved by water. The osmotic pressure in solutions is determined by osmolality. Normally, the osmotic pressure of the cytosol is the same as the osmotic pressure of the interstitial fluid outside cells. Osmolality: is the concentration of osmotically active substances in the solution. It is the number of Page 6 of 9 particles (osmoles) per kilogram of water. Osmolality describes the total concentration of all particles that are free in a solution. Thus, glucose contributes one particle, whereas fully dissociated NaCl contributes two. Particles bound to macromolecules do not contribute at all to osmolality. Osmolarity: is another term to express the osmotic concentration. It is the number of particles (osmoles) per liter of solution. The osmolarity of plasma = 290 mosm/L. Comparing osmolarities of two solutions: You can compare the osmolarities of 2 solutions as long as the concentrations are expressed in the same units: If the 2 solutions contain the same number of solute particles per unit volume, we say that the solutions are isosmotic If solution (A) has higher osmolarity than solution (B), we say that solution (A) is hyperosmotic and solution (B) is hypoosmotic Tonicity: Tonicity is a physiological term used to describe a solution and how such solution affects the cell volume if the cell were placed in the solution and allowed to come to equilibrium. Tonicity compares a solution and a cell. (table 2, figure 5 ) Table 2: the effect of tonicity on cell volume Table 2: the effect of tonicity on cell volume Page 7 of 9 Figure 5: The effect of different tonicities on the cell size Membrane potential: A particular characteristic of all living cells is that there is always an electrical potential difference between the outer and inner surface of its surrounding membrane. It is caused by unequal distribution of electrically charged ions on both sides of the membrane with prevalence of cations at the outer surface and anions at the inner surface. Factors involved in production of membrane potential: 1)- Selective permeability across the cell membrane: The chief ions on the outer surface of the membrane are: Na+, Cl-, HCO3-, with small amounts of K+. The chief ions on the inner surface are: K+, and proteins , with little amounts of Na+, Cl- and HCO3-. Under resting conditions, the permeability of cell membrane to various ions is a matter of selection. It contains leak channels which allow certain ions to pass and prevents others. Every channel is specifically selective for the passage of one or more ions. The selective permeability of the membrane will be as follow: impermeable to proteins, which create negative charge at the inner surface of the membrane semipermeable to Na+ and K+ ions: ü sodium channels are specifically selective for passage of Na+ ions (leak channels). Na+ ions try to pass from EC to IC space due to concentration gradient ü K+ ions are smaller than Na+ ions , therefore they can pass easily through K+ leak channels. Membrane permeability is 50-100 times more than its permeability to Na+ ions.K+ ions try to pass from IC to EC space. Freely permeable to Cl- and HCO3, which diffuse from outside to inside of the membrane according to their concentration gradient. Migration of ions cannot continue because once the state of equilibrium is reached, the membrane becomes polarized i.e with positive charge at the outer surface of the membrane and negative charge at the inner surface. The +ve charge on the outer surface repels the outflux of K+ ions The –ve charge on the inner surface prevents further passage of Cl- and HCO3 Any amount of Na+ leaked to interior of the cell is out fluxed by Na+ pump 2)- Sodium- potassium pump: The cell maintains a relatively high K+ concentration and low Na+ concentration, not by making its membrane totally impermeable to these ions but by using the Na-K pump to actively extrude Na+ from the cell and to actively transport K+ into the cell. It is the most important active transport mechanism in the body. (Figure 6) Na+- K+ pump is responsible for the distribution of Na+ and K+ ions across the cell membrane and the development of –ve electrical potential inside the cell. Page 8 of 9 Figure(6) : Na+-K+ pump Page 9 of 9 5 SIGNALING ACROSS BIOMEMBRANES ILOs By the end of this lecture, students will be able to 1. Classify the different types of membranous receptors 2. Describe the mechanism of the signalling transduction pathways 3. Deduce importance of signalling across biomembranes in health and disease What is signaling? Signals evolving from cells are mostly released as chemicals/molecules in the extracellular fluid around them. They can be transported to distant targets in the body (endocrine signaling by hormones), to adjacent cells (paracrine signaling) or can even act on the same cell (autocrine signaling). The information conveyed by such signals are converted into several cellular responses within the target cells such as metabolism, gene expression, cell division and differentiation..etc. Stages of cell signaling Divided into 3 stages: Reception, Transduction, Response ( Figure 1) 1. Reception: Is the process where a cell recognizes the signaling molecule (a ligand) by a specific protein (a receptor) (on its surface or within its cytosol or nucleus). Binding of the ligand to its specific receptor has to occur in order to start signaling process. Once bound, the receptor initiates a process that amplifies the signal or integrates it with inputs from other receptors. Response ends by loss of binding of the ligand to its receptor. 2. Transduction: Is the cascade of events initiated by receptor-ligand binding which changes the conformation of the receptor causing its activation, hence permitting the flow of information to passes along sequential steps of molecular relays (Signal transduction). In such Signal Transduction Pathway, each relay molecule changes and activates the next group of molecules in the pathway causing amplification of the signal. Also along such pathway there is always upstream molecules and events that come earlier in the relay chain, followed by downstream ones that come later. N.B.Relay Molecules These are mostly proteins/enzymes but are sometimes non-protein or ions. They help transmit the signal from one relay molecule to the other until cellular response is reached. With some receptors, enzymes are the first effector proteins/relay [e.g adenyl cyclase, guanyl cyclase, phospholipase C] enzymes. Such enzymes are activated downstream of ligand binding to such receptors. In turn, they will either switch on/off other sets of proteins directly or achieve that through non-proteins [as cyclic AMP (cAMP), cyclic GMP (cGMP), phospholipid derivatives as inositol phosphate] or ions [as 1 Calcium]. These become considered as secondary messengers transmitting information further downstream. Relay proteins of transduction cascade are switched on/off by a phosphorylation/dephosphorylation process. Phosphorylation is achieved by addition of phosphate to serine or threonine residues by the action of “kinase family” of enzymes. While dephosphorylation is achieved by their removal by the action of “phosphatase family” of enzymes. Although in most cases, phosphorylation acts to switch on (activate) transmission in the face of dephosphorylation which turns it off, yet the reverse is often true where dephosphorylation can switch on transmission. 3. Response: Is the final step in a signal transduction pathway that activates a cellular response; contraction, secretion, motility, metabolic reaction, growth, transcription regulation and differentiation…etc) Figure 1. Steps of cell signaling TYPES OF RECEPTORS These are either present on Cell-Surface or Intracellular. I) Cell-Surface Receptors [Cell Membrane Receptors] Are membrane-anchored proteins that bind to ligands on the outside surface of the cell without need to cross the plasma membrane. Such ligands include large, hydrophilic molecules [as peptides and proteins] including growth factors, cytokines, some hormones or certain neurotransmitters Structurally: they have three different domains: an Extracellular Ligand-binding Domain for reception of ligand, a Hydrophobic Intra-membranous Domain and an Intra-cytosolic Domain for transmission of signals. Many types exist, but the commonest are: Ligand-gated ion channels, G protein-coupled receptors, and enzyme-linked receptors. A) Ligand-Gated Ion Channels ( Figure 2) Are ion channels that can open in response to the binding of a ligand to the receptor. 2 The hydrophilic channel within it, permits crossing of ions [Na+, K+, Ca 2+, Cl-] without having to touch the hydrophobic core of membrane phospholipid bilayer. The reverse is also true, as some channels are initially opened and ligand binding induces their closure. Their cellular response is rapid occurring on milliseconds. They are commonly found in excitable tissues as neurons, muscles and some secretory cells. Figure 2.Ligand gated ion channels Clinical Implication Gene mutation of chloride channels present on surface of epithelial cells in lungs, intestines, skin, and pancreas, results in Cystic Fibrosis. The disease presents as defective ion transport that leads to presence of very high concentrations of Na+ and Cl– in sweat and of highly viscous mucus that obstructs pancreatic and bile ducts and airways of the lungs. b) G Protein-Coupled Receptors (GPCRs) Are a large family of cell surface receptors that share a: Common structure [seven different protein segments crossing the membrane] present in an inactive state in the cell membrane when ligand is absent. Method of transmission of signal to inside the cell [by a protein called G protein ] Structurally; a G protein is heterotrimeric, i.e. made up of three subunits (α, β, γ). It is inactive “off” when attached to guanosine diphosphate (GDP) in absence of ligand. When ligand binds it becomes active (on) as guanosine triphosphate (GTP) becomes attached instead. G protein has an intrinsic GTPase activity that can break down (hydrolyze) GTP to GDP rendering it inactive as seen in Figure 3 Figure 3.G protein coupled receptors 3 There are many types of G protein that are categorized depending on the function of its α subunit.The most abundant of these are: Gs: Stimulates adenylate cyclase i.e the cyclic AMP pathway and Ca2+ Channels Gi: Inhibits adenylate cyclase Gq: Stimulates phospholipase C i.e. the phosphoinositide pathway. G0: Inhibits Ca2+ channels Clinical Implication Toxins produced by microorganisms as Bordetella pertussis (pertussis toxin) and Vibrio cholerae (cholera toxin) modify functions of certain G proteins producing diseased states. The Cholera toxin; is an enzyme that covalently modifies the α subunit of Gs to sustain it in a persistently activated state. This increases cAMP, in intestinal epithelium to induce large efflux of Cl and water into the gut producing the severe and potentially fatal diarrhea associated with - cholera. The Pertussis toxin; similarly, activates α subunit of Gi in airway epithelium. This inhibits exchange of GDP for GTP. As a consequence, adenylate cyclase is not inhibited and the increased cyclic AMP will lead to development of fluid imbalance and severe, life-threatening congestion characteristic to whooping cough in airway of patients infected with such organism. c) Enzyme-Linked Receptors Are receptors of many growth factors, cytokines and hormones and have a major role in regulation of cell growth, proliferation and differentiation. Structurally, beyond their extracellular ligand-binding domain, they posses a short hydrophobic transmembrane helix linked to an intracellular effector domain that possess an intrinsic enzyme activity or is associated directly with an intracellular enzyme. Enzyme linked receptors have many types; categorized according to nature of the linked effector enzyme. Examples include: 1-Receptor Tyrosine Kinase (RTK) The receptor is present at the cell surface in the form of a monomer (1 unit).When the ligand [e.g. insulin and most growth factors] binds the receptor, two receptors dimerize, which leads to activation of their intrinsic tyrosine kinase activity. This in turn induces auto-phosphorylation (on tyrosine units on the receptor) to further phosphorylate down-stream adapter proteins and other kinases.(Figure 4) RAS (small G-protein) is a downstream signal of RTK, that regulates normal cell proliferation and growth. Insulin receptor is a special RTK with special structure that regulates many cellular metabolic processes, mainly carbohydrate metabolism. (Refer to endocrine module) 4 2-Receptor Serine/Threonine Kinase When their ligand [few growth factors] binds, the sequence proceeds like that with RTK, but serine or threonine residues are autophosphorylated instead of tyrosine. 3-Tyrosine-Kinase Associated Receptors Their ligand [most cytokines] binds to dimerize two receptors. Thsis receptor doesn’t have an intrinsic kinase activity, but rather activates the associated proteins that have tyrosine kinase activity to phosphorylate downstream adapter proteins and other kinases 4-Receptor Guanylyl Cyclase Their ligand (Natriuretic Peptides] bind to activate guanyl cyclase with production of cGMP as a secondary messenger. Figure 4. Receptor tyrosine kinase Clinical Implication: The small RAS G-protein that is downstream of RTK can switch to become oncogenic under the influence of many cellular injurious insults. Consequently, it becomes constitutively active promoting uncontrolled cellular growth and cancerous transformation. Recent understanding of signaling via enzyme-linked receptors has permitted development of drugs in fields of regenerative medicine & tissue repair and in control of immune disorders and cancer. 5 6 15 Drugs Modulating Cell Signalling ILOs: By the end of this lecture, students will be able to 1. Classify how drugs can generally act while being outside the cell membrane. 2. Appraise how they can exactly modulate cell signalling mainly via targeting membrane receptors. 3. Analyse the plotted relation between drug concentration/dose and its response when constructing a graded dose response curve. 4. Distinguish the two concepts of agonism and antagonism and their subtypes in relation to changes in receptor signalling functions. 5. Correlate the modality of drug receptor interaction to their utility for achieving a therapeutic benefit. From what was so far studied, it was mentioned that drugs (like any other biochemical substance) are variably capable of modulating the: ▪ Actions of enzymes (extracellular or intracellular after diffusion or transport). (Refer to enzymes) ▪ Action on voltage-gated ion channels. (Refer to Transport) However, for most of the drugs in clinical use, the major target of drug action are the receptors whether being Cell-Surface Receptors (Cell-Membrane) (Refer to signalling across biomembranes) or intracellular (Refer to gene expression). Also, another target of drug action is on some membrane transporters (Refer to Victorian Transport) THE ACTION OF DRUGS IN MODULATING THE EFFECT OF RECEPTOR TRANSDUCTION PATHWAYS ACROSS THE CELL MEMBRANE, i.e., this is by modifying transduction of extracellular signals into intracellular responses. Such action falls into three categories: A. Drugs Modulating Ligand-Gated Ion Channels (Metabotropic Receptors) By binding instead of the ligand, drugs will alter flow of ions passing in the channels coupled to such receptors. Doing so, drugs can modulate diverse functions in excitable tissues as neurotransmission, neuronal or cardiac excitability, cardiac or muscle contractility. They can achieve this as in: a. Inhibition of influx of Na and efflux of K, in response to activation of Nicotinic Receptors at the neuromuscular junction to help in muscle relaxation with anaesthetics during operations. b. Stimulation of influx of chloride, in response to Gamma-amino Butyric Acid (GABA) receptor A to suppress neuronal function to help in treatment of convulsions or induction of sleep. A. Drugs Modulating G-Protein Coupled Receptors By binding instead of the ligand to these receptors, drugs will ignite or switch off the transduction cascade. Such cascade is initiated by the specific G-protein in question and its coupled 2nd messenger that links to their specific protein kinase, to affect many other down-stream effectors regulating diverse cellular functions. Since these receptors exist in most tissues, most of the Page 1 of 4 available drugs do work through their modulation to yield a therapeutic response triggered mainly by: a. Gs proteins linked to 2 adrenergic receptors, to activate adenylate cyclase (AC)→ increase cAMP → activate protein kinase A (PKA) to induce bronchodilation in bronchial asthma. b. Gi proteins linked to α2 adrenergic receptors, to inhibit AC → decrease cAMP → inhibit PKA, to reduce blood pressure in cases of hypertension c. Gq proteins, linked to α1 adrenergic receptors, to activate PLC → increase IP3 & DAG → activate PKC to increase blood pressure in hypotension induced by anaesthesia during surgery. B. Drugs Modulating Enzyme-Linked Receptors Most advancement in therapies targets these receptors that belong to growth factors, cytokines, some peptides, etc. acting mostly to control autoimmunity, inflammations, and cancer. When drugs act in link to these receptors they can: ▪ Bind to receptor instead of ligand to activate or inhibit down-stream receptor activation as the use of Exogenous Insulin to stimulate intrinsic tyrosine-kinase receptor to control Type I more than Type II Diabetes. ▪ Bind to the ligand itself to prevent its activation to its receptors as during the use of anti-TNFα monoclonal antibodies [mAb] to control Rheumatoid or Inflammatory Bowel disorders ▪ Binding to the down-stream signalling of the receptor directly as when immune modulators is used to treat some cancers. DRUG-RECEPTOR INTERACTIONS Upon interaction of a drug with its receptor the following is considered: Drug AFFINITY to show how well a drug recognises and binds to its receptor. Drug POTENCY to measure the required amount(quantity)(concentration)(dose) of drug necessary to produce an effect of a given intensity when reaching and binding with variable affinity to its receptor. Drug EFFICACY to measure the effectiveness (inherent ability) (intrinsic activity) of a drug to elicit a maximum achieved response, when given at the highest practical concentration. Accordingly, ⮚ When a drug binds to its specific receptor and the formed bound complex fails to elicit any desired response and meanwhile prevent the endogenous ligand from binding to such receptor, the drug is then called an ANTAGONIST; denoting that it only possesses affinity but no intrinsic activity. ⮚ When a drug (that possesses an intrinsic inherent ability) binds to its receptor and the formed bound complex elicits a desired response, the drug then is called an AGONIST; denoting that it possesses both affinity and efficacy. If the Relationship between the log Concentration (or the dose) of an Agonist and the evoked- response is plotted, an S-shaped curve develops and is called: Page 2 of 4 The Graded Dose-Response Curve: Figure 1 The curve, clears that: 🞂 The response increases with increasing the agonist dose, till it plateaus at a maximum concentration (Emax); we are speaking then of drug efficacy. Accordingly, the concentration giving half-maximal response; is then considered Effective Concentration 50 (EC50). 🞂 The potency of a drug is considered high if an effect of a given intensity is evoked by a small dose of a drug. If higher doses are needed, the potency of drug is low. 🞂 There are different types of agonistic and Figure 1: Dose-Response Curve antagonistic drugs that act on a receptor. A. Types of AGONISTS (Figure 2) If the response of that agonist ▪ Mimics the response of endogenous ligand, i.e has the same efficacy (inherent activity = 1) as natural ligand and is giving a maximum response, then this denotes that the drug is a FULL AGONIST. ▪ Is incapable of reaching the response of endogenous ligand; i.e has lower efficacy (inherent activity > zero & < 1) and is giving Full Agonist sub-maximal response, then this denotes that the drug is a PARTIAL AGONIST. ▪ Stabilizes a spontaneously activated receptor setting it back to Partial Agonist its inactive state, by utilizing its inherent activity (less than zero), to exert a pharmacological effect opposite to that triggered by the agonist; the drug is then called an INVERSE AGONIST. Inverse Agonist N.B.: On clinical use, no clear distinction can be made between inverse agonists and antagonists. Therefore, many of the inverse agonists are commonly classified as antagonists. B. Types of ANTAGONISTS If the response of that antagonist: ▪ Is blocking the agonist ability to bind to the specific binding site Figure 2: Types of agonists (active site) of its receptor. They have high affinity (competing with agonist for binding) - have zero inherent activity (maintaining receptor in inactive state) According to nature of binding, the drug is either: a. COMPETITIVE REVERSIBLE ANTAGONIST: its binding is non-permanent (reversible) and can be overcome by increasing the concentration of the agonist relative to it. b. COMPETITIVE IRREVERSIBLE ANTAGONIST: its binding is permanent because it forms a covalent bond with the active site. It cannot be overcome by increasing the concentration of the agonist. Its action only disappears after the body synthesis new receptors. Page 3 of 4 ▪ Is blocking the agonist ability to activate its receptor by binding at a different site (allosteric site). They do not interfere with binding of the agonist to its active site (have no affinity to it) and are not overcome by increasing concentration of the agonist. They have zero inherent activity (maintaining receptor in inactive state). They are called; NON-COMPETITIVE ANTAGONIST [ALLOSTERIC ANTAGONIST]. N.B. Other Modalities of Antagonism: 1. Physiological Antagonism: Achieving antagonistic effect by acting on a different receptor. As using adrenaline that induces vasoconstriction by action on α1-adrenergic receptors to antagonize the vasodilator action of histamine produced by its action on H1 receptors. 2. Chemical Antagonism: Achieving antagonistic effect by reacting chemically to form an inactive complex thus abolishing the action of the drug in question. As using Protamine sulphate that forms an inactive complex with heparin when there is need to stop haemorrhage induced by it. Page 4 of 4 7 Dynamics of Drug Actions ILOs By the end of this lecture, students will be able to: 1. Appraise the importance of efficacy versus potency in therapeutic selection. 2. Compare the quantitative distinction in response of different drugs when either acting on the same receptors or on different ones. 3. Explain the importance of potentiation & antagonism in fields of therapy. 4. Predict relative drug safety and drugs to be monitored upon analysing the quantal dose-frequency curves considering its effective and toxic responses. 5. Appraise implications of variation of drug response, in fields of therapy. GRADED DOSE-RESPONSE CURVE IS USED FOR: Quantitative Comparison of Effect of Different Drugs Acting on SAME RECEPTOR: E A B Comparing agonistic action of B, C, D, E, F to the full agonist “A” C as shown in Figure 1 which reveals: a. Drugs B, C, E are of same efficacy as “A” i.e., Full Agonists. D F b. It also reveals that potency of E> “A” while “A”>B>C in potency. c. Drugs D & F have less efficacy than “A” i.e., Partial Agonists. D>F in efficacy, while F> D in potency. Comparing the effect of addition of another drug to “A” a. If this drug causes a slope shift to the left “like the effect of E”: it is called “ POTENTIATION “. b. If this drug causes a slope shift to the right“like the effect of B” it is called “ANTAGONISM”. Comparing the effect of addition of an antagonist to “A” as shown in Figure 2 which reveals: a. If it causes a right parallel shift and appears to decrease potency of an agonist as in “B” and can be overcome by increasing concentration of the agonist, it is a Competitive Reversible Antagonist. b. If it causes a nonparallel shift to the right and appears to decrease efficacy of an agonist as in “C” and cannot be overcome by increasing concentration of the agonist, it is either a Competitive Irreversible Antagonist or a Non- Competitive Antagonist. A B Competitive – Potency Antagonism Efficacy Quantitative Comparison of Effect of Different Drugs C Irreversible - Acting on DIFFERENT RECEPTORS: Competitive Antagonism Non--Competitive Antagonism Fig 1: Comparing effects of Different Agonists. Fig 2: Dose-Response-Curve of Different Antagonists Comparing the action of drugs, A, B, C, D, on different receptors, shown in Figure 3 reveals: They can vary in efficacy; Drug B >A >D >C in efficacy. They could not be compared in potency as they do not act on same receptor. N.B. If one drug acting on a receptor increases the action of another drug acting on a different receptor; this is termed “SYNERGISM” or “ SUMMATION” , the new curve induced by both drugs will be more efficacious than that of the first drug alone. This is to differentiate from the forestated “POTENTIATION”, where the new curve induced by both drugs will be of more potency than that of the first drug alone. N.B. The Graded-Dose-Response-Curve gives information about the relation of drug concentration/dose in a particular tissue or whole body, but it does not reflect the relation between the drug dose and the proportion of population that therapeutically responded or that developed side effects. Alternatively, a QUANTAL DOSE-RESPONSE-CURVE (figure 4) has become of major clinical importance in justifying that. It is quantal because for any individual in the population the response is always all or none, i.e., - Therapeutically [a drug for sleep; induce sleep or not / a drug lowering cholesterol; dropped it to target level or not] - Adversely, e.g., hypoglycaemia, hepatic injury, hypertension, etc. or not]. QUANTAL DOSE-RESPONSE CURVE IS USED FOR: Predicting the relative DRUG SAFETY by: 1. Determining from this dose-response-frequency curve: Median-Effective-Dose, ED50: the drug dose that induces a specific therapeutic response in half the population. Median-Toxic -Dose, TD50: the drug dose that induces a special (adverse) toxic response in half the population. 2. Calculating the relative measure of drug safety, termed “THERAPUTIC INDEX” [TI] = TD50 / ED50 whereby if: TI is low drug is = not safe, as Digoxin. TI is high drug is = safe, as Penicillin (regarding the high doses). Determining Drugs that need THERAPEUTIC MONITORING: In clinical practice, determination of blood drug concentration is recommended for certain therapeutics. This is termed Therapeutic Drug Monitoring and is indicated when a drug has narrow therapeutic window, i.e., when the difference between Fig 3: Comparable Dose-Response of Different Fig. 4: Quantal Dose-Response-Curve the dose causing Drugs acting on different receptors. toxicity and therapeutic effect is very small, i.e., unsafe drugs as Warfarin. Drugs with wide therapeutic window, are safe and do not need monitoring as Ampicillin as shown in Figure 5. Fig. 5: Narrow versus Wide Therapeutic Window of drugs. VARIATION IN DRUG RESPONSE In certain instances, the response of drugs may become reduced, increased, or altered. If responsiveness to a drug becomes REDUCED gradually, in consequence to repeated administration, this is “TOLERANCE”. It indicates a need to increase the dose of a drug, to maintain the attained response. It could be caused by down regulation of receptors, or decrease in response effectiveness. “TACHYPHYLAXIS” is an acute rapidly developed tolerance, when doses of a drug are repeated in quick succession. N.B. “REFRACTORINESS” signifies the loss of therapeutic efficacy of a drug. “RESISTANCE” signifies the complete loss of effectiveness to antibiotics or anticancer…etc. If responsiveness to a drug becomes INCREASED: as the exaggeration in vasodilatation produced by Nitrates when it induces syncope; this is “HYPER-SUSCEPTIBILITY” (DRUG INTOLERANCE). If responsiveness to a drug becomes ALTERED: When an abnormal response to a therapeutic dose of a drug develops due to a genetic defect, this is “IDIOSYNCRASY” as with Sulphonamide developing haemolytic anaemia in patients with glucose-6- phosphate deficiency. When an immune response develops due to formation of antigen-antibody reaction, this is “HYPERSENSITIVITY REACTION” as with Penicillin developing skin reaction, bronchial asthma, or even anaphylaxis. When an adaptive state develops to repeated drug administration and upon its cessation, withdrawal manifestations appear, this is “DEPENDENCE” as with Habituation; developing to Nicotine in Cigarettes or Cannabis or as Physical Dependence “Addiction”; developing to Diazepam or Morphine. 8 Nucleus and phases of cell cycle ILOs By the end of this lecture, students will be able to 1. Correlate structure of different components to the nucleus to its function. 2. Differentiate between functional forms of the chromatin. 3. Interpret structural organization of the chromosome. 4. Discuss the nuclear and cellular changes during the phases of the cell cycle The Cell Nucleus: Introduction The nucleus contains a blueprint for all cell structures and activities, encoded in the DNA of the chromosomes. It also contains the molecular machinery to replicate its DNA and to synthesize and process the three types of RNA; ribosomal (rRNA), messenger (mRNA), and transfer (tRNA). (Are there DNA in the cell outside the nucleus?) The nucleus does not produce proteins; the numerous protein molecules needed for the activities of the nucleus are imported from the cytoplasm. Structure of the nucleus as seen by LM The nucleus frequently appears as a rounded or elongated structure, usually in the center of the cell (Figure.1A). Its main components are the nuclear envelope, chromatin, nucleolus, and nuclear matrix (Figure.1B). The size and morphological features of nuclei in a specific normal tissue tend to be uniform. In common hematoxylin and eosin-stained preparations; the nucleus, however, appears intensely stained dark blue or black. (Why?) Ultrastructure of the nucleus Three components are recognized: 1. Nuclear Envelope Electron microscopy shows that the nucleus is surrounded by two parallel membranes separated by a narrow space called the perinuclear cisterna. Together, the paired membranes and the intervening space make up the nuclear envelope. (Fig 1B) Polyribosomes are attached to the outer membrane, showing that the nuclear envelope is a in continuity with the endoplasmic reticulum. (Why?) At sites at which the inner and outer membranes of the nuclear envelope fuse, there are gaps, the nuclear pores (Figure 1B), that provide controlled pathways between the nucleus and the cytoplasm. Because the nuclear envelope is impermeable to ions and molecules of all sizes, the exchange of substances between the nucleus and the cytoplasm is made only through the nuclear pores. Ions and Page 1 of 5 molecules with a diameter up to 9 nm pass freely through the nuclear pore without consuming energy. But molecules and molecular complexes larger than 9 nm are transported by an active process, mediated by receptors, which uses energy from adenosine triphosphate (ATP). 2. Chromatin o Chromatin is composed mainly of coiled strands DNA bound to basic proteins (histones). o The basic structural unit of chromatin is the nucleosome (Figure 2), which consists of a core of four types of histones, wrapped around DNA base pairs. (What is the role of histones?) o Linker DNA; An additional DNA segment forms a link between adjacent nucleosomes, and another type of histone is bound to this DNA. This organization of chromatin has been referred to as "beads-on-a-string." Nonhistone proteins are also associated with chromatin, but their arrangement is less well understood. o Functional forms of Chromatin; in nondividing nuclei, is in fact the chromosomes in a different degree of uncoiling. According to the degree of chromosome condensation, two types of chromatin can be distinguished with both the light and electron microscopes (Figure 3). Heterochromatin (Gr. heteros, other, + chroma, color), which is electron dense, appears as coarse granules in the electron microscope and as basophilic clumps in the light microscope. It represents the inactive form of chromatin and acts as a reserve in less active cells. Euchromatin is the less coiled portion of the chromosomes, visible as a finely dispersed granular material in the electron microscope and as lightly stained basophilic areas in the light microscope. It represents the active form of chromatin and more abundant in active cells. The proportion of heterochromatin to euchromatin accounts for the light-to-dark appearance of nuclei 0in tissue sections as seen in light and electron microscopes. The intensity of nuclear staining of the chromatin is frequently used interpret the functional state of the nucleus. (How?) Figure 2 Nucleosome structure Page 2 of 5 Figure 3 Electron micrograph of a nucleus showing the heterochromatin (HC) and euchromatin (EC). Unlabeled arrows indicate the nucleolus-associated chromatin around the nucleolus (NU). Arrowheads indicate the perinuclear cisterna. Underneath the cisterna is a layer of heterochromatin, the main component of the so-called nuclear membrane seen under the light microscope. X 26,000. Careful study of the chromatin of mammalian cell nuclei reveals a heterochromatin mass that is frequently observed in female cells but not in male cells. This chromatin clump is the sex chromatin and is one of the two X chromosomes present in female cells. The X chromosome that constitutes the sex chromatin remains tightly coiled and visible, whereas the other X chromosome is uncoiled and not visible. Evidence suggests that the sex chromatin is genetically inactive. The male has one X chromosome and one Y chromosome as sex determinants; the X chromosome is uncoiled, and therefore no sex chromatin is visible. In human epithelial cells, sex chromatin appears as a small granule attached to the nuclear envelope. The cells lining the internal surface of the cheek are frequently used to study sex chromatin. Blood smears are also often used, in which case the sex chromatin appears as a drumstick-like appendage to the nuclei of the neutrophilic leukocytes. 3. Nucleolus o The nucleolus is a spherical structure (Figure 17-5) that is rich in rRNA and protein. It is usually basophilic when stained with hematoxylin and eosin. o Significance of the nucleolus; it contains DNA that codes for rRNA (type of RNA present inside ribosomes). The nucleolus is the site of synthesis of ribosomal subunits (to be explained later). Ribosomal proteins, synthesized in the cytoplasm, become associated with rRNAs in the nucleolus; ribosome subunits then migrate into the cytoplasm. Heterochromatin is often attached to the nucleolus (nucleolus-associated chromatin), but the functional significance of the association is not known. The rRNAs are synthesized and modified inside the nucleus. In the nucleolus they receive proteins and are organized into small and large ribosomal subunits, which migrate to the cytoplasm through the nuclear pores. (Figure 17-4) Page 3 of 5 4. Nucleoplasm: The protoplasm within the nucleus, consisting of a fluid portion, a proteinaceous matrix, and various ribonucleoproteins particles. Nuclear & cellular changes during cell cycle: Cell cycle The cell cycle is a series of events within the cell that prepare the cell for dividing into two daughter cells. Phases of the cell cycle: I- Interphase; long period of time during which the cell increases its size and content and replicates its genetic material. It includes three stages: a) G1 (gap) phase, synthesis of macromolecules essential for DNA duplication begins & cell growth. b) S (synthetic) phase, DNA is duplicated. c) G2 phase, the cell undergoes preparations for mitosis. II- Mitosis, a shorter period of time during which the cell divides its nucleus and cytoplasm, giving rise to two daughter cells. The cell cycle may be thought of as beginning at the conclusion of the telophase stage in mitosis, after which the cell enters interphase. (Figure 6) The interphase Gap 1 Daughter cells formed during mitosis enter the G1 phase. During this phase, the cells synthesize RNA, regulatory proteins essential to DNA replication, and enzymes necessary to carry out these synthetic activities. Thus, cell growth occurs restoring cell size to normal. The centrioles (a cell organelle involved in cell division) begin to duplicate themselves, a process that is completed by the G2 phase. S Phase During the S phase, the synthetic phase of the cell cycle, the genome is duplicated. The cell now contains twice the normal complement of its DNA. Autosomal cells contain the diploid amount of DNA before the synthetic (S) phase of the cell cycle that becomes doubled after S phase in preparation for cell division. Page 4 of 5 G2 Phase The gap 2 phase (G2 phase) is the period between the end of DNA synthesis and the beginning of mitosis. During the G2 phase, the RNA and proteins essential to cell division are synthesized, the energy for mitosis is stored. Duplication of centrioles and formation of the needed microtubules are completed. DNA replication is analyzed for possible errors, and any of these errors is corrected. Cells that become highly differentiated (What is meant by differentiation?) after the last mitotic event may stop to undergo mitosis either permanently (e.g., neurons, muscle cells) or temporarily (e.g., peripheral lymphocytes) and return to the cell cycle at a later time. Cells that have left the cell cycle are said to be in a resting stage, the G0 (outside) phase, or the stable phase. Figure 6. The cell cycle in actively dividing cells. Nondividing cells, such as neurons, leave the cycle to enter the G0 phase (resting stage). Other cells, such as lymphocytes, may return to the cell cycle. Page 5 of 5 9 NUCLEOTIDES & NUCLEIC ACIDS ILOs By the end of this lecture, students will be able to 1. Describe types & structure of nucleotides and nucleic acids 2. Explain function of nucleotides and nucleic acids 3. Interpret why the structure and organization of nucleic acids best fits their function What are nucleic acids? Nucleic acids are the most important of all biomolecules. They function to create and encode and then store information in the nucleus of every living cell of every life-form organism on earth. They represent the hard-disk of our body. The term nucleic acid is the overall name for Deoxyribonucleic acid (DNA) and Ribonucleic acid (RNA). They are composed of nucleotides, which are the monomers made of three components: 5-carbon sugar (pentose): If the sugar is ribose, the nucleic acid is termed RNA; if the sugar is deoxyribose, the nucleic acid is termed DNA. Phosphoric acid: Phosphoric acid is attached to OH group at 3′ carbon of deoxyribose sugar in one nucleotide and OH group at 5′ carbon of deoxyribose sugar in adjacent nucleotide. Nitrogenous bases: They are organic molecule with a nitrogen atom that has the chemical properties of a base. The main biological function of a nitrogenous base is to bond nucleic acids together. Nitrogenous bases are typically classified as the derivatives of two parent compounds: pyrimidines and purines (Figure1) Pyrimidines are formed of 1 cyclic ring. These include: Cytosine, thymine, uracil Purines are formed of 2 heterogeneous rings. These include: Adenine, Guanine Purines are present in both DNA and RNA. As for pyrimidines, the distribution is different. DNA contains cytosine and thymine while RNA contains cytosine and uracil. Thus, in summary, DNA and RNA are polymers of nucleotides. A nucleotide is composed of a nitrogenous base bound to a pentose sugar (both together form what is called NUCLEOSIDE), in addition to phosphate group. 1 Figure 1: Nitrogenous bases present in nucleic acids The number of phosphate groups can be either one group (nucleoside monophosphate), or 2 groups (nucleoside diphosphate), or 3 groups (nucleoside triphosphate) Formation of nucleotide polymers (Figure 2) Nucleotides are attached to each other through a 3’-5’ phosphodiester bond. This bond links carbon number 3 of the sugar and carbon number 5 in the sugar of the next nucleotide. Carbon number 5 links to this bond through sharing by its phosphate group. After polymerization, the formed chain will have a 3’ end (in which carbon number 3 in the sugar is free and not involved in any bond) and a 5’ end (in which carbon number 5 in the phosphate group of the sugar is free and not involved in any bond). Figure 2: Formation of Deoxyribonucleic acid (DNA) phosphodiester bond to link nucleotides to form nucleic acids To form DNA, the 2 strands of deoxyribonucleotides wind around each other in a clockwise direction, forming a structure called double helix. This structure is linear. In the double helix, the sugar and phosphates are located to the exterior, forming the backbone, while the nitrogenous bases are located to the interior for better protection from degradation. DNA is located within the nucleus of cell. It is associated with basic proteins and forms chromosomes. This is called nuclear DNA. 2 DNA is also located within mitochondrial matrix (mitochondrial DNA (mtDNA) and takes a circular double stranded form. Properties of DNA strands 1- Polarity Each DNA strand has polarity, with a 3′ End (3 prime) which is the terminal of DNA strand where C3 of deoxyribose sugar is not linked and is free, and 5′ End (5 prime) which is the end of DNA strand where C5 of deoxyribose sugar is not linked and is free. Therefore, the two DNA strands show polarity, where each strand shows runs either in 3′–5′ direction or 5′–3′ direction. 2- Anti-polarity (antiparallel strands) In DNA helix, two strands run in antiparallel direction; one strand runs from 3’ -5’ direction while the other runs from 5’-3’ direction. 3- Base Pairing Base pair signifies two nitrogenous bases held together through hydrogen bonding. Each pair of base consists of purine in one strand and pyrimidine in another strand. Figure 3: base pairing and antipolarity in DNA Particular purine always pairs with particular pyrimidine. This is called Complementary base pairing, where adenine pairs with thymine with double hydrogen bond and guanine pairs with cytosine with tripe hydrogen bond (A=T, G≡C) Eukaryotic DNA organization To fit the 2 meter long DNA in the nucleus, it is wrapped around the basic proteins called histones (5 types designated H1, H2A, H2B, H3, and H4) that, along with ions such as Mg 2+ , help neutralize the negatively charged DNA phosphate groups. The N-terminal ends of these histones can be acetylated, methylated, or phosphorylated. These reversible covalent modifications influence how tightly the histones bind to the DNA, thereby affecting the expression of specific genes. Histone modification is an example of epigenetics, or heritable changes in gene expression caused without alteration of the nucleotide sequence.(Refer to regulation of gene expression lecture) 3 Functions of DNA 1. DNA carries the genetic material of the cell and is responsible for transmission of this material to newly divided cells through making exact copies on itself. (Refer to DNA replication lecture) 2. DNA is responsible for protein synthesis through the process of gene expression. (Refer to transcription and translation lectures) Ribonucleic acid (RNA): RNA is present mainly in the cytoplasm of the cell and has three types: 1. Messenger RNA (mRNA): It constitutes 5% of all RNA. It is synthesized in the nucleus by DNA and then sent to the ribosomes in the cytoplasm. The mRNA carries a “coded” message from DNA in the nucleus, where it is synthesized, to the ribosomes in the cytoplasm, where it is going to direct the synthesis of a specific protein. The letters of the message are the nitrogenous bases, the sequence of which is complementary to that of the DNA from which it was copied. mRNA contains adenine, guanine, cytosine and URACIL. Each 3 successive bases in the mRNA are called a “codon” because they code for a specific amino acid. These codons are responsible for arranging the amino acids in proper order in the polypeptide chain to be synthesized. The process of synthesis of mRNA in the nucleus under the directions of DNA is called “transcription”. (Refer to transcription lecture) 2. Transfer RNA (tRNA): (Figure 5) It constitutes 15% of all RNA. It is present in the cytoplasm and may be also known as soluble RNA (sRNA).It is composed of 74 to 95 nucleotides. It has a hairpin structure and is stabilized by hydrogen bonds. tRNA molecules function as carriers for the amino acids and transfer them to the machinery site of protein synthesis in the cell ( Ribosome ). There is, at least, a specific tRNA for each amino acid. This means that there are, at least, 20 different tRNA molecules in every cell, each of which is specialized to carry one of the 20 different amino acids required for the process of protein synthesis. There is an amino acid binding site on the 3’ terminal. In addition there is an anticodon site for recognition of codons on mRNA. 3. Ribosomal RNA (rRNA): It constitutes 80% of total RNA. It is present in the ribosomes of the cytoplasm. 4 The ribosome is a cytoplasmic nucleoprotein structure which acts as the machinery for the synthesis of proteins. On the ribosomes, the mRNA and tRNA molecules interact to translate a specific protein. (Refer to the protein machinery lecture). Figure 5: Transfer RNA 5 10 DNA REPLICATION I : STEPS OF FORMATION ILOs By the end of this lecture, students will be able to 1. Interpret the importance of DNA replication rules in preserving human body 2. Identify the requirements of DNA replication 3. Discuss process of DNA replication 4. Correlate the benefits of altering specific steps of DNA replication by drugs to control certain disease states What is meant by replication? Replication is the process whereby DNA makes a copy on itself each time the cell divides, in order to supply daughter cells with a typical copy of DNA present in parent cells. In Eukaryotes, DNA replication occurs in the nucleus during S-phase of interphase (interval between two cell divisions).(Refer to cell cycle lecture) Rules of DNA replication in eukaryotes 1. DNA replication is semiconservative: Each DNA strand serves as a template for synthesis of a new strand producing two double-stranded DNA molecules, each with one new strand and one old strand. 2. Replication begins at multiple origins and usually proceeds bidirectionally. Having multiple origins of replication provides a mechanism for rapidly replicating the great length of eukaryotic DNA molecules. 3. Replication exhibits polarity: DNA synthesis proceeds in a 5` 3` direction and is semi- discontinuous (What do you think this means?) 4. Replication is very accurate: replication proceeds with an extraordinary degree of fidelity. Identification of origins of Replication A human chromosome is tens to hundreds of millions of base pairs long. Logistically, this means that replication would be far too slow if it proceeded from only a single origin. In reality, replication starts at a number of different sites, termed origins of replication, spaced 30,000 to 300,000 base pairs apart. From each origin, a replication fork (the point at which DNA strands unwind) is formed, and proceeds in either direction until they meet, or until they reach the end of their chromosome. The number of active origins of replication is variable. At times when rapid 1 duplication of DNA is required (e.g., cell division of the early embryo), more origins of replication may be active. To identify the points in DNA strands where replication should start, certain proteins called origin recognition complex (ORC)proteins, scan the DNA strands for regions rich in Adenine and thymine complementary bases (Can you deduce the reason?). Once found, the proteins bind to those regions, tagging them as origins of replication. (Figure 1) Steps of DNA replication Step 1: DNA Unwinding To be copied, the DNA double helix must first be unwound and the strands separated by breaking the hydrogen bonds between the nitrogenous bases. The process is catalyzed by enzymes called DNA helicases (Molecular scissors). (Figure 1) To prevent unwound DNA double strands from rewinding back, certain proteins called single-stranded DNA binding proteins, bind to unwound DNA strands. Local unwinding can cause overwinding, or supercoiling, of DNA downstream, the thing that can prevent DNA unwinding downstream. This supercoiling is prevented by proteins called DNA topoisomerases, which create breaks between nucleotides, allow the DNA to uncoil, and then re-anneal the broken nucleotides. ⮚ Topoisomerase I makes single-stranded breaks. ⮚ Topoisomerase II makes double-stranded breaks. Unwinding of DNA, causes the formation of a structure called replication fork As a rule, DNA replication should proceed in the 5’>>3’ direction on the newly synthesized strand, and it should proceed AWAY from the origin of replication. Figure 1: Initiation of replication 2 Step 2: RNA Primer Synthesis DNA polymerases, the enzymes responsible for replication, cannot initiate synthesis of a new strand by linking free nucleotides together. In addition to a template, DNA polymerase requires a primer, a short piece of RNA with a free 3' hydroxyl, which the DNA polymerase can elongate. RNA primers are synthesized by an enzyme called RNA primase, which is a component of a DNA polymerase- α protein complex. The RNA primase synthesizes a short RNA primer (about 8-12 bp long) and the DNA polymerase-δ and polymerase-ε extend this primer by adding deoxynucleotides. Step 3: DNA Polymerization (Figure 2) The new daughter DNA strand is synthes