Biological Membranes and Membrane Transport PDF
Document Details
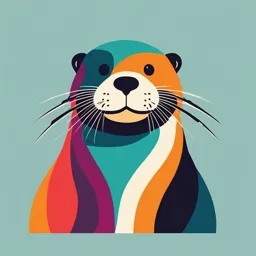
Uploaded by ComfortingAestheticism
University of Debrecen
2019
György Vámosi
Tags
Related
- Membrane Biochemistry Lecture 10 - Transport Across Biological Membranes I
- Transport Across Membrane PDF
- Hücrede Biyofiziksel Olaylar PDF
- RCSI FUNBIO.8 Biological Membranes Transport I (Microtransfer) PDF
- Lecture 2 2024 Functions of Body Functions and Biophysics PDF
- 02-Topic 2 Biological Membranes PDF
Summary
This document provides a detailed overview of biological membranes and membrane transport mechanisms, focusing on the structure, composition, and function of lipids, proteins, and carbohydrates within the membrane. It also delves into the dynamics of the membrane, including lipid rafts, and how different molecular motions like lateral diffusion are studied.
Full Transcript
Biological membranes and membrane transport The text under the slides was written by György Vámosi 2019 This instructional material was prepared for the biophysics lectures held by the Department of Biophysi...
Biological membranes and membrane transport The text under the slides was written by György Vámosi 2019 This instructional material was prepared for the biophysics lectures held by the Department of Biophysics and Cell Biology Faculty of Medicine University of Debrecen Hungary https://biophys.med.unideb.hu Dr. György Vámosi 2 Biological membranes are composed of two layers of lipids (bilayer) forming flat sheets that surround the cell and intracellular organelles: the cell nucleus and other subcellular structures. Biological membranes make it possible for the cell to maintain compartments having different molecular compositions and milieus with e.g. different pH values. This way the ions, proteins and other molecules can be kept where they are needed and can be prevented from diffusing into areas where they should not be. Various membrane-bounded compartments having specialized functions host distinct sets of enzymes, and other molecules and ions provide optimal conditions for the biochemical processes that take place in them. The plasma membrane (and organelle membranes) have a dual role. They not only isolate the cell (or organelle) from their environment as a diffusion barrier and as an electric insulator, but also connect them with the environment. They facilitate the controlled transport of materials into and out of the cell (or organelle), and transmit electric and chemical signals. 3 The cell membrane is composed of lipids (40-60%), proteins (30-50%) and carbohydrates: oligosaccharides (10%), which decorate lipids and proteins localized in the outer leaflet of the membrane (-> glycolipids, glycoproteins). 4 Lipids are amphipathic molecules having a hydrophilic head and a hydrophobic tail. In the plasma membrane lipids are organized into two leaflets opposing each other with the hydrophobic tails looking inward and the hydrophilic heads facing the aqueous phases of the extracellular space or the cytoplasm. Membrane proteins can be classified based on their mode of interaction with the membrane. Integral proteins immerse in the lipid bilayer, interacting with the hydrophobic core. They are insoluble in water. Transmembrane proteins span both leaflets, the membrane-spanning domain is usually α-helical. Peripheral or extrinsic proteins have no direct connection with the hydrophobic core, they connect to the membrane by noncovalent attachment to integral membrane proteins or by electrostatic interaction with the polar head groups of phospholipids. In glycolipids a mono- or oligosaccharide is connected to the head group at the extracellular side. To proteins called glycoproteins oligosaccharides are connected also on the ec. side. There are membrane proteins that covalently bind to membrane lipids, called glycosil- phosphatidyl-inositol (GPI) anchored proteins, named after the lipid that anchors them to the membrane. 5 Phospholipids are a class of lipids that are a major component of all cell membranes. They are amphipathic molecules containing hydrophilic (polar) and hydrophobic (non-polar) parts. The non-polar part is formed by two side chains of fatty acids containing 14-22 carbon atoms, connected by ester bonds to the glycerol. The third carbon atom of the glycerol backbone connects through a negatively charged phosphate group to another charged polar group such as choline, ethanolamine or serine resulting in phosphatidyl-serine, phospahtidyl-ethanolamine, etc. The polar heads have zero net electric charge for phospahtidyl-cholin and -ethanolamine, and carry unit negative charge for phosphatidyl-serine and -inositol. Sphingolipids contain a backbone of sphingoid bases, a set of aliphatic amino alcohols that include sphingosine. They play important roles in signal transduction and cell recognition. Sphingomyelin is a type of sphingolipid found in animal cell membranes, especially in the membranous myelin sheath that surrounds some nerve cell axons. The cell membrane is asymmetrical in composition. Phosphatidyl-ethanolamine, the negatively charged phosphatidyl-serine and inositol lipids involved in signaling (such as inositol 4,5- bisphosphate) are localized in the cytoplasmic leaflet almost exclusively. Phosphatidylcholine, sphingomyelin are mainly located in the outer leaflet. Glycolipids containing oligosaccharides composed of a varying number of sugar rings (galactose, glucose, N-acetyl-galactosamine) or negatively charged sialic acid, attached to the head groups, are localized in the outer leaflet. 6 In an aqueous environment lipids tend to aggregate to minimize their (Gibbs free) energy by avoiding contact of the apolar, hydrophobic parts with polar water molecules. Lipids line up in two leaflets opposing each other with the hydrophobic fatty acid tails looking inward and the hydrophilic heads facing the aqueous phases of the extracellular space or the cytoplasm. Such bilayers are ~5 nm thick. Membrane lipids usually contain one saturated fatty acid side chain and one unsaturated side chain with one or more double bonds. The shape of saturated fatty acids tails is linear, and the interaction between parallel-aligned chains is strong. Cis double bonds bend the axis of the aliphatic chain, which results in a higher separation distance and weakened interaction between aliphatic tails. In some lipids both aliphatic chains are saturated. Lipids can also form closed, spherically shaped bilayers called liposomes, and closed single lipid layers called micelles. Artificial liposomes are used to deliver drugs, medical imaging contrast substances, radiopharmacons, nucleic acids. The lipid composition of liposomes can be chosen freely according to their purpose, e.g., positively charged lipids are used for gene transfection (delivery of genes into target cells through the plasma membrane). Liposomes can be unilamellar (single bilayer) or multilamellar, and can vary in size. 7 Membrane proteins can be classified based on their different functions: transporters, anchors, receptors carrying out signal transduction and proteins having enzymatic activity. In this lecture we focus only on transporters. 8 Some of the lipids and proteins in the outer layer of the plasma membrane have sugars covalently attached to them. The great majority of these proteins have short chains of sugars, called oligosaccharides, linked to them; they are called glycoproteins. Other membrane proteins, the proteoglycans, contain one or more long polysaccharide chains. Carbohydrates attached to proteins and lipids form a sugar coating called the carbohydrate layer or glycocalyx. This layer of carbohydrate helps protect the cell surface from mechanical damage. As the oligosaccharides and polysaccharides adsorb water, they also give the cell a slimy surface, which helps motile cells such as white blood cells squeeze through narrow spaces and prevents blood cells from sticking to one another or to the walls of blood vessels. They have an important role in cell–cell recognition and adhesion as well. Proteins called lectins are specialized to bind to particular oligosaccharide side chains. The oligosaccharide side chains of glycoproteins and glycolipids, although short (typically fewer than 15 sugar units), are enormously diverse and can form branched structures. The carbohydrate layer on the surface of cells in a multicellular organism serves as a kind of distinctive clothing, like a uniform. It is characteristic of each cell type and is recognized by other cell types that interact with it. Specific oligosaccharides in the carbohydrate layer are involved, for example, in the recognition of an egg by a sperm. In bacterial infection, the carbohydrate on the surface of white blood cells called neutrophils is recognized by a lectin on the cells lining the blood vessels at the site of infection; this recognition causes the neutrophils to adhere to the blood vessel wall and then migrate from the bloodstream into the infected tissue. 9 If the organizing effect of the attracting forces between the hydrophobic side chains is not compensated by the intensity of thermal motion, the membrane is in an ordered, rigid, gel-like phase. In this state packing of lipids is tighter, diffusion is slower. If the temperature increases, the average kinetic energy per molecule increases leading to a phase transition of the membrane into the fluid phase. Above the phase transition temperature, called melting temperature (Tm), the lipid molecules become more mobile, lateral and rotational diffusion of lipids and the whipping motion of the tails is faster. Fatty acid chains become more disordered, and the interfacial area per molecule increases, the thickness of the bilayer decreases. The term used to characterize the ease at which molecules can diffuse in the membrane is membrane fluidity (that is the reciprocal of membrane viscosity). In the gel phase membrane fluidity is low and viscosity is high, in the fluid phase it is the opposite. 10 Tm increases linearly with added carbon atoms, and decreases with increasing number of cis double bonds. Tibor Farkas, a Hungarian biochemist discovered that fish regulate the Tm of their membranes by changing the ratio of saturated and unsaturated lipids of their membranes to adapt to seasonal temperature changes and maintain a constant membrane fluidity throughout the year. Cholesterol is a special lipid, having a dual effect on membrane fluidity. It has a rigid hydrophobic part consisting of a sterane frame with planar rings and a short hydrocarbon chain. The interaction of the planar rings with the fatty acid tail and, via H-bonds, with the polar head group of the neighboring lipid on one side is very strong; thus, cholesterol decreases membrane fluidity in the fluid phase. On the other hand, because of the rigidity of the planar rings, the hydrocarbon tail of cholesterol can only interact with the neighboring lipid on the other side; thus, cholesterol increases membrane fluidity in the gel phase. 11 An important experiment that provided evidence supporting fluid and dynamic biological membranes was performed by Frye and Edidin. They used Sendai virus to force human and mouse cells to fuse and form a heterokaryon (a multinucleate cell that contains genetically different nuclei). Using antibody staining, they were able to show that the mouse and human proteins remained segregated to separate halves of the heterokaryon a short time after cell fusion. However, the proteins eventually diffused and over time and became evenly distributed. Lowering the temperature slowed the rate of this diffusion by causing the membrane phospholipids to transit from a fluid to a gel phase. Singer and Nicholson rationalized the results of these experiments using their fluid mosaic model. The model is called mosaic because it proposes that the membrane is made up of many different parts, including proteins, carbohydrates, and lipids, which pave the surface of the cell much like the individual tiles of an ordinary mosaic. The model devised by Singer and Nicolson in 1972 describes the cell membrane as a two-dimensional liquid that allows a restricted lateral diffusion of membrane components. 12 The plasma membrane is not a homogenous “sea” of lipids and proteins, rather it contains microdomains that have distinct composition. Lipid rafts contain a higher amount of glycosphingolipids and cholesterol than the rest of the membrane, and certain membrane receptors are also enriched in them. It has been proposed that they are specialized membrane microdomains which compartmentalize cellular processes by serving as organizing centers for the assembly of signaling molecules and a platform for transmembrane signaling processes. Lipid rafts are more ordered and tightly packed than the surrounding bilayer, but float freely in the membrane bilayer. Although more common in the cell membrane, lipid rafts have also been reported in other parts of the cell, such as the Golgi apparatus and lysosomes. 13 Lipids and proteins in the membrane perform different types of motion: lateral and rotational diffusion, a whipping motion of the lipid tails, and flip-flop (exchange) between the membrane leaflets. Rotational diffusion of a fluorescent probe that is enriched in the membrane (e.g. the dye called diphenylhexatriene, DPH) gives information about the local viscosity of the membrane. A high fluorescence anisotropy of DPH refers to a low rotational mobility of the dye and a high membrane viscosity. (Fluorescence anisotropy or polarization measure by how much a dye can rotate due to thermal motion during the excited state of the dye. The less the rotation, the higher the anisotropy or polarization). The lateral diffusion of membrane components can be studied by several microscopy methods. Fluorescence Recovery After Photobleaching (FRAP) and Fluorescence Correlation Spectroscopy (FCS) report on the average mobility of an ensemble of molecules over a few micrometers or a few hundred nanometers distance, respectively, whereas Single Particle Tracking (SPT) can follow the trajectory (path) of single molecules. From such molecular mobility measurements the free/bound state or the monomeric/associated state of proteins and hindering of diffusion by e.g. the cytoskeleton or the boundaries of membrane domains can be revealed. 14 The lipid membrane acts a selective barrier for the diffusion of molecules and ions. Permeability decreases with increasing size and increasing polarity/electric charge of the particle. Small molecules such as gases (CO2, O2, NO) and small, uncharged polar molecules (e.g. urea and ethanol) can diffuse across the membrane because they do not have to interact very much with the hydrophobic tails of lipids due their small size. Some apolar, hydrophobic molecules (e.g. steroid hormones, the receptors of which are inside the cell) can also penetrate through the membrane. For large uncharged polar molecules (e.g. glucose), ions and charged polar molecules (e.g. amino acids, ATP, nucleotides) the membrane has a very low permeability. Such substances need the help of transport proteins to pass through the membrane. 15 Transport processes can be classified to passive mechanisms not requiring external energy and active mechanisms requiring it. Passive transport has two types: simple and facilitated diffusion. Simple diffusion takes place without the help of transport proteins. For neutral particles net diffusion occurs from the area of high concentration to the area of low concentration (or from high to low chemical potential). This is the transport mechanism for gases and hydrophobic substances. Facilitated diffusion takes place with the aid of transport proteins (channels or carriers), for neutral particles from high concentration to low concentration (i.e. from high to low chemical potential region), and for ions from high to low electrochemical potential. Active transport requires (Gibbs free) energy investment, and has two forms as well: primary and secondary active transport. In both cases at least one substance is transported from an area of lower to higher concentration (for ions, from lower to higher electrochemical potential). (A detailed description is given on a later slide.) 16 17 Membranes are permeable to uncharged, apolar molecules, which can cross the membrane by simple diffusion. If the extra- and intracellular concentrations of such a substance differ from each other, net transmembrane diffusion will occur. The matter flow density through the membrane can be described by Fick’s 1st law: 𝜟𝒄 𝒄𝒎𝟐 −𝒄𝒎𝟏 𝑱𝒎 = −𝑫𝒎 𝜟𝒙 = −𝑫𝒎. (Dm: diffusion coefficient of the substance in the lipid phase, d: 𝒅 thickness of the membrane). The concentrations of cm1 and cm2 in this equation (in the expression of the gradient) refer to the values measureable in the membrane near the membrane-water interface. However, only the extra- and intracellular concentrations in the aqueous extra- and intracellular phases, cw1 and cw2, are known per se. If a substance is dissolved in a hydrophobic (e.g. lipid membrane) and an aqueous medium (the extracellular space or the cytoplasm), which are in contact with each other but are immiscible, there will be an equilibrium between the concentrations of the substance present in the two different phases, and the ratio of the concentrations will be a constant value called partition coefficient (K), characteristic for the given substance and the solvents. The value of K expresses the relative solubility of the substance in the two media. For hydrophobic substances K>1, for hydrophilic ones K