Lecture 5 - Genetics PDF
Document Details
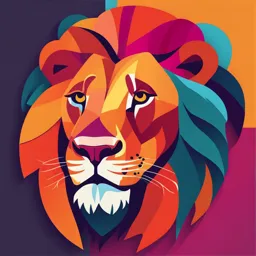
Uploaded by WonMaple
International Higher School of Medicine
Sven Vilain
Tags
Summary
This lecture covers gene regulation and switches in cells, differentiating between housekeeping and cell-type-specific genes. It also details transcriptional switches and their function, eukaryotic regulation, iPS cells, and the control of gene function by regulatory RNAs.
Full Transcript
International School of Medicine - Department of Medical Biology Medical Biology and Genetics Gene regulation and genetic switches Committee 2 - Lecture 5 Assist. Prof. Sven VILAIN [email protected] North Campus, C...
International School of Medicine - Department of Medical Biology Medical Biology and Genetics Gene regulation and genetic switches Committee 2 - Lecture 5 Assist. Prof. Sven VILAIN [email protected] North Campus, C Blok, Floor 1, Room 149 Objectives 1) Explain how gene expression can change inside cells, Determine the difference between housekeeping genes (or proteins) and cell type-specific genes (or proteins) 2) Describe the function and mechanism of transcriptional switches (regulators). Analyze logo diagrams. 3) Explain the mechanism of the bacterial switches in the Tryptophan operon and Lac operon. 4) Describe the difference between repressors and activators 5) Explain Eukaryotic transcription regulation via transcription complex (Mediator) 6) Describe how gene activators/repressors allow/prevent RNA polymerase access to open chromatin 7) Explain how and why different transcription regulators are used to control eukaryotic gene expression. 8) Describe how a single protein can activate several genes and how few transcription regulators and positive feedback loops regulators allows formation and maintenance of different cell types 9) Explain how iPS cells can be generated and used to study human diseases 10) Explain how mRNA can get degraded, untranslated regions 11) Explain how regulatory RNAs allows post-transcriptional control of gene function (microRNA, small interfering RNA and long non-coding RNA) The different cell types of a multicellular organism contain the same DNA Are genes selectively lost when a cell becomes more specialized? -> Can this genome of a differentiated cell develop into a complete organism? -> If it can develop to a complete new organism, no genes are lost -> Genes are necessary for normal development The different cell types of a multicellular organism contain the same DNA -> Take the genome from a differentiated cell -> Use this genome to develop a complete organism Example: Take the nucleus from a skin cell of an adult frog Injected it into a frog egg from which the nucleus has been removed The different cell types of a multicellular organism contain the same DNA -> Take the genome from a differentiated cell -> Use this genome to develop a complete organism Example: Take the nucleus from a skin cell of an adult frog Injected it into a frog egg from which the nucleus has been removed -> In some cases this egg will develop into normal tadpole -> If the chromosomes of the differentiated cell were altered irreversibly during development, they would not be able to accomplish this feat. The different cell types of a multicellular organism contain the same DNA -> Take the genome from a differentiated cell -> Use this genome to develop a complete organism Example: Take the nucleus from a skin cell of an adult frog Injected it into a frog egg from which the nucleus has been removed -> In some cases this egg will develop into normal tadpole -> If the chromosomes of the differentiated cell were altered irreversibly during development, they would not be able to accomplish this feat. Cells have the ability to change which genes they express without altering the nucleotide sequence of their DNA -> no genes are lost Cells have the ability to change which genes they express without altering the nucleotide sequence of their DNA This is also true for other multicellular organisms -> Take the genome from a differentiated cell -> Use this genome to develop a complete organism ⇒ All the cells of a multicellular organism contain the same genome. ⇒ A typical differentiated cell expresses only about half the genes in its total repertoire How can you analyze differences in gene expression between the different cell types? Different cell types produce different sets of proteins Analyze the protein content of a cell in liver, heart, brain, … Proteins common to all the cells of a multicellular organism -> called housekeeping proteins The structural proteins of chromosomes (RNA polymerases, DNA repair enzymes,…) Enzymes involved basic metabolic processes Proteins that form the cytoskeleton. Cell type specific proteins Specialized proteins responsible for the cell’s distinctive properties. (hemoglobin is made almost exclusively in developing red blood cells) Different cell types produce different sets of mRNAs Analyze the RNAs of a cell (including mRNAs that encode protein) Determine the nucleotide sequence and abundance of every RNA molecule a typical differentiated human cell expresses 5000–15,000 protein-coding genes from a total of about 21,000. mRNA common to all the cells of a multicellular organism -> Housekeeping genes Cell type specific mRNA What causes changes in gene expression in cells? A cell can change the expression of its genes in response to external signals External signal: Steroid hormone cortisol: released by the adrenal gland during periods of starvation, intense exercise or prolonged stress The fact that different cell types often respond in different ways to the same extracellular signal contributes to the specialization that gives each cell type its distinctive character. A cell can change the expression of its genes in response to external signals External signal: Steroid hormone cortisol: released by the adrenal gland during periods of starvation, intense exercise or prolonged stress Cellular response in: Liver: Increase production of several proteins is dramatically. Boost the production of glucose from amino acids and other small molecules. These proteins includes enzymes such as tyrosine aminotransferase, which helps convert tyrosine to glucose. Fat cells: the production of tyrosine aminotransferase is reduced Some other cell types do not respond to cortisol at all. present > protein production returns to resting level -mone not - The fact that different cell types often respond in different ways to the same extracellular signal contributes to the specialization that gives each cell type its distinctive character. At what level is the control of gene expression exercised? Gene expression can be regulated at various steps from DNA to RNA to protein A cell can control the proteins it contains by (1) Controlling when and how often a given gene is transcribed ↳ Transcriptional control ensures no unnecessary intermediates synthesised (2) Controlling how an RNA transcript is spliced or otherwise processed (3) Selecting which mRNAs are exported from the nucleus to the cytosol (4) Regulating how quickly certain mRNA molecules are degraded (5) Selecting which mRNAs are translated into protein by ribosomes (6) Regulating how rapidly specific proteins are destroyed (7) Activity of individual proteins can be further regulated in a variety of ways How do transcriptional switches work? Control of transcription happens when the transcription is initiated The promoters of bacterial and eukaryotic genes have a transcription initiation site Where RNA synthesis begins: Sequence of approximately 50 nucleotide pairs upstream from the initiation site. This region contains sites that are required for the RNA polymerase to recognize the promoter RNA polymerase does not bind to these sequences directly. Recognition sites for proteins that associate with the active polymerase -> sigma factor in bacteria -> the general transcription factors in eukaryotes Control of transcription happens when the transcription is initiated ON/OFF The promoters of bacterial and eukaryotic genes have a transcription initiation site, Where RNA synthesis begins Bacterial and eukaryotic genes have regulatory DNA sequences that are used to switch the gene on or off. Predominantly in bacteria Very short (10 nucleotide pairs) Act as simple regulatory switches that respond to a single signal Control of transcription happens when the ON/OFF transcription is initiated The promoters of bacterial and eukaryotic genes have a transcription initiation site, Where RNA synthesis begins Bacterial and eukaryotic genes have regulatory DNA sequences that are used to switch the gene on or off. Especially in Eukaryotic cells Regulatory DNA sequences are very long (more than 10,000 nucleotide pairs) Act as molecular command that dictates how often transcription of the gene is initiated. Regulatory DNA sequences do not work by themselves. Transcription regulators bind to regulatory DNA sequences GeneX transcription ON/OFF Regulatory sequences are recognized by proteins called transcription regulators. Binding of a transcription regulator to a regulatory DNA sequence controls transcription. The transcription regulators recognizes a specific DNA sequence The transcription regulators regulates a distinct set of genes. Humans contain several thousand transcription regulators, bacteria several hundreds Regulatory sequence Transcription regulators bind to regulatory DNA sequences GeneX transcription ON/OFF How do they function? Proteins that recognize a specific nucleotide sequence The surface of the protein fits tightly against the surface features of the DNA double helix in that region. This surface features varies depending on the regulatory sequence Regulatory sequence Mostly the protein inserts into the major groove of the DNA helix Transcription regulators bind to regulatory DNA sequences Mostly the protein inserts into the major groove of the DNA helix -) It makes a series molecular contacts with nucleotide pairs in the groove. -) Each individual contact is weak -> 10 to 20 contacts are highly specific and very strong Transcription regulators bind to regulatory DNA sequences Many transcription regulators bind to the DNA helix as dimers -) These dimers doubles the area of contact with the DNA -) Increases the strength and specificity of the protein–DNA interaction. Regulatory DNA sequences can be represented by ‘logo’ diagrams Represents the preferred nucleotide at each position of the sequence The height of each letter is proportional to the frequency with which this base is found at that position in the regulatory sequence. Regulatory DNA sequences can be represented by ‘logo’ diagrams The height of each letter is proportional to the frequency with which this base is found at that position in the regulatory sequence. In the first position: -> T is found more often than C In the second and third position: -> A is the only nucleotide found there Although regulatory sequences in the cell are double-stranded, a logo typically shows the sequence of only one DNA strand; the other strand is simply the complementary sequence. Transcriptional switches allow cells to respond to changes in their environment Bacterial switches Bacterial switches: Tryptophan operon Bacteria regulate the expression of many of their genes according to the food sources that are available in the environment. Tryptophan operon When tryptophan is abundant in the environment The amino acid is imported into the cell Enzymes are not needed Production of the enzymes is shut down Tryptophan operon When tryptophan is not abundant in the environment The amino acid is generated by the cell Enzymes are needed Enzymes are produced Tryptophan operon E. Coli has five genes code for enzymes that manufacture the amino acid tryptophan They are arranged in a cluster on the chromosome These coordinately transcribed clusters are called operons. Note: Operons are common in bacteria but rare in eukaryotes, where genes are transcribed and regulated individually Tryptophan operon E. Coli has five genes code for enzymes that manufacture the amino acid tryptophan They are arranged in a cluster on the chromosome Transcribed from a single promoter as one long mRNA molecule These coordinately transcribed clusters are called operons. Note: Operons are common in bacteria but rare in eukaryotes, where genes are transcribed and regulated individually Tryptophan operon Tryptophan concentrations are low Operon is transcribed -> mRNA the resulting mRNA is translated -> Proteins -> Expression of biosynthetic enzymes, which work in tandem to synthesize tryptophan. Tryptophan operon When tryptophan is abundant in the environment X the amino acid is imported into the cell Enzymes are not needed production of the enzymes is shut down Note: Operons are common in bacteria but rare in eukaryotes, where genes are transcribed and regulated individually How does the tryptophan operon works? Transcriptional switches allow cells to respond to changes in their environment There is a short DNA sequence called the operator within the operon’s promoter Which is recognized by a transcription regulator, the tryptophan repressor protein The tryptophan repressor protein is always present in the cell. How does the tryptophan operon works? The promoter contains two key blocks of DNA sequence information, the –35 and –10 regions, highlighted in yellow, which are recognized by RNA polymerase Essential Cell Biology, Fifth Edition Copyright © 2019 W. W. Norton & Company How does the tryptophan operon works? Low concentration of tryptophan inside a bacterium RNA polymerase (blue) binds to the promoter and transcribes the five genes of the tryptophan operon. Essential Cell Biology, Fifth Edition Copyright © 2019 W. W. Norton & Company How does the tryptophan operon works? High concentration of tryptophan, Repressor protein (dark green) becomes active and binds to the operator (light green) Binding of RNA polymerase to the promoter is blocked Essential Cell Biology, Fifth Edition Copyright © 2019 W. W. Norton & Company How does the tryptophan operon works? When concentration of intracellular tryptophan drops The repressor falls off the DNA, allowing the polymerase to transcribe the operon again. Essential Cell Biology, Fifth Edition Copyright © 2019 W. W. Norton & Company Repressors Turn Genes Off/Activators Turn Them On An activator protein binds to a regulatory sequence on the DNA -> Interacts with the RNA polymerase -> Helps to initiate transcription. Without the activator, the promoter fails to initiate transcription efficiently. Essential Cell Biology, Fifth Edition Copyright © 2019 W. W. Norton & Company Repressors Turn Genes Off/Activators Turn Them On In bacteria, the binding of the activator to DNA is often controlled by the interaction of a metabolite or other small molecule (red circle) with the activator protein. Essential Cell Biology, Fifth Edition Copyright © 2019 W. W. Norton & Company The Lac Operon When the cell has shortage of glucose but lactose is present, lactose can be used as energy source The Lac operon is controlled by two transcription regulators, the Lac repressor and CAP. NO LACTOSE: the Lac repressor binds to the Lac operator and shuts off expression of the operon. LACTOSE PRESENT -> Release of Repressor (Lactose increases the intracellular concentration of allolactose; allolactose binds to the Lac repressor, causing it to undergo a conformational change that releases its grip on the operator DNA.) The Lac operon is controlled by two transcription regulators, the Lac repressor and CAP. NO GLUCOSE: cyclic AMP (red circle) is produced by the cell, and CAP binds to DNA. For the operon to be transcribed, glucose must be absent (allowing the CAP activator to bind) and lactose must be present (releasing the Lac repressor). LacZ, the first gene of the operon, encodes the enzyme β-galactosidase, which breaks down lactose to galactose and glucose The Lac operon is controlled by two transcription regulators, the Lac repressor and CAP. For the operon to be transcribed: -) Glucose must be absent (allowing the CAP activator to bind) -) Lactose must be present (releasing the Lac repressor). LacZ, the first gene of the operon, encodes the enzyme β-galactosidase, which breaks down lactose to galactose and glucose The Lac operon is controlled by two transcription regulators, the Lac repressor and CAP. Summary: The CAP activator allow the cell to utilize alternative sources of carbon—including lactose. The Lac repressor shuts off the operon in the absence of lactose. Lac operon integrates two different signals for its activity Glucose must be absent and lactose must be present Eukaryotic transcription regulators control gene expression from a distance Eukaryotes use transcription regulators—both activators and repressors—to regulate the expression of their genes. The DNA sites to which eukaryotic gene activators bind are termed enhancers, -> their presence dramatically enhances the rate of transcription. Activator proteins can enhance transcription even when they are bound thousands of nucleotide pairs away from a gene’s promoter. How do enhancer sequences and the proteins bound to them function over such long distances? How do they communicate with the promoter? Eukaryotic transcription regulators control gene expression from a distance Many models for this “action at a distance” have been proposed The DNA between the enhancer and the promoter loops out to allow eukaryotic activator proteins to influence directly events that take place at the promoter. Eukaryotic transcription regulators control gene expression from a distance Many models for this “action at a distance” have been proposed The DNA between the enhancer and the promoter loops out to allow eukaryotic activator proteins to influence directly events that take place at the promoter. Often, additional proteins serve to link the distantly bound transcription regulators to these proteins at the promoter; The most important of these regulators is a large complex of proteins known as Mediator Eukaryotic transcription regulators control gene expression from a distance Many models for this “action at a distance” have been proposed One of the ways in which these proteins function: Help assemble the general transcription factors and RNA polymerase -> form a large transcription complex at the promoter. Eukaryotic repressor proteins do the opposite: they decrease transcription by preventing the assembly of the same protein complex. Initiation of transcription in eukaryotic cells must also take into account the packaging of DNA into chromosomes Eukaryotic DNA is packed into nucleosomes, which, in turn, are folded into higher-order structures. Nucleosomes can inhibit the initiation of transcription If they are positioned over a promoter They physically block the assembly of the general transcription factors or RNA polymerase on the promoter. How do transcription regulators, general transcription factors, and RNA polymerase gain access to such DNA? Chromatin structure can be altered by chromatin-remodeling complexes Enzymes can covalently modify the histone proteins that form the core of the nucleosome Gene activators can recruit chromatin-modifying proteins to promoters. -> Recruitment of histone acetyl- transferases Promotes the attachment of acetyl groups to lysines in histone tails. -> This modification alters chromatin structure allowing greater accessibility to the underlying DNA The acetyl groups themselves attract proteins that promote transcription, including some of the general transcription factors Chromatin structure can be altered by chromatin-remodeling complexes Gene activators can recruit chromatin-modifying proteins to promoters. Gene repressors can recruit chromatin-modifying proteins to promoters. Gene repressor proteins can modify chromatin in ways that reduce the efficiency of transcription initiation. Repressors can attract histone deacetylases enzymes that remove the acetyl groups from histone tails, Reverse the positive effects that acetylation has on histone deacetylases transcription initiation. The molecular mechanisms that create specialized cell types All cells must be able to switch genes on and off in response to signals in their environment. Cells of multicellular organisms have evolved this capacity to an extreme degree to form organized arrays of differentiated cell types. Once a cell in a multicellular organism decides to differentiate into a specific cell type The choice of fate is maintained through next cell divisions. Eukaryotic genes are controlled by combinations of transcription regulators Eukaryotic transcription regulators can control transcription initiation many base pairs away from the promoter Transcription regulators can function individually to turn a gene on or off. In eukaryotic cells transcription regulators work as part of a “committee” of regulatory proteins Goal: To express the gene in the right place, in the right cell type, in response to the right conditions, at the right time, and in the required amount. In eukaryotic cells transcription regulators work as part of a “committee” of regulatory proteins Eukaryotic genes Are controlled by combinations of transcription regulators In eukaryotes a typical gene is controlled by dozens of transcription regulators. These help assemble via Mediator complex Chromatin-remodeling complexes Histone-modifying enzymes RNA polymerase General transcription factors In many cases, both repressors and activators will be present in the same complex How the cell integrates the effects of all of these proteins to determine the final level of gene expression is only now beginning to be understood. The expression of different genes can be coordinated by a single protein In addition to being able to switch individual genes on and off All cells need to coordinate the expression of different genes. When a eukaryotic cell receives a signal to divide A number of unexpressed genes are turned on together This will set in motion the events that lead eventually to cell division The expression of different genes can be coordinated by a single protein All cells need to coordinate the expression of different genes. Bacteria coordinate the expression of a set of genes clustered in an operon controlled by single promoter. Such clustering is not seen in eukaryotic cells, where each gene is transcribed and regulated individually. So how do Eukaryotic cells coordinate gene expression? How can it rapidly and decisively switch whole groups of genes on or off? The expression of different genes can be coordinated by a single protein A single transcription regulator can be decisive in switching any particular gene on or off, It can complete the combination needed to activate or repress that gene. The expression of different genes can be coordinated by a single protein A single transcription regulator can be decisive in switching any particular gene on or off, It can complete the combination needed to activate or repress that gene. When different genes contain regulatory DNA sequences recognized by the same transcription regulator, They can all be switched ON or OFF together, as a coordinated unit. The expression of different genes can Be coordinated by a single protein cortisol receptor protein. In order to bind to regulatory sites in DNA, this transcription regulator must first form a complex with a molecule of cortisol. In response to cortisol, liver cells increase the expression of many genes. All these genes are regulated by the binding of the cortisol–receptor complex to a regulatory sequence in the DNA of each gene. The expression of different genes can Be coordinated by a single protein When the cortisol concentration decreases The expression of these genes drops to normal level. In this way, a single transcription regulator can coordinate the expression of many different genes. How can different cell types be generated? Combinatorial control can generate different cell types The ability to switch many different genes on or off using a limited number of transcription regulators allows eukaryotic cells to diversify into particular types of cells during embryonic development. Example: development of muscle cells: mammalian skeletal muscle cell -) Produces large numbers of muscle-specific proteins -> These genes are all expressed at the same time as muscle differentiate -) A small number of key transcription regulators, expressed in potential muscle cells are crucial for muscle-cell differentiation. -> coordinate muscle-specific gene expression -> are thus crucial for muscle-cell differentiation. Combinatorial control can generate different cell types Some transcription regulators can convert one MyoD specialized cell type to another. Fibroblast + transcription regulator MyoD (artificially introduced) -> muscle like cells. Fibroblasts ORIGIN: same class of embryonic cells as muscle cells, => They have the necessary transcription regulators required for the combinatorial control of the muscle-specific genes Addition of MyoD completes the unique combination required to direct the cells to become muscle. Combinatorial control can generate different cell types This type of reprogramming can produce even more dramatic effects. Artificially expression of a set of nerve-specific transcription regulators can convert liver cells into functional neurons. Combinatorial control can generate different cell types Lee and young, 2013 Combinations of a few transcription regulators can generate many cell types during development Simplified scheme (not real): A new transcriptional regulator is made after each division Repetition can generate 8 different cell types Each of these cell types would express many different genes Transcription regulators Allow Differentiated Cells to Maintain Their Identity Many differentiated cells will divide many times (proliferate) in the life of an individual. When they divide, they give only rise to cells like themselves. Cells have several ways of ensuring that their daughters “remember”what kind of cells they are. A master transcription regulator activates transcription of cell-type–specific genes Cells have several ways of ensuring that their daughters “remember”what kind of cells they are. Positive feedback loop Master transcription regulator activates transcription of its own gene. Each time a cell divides the regulator is distributed to both daughter cells, where it continues to stimulate the positive feedback loop. The continued stimulation ensures that the regulator will continue to be produced in subsequent cell generations. Specialized cell types can be experimentally reprogrammed to become pluripotent stem cells Pluripotent Embryonic stem (ES) cells -> can generate all the specialized cell types in the body Specialized cell types can be experimentally reprogrammed to become pluripotent stem cells Transcription regulators can persuade various differentiated cells To de-differentiate into pluripotent stem cells Much like the embryonic stem (ES) cells Specialized cell types can be experimentally reprogrammed to become pluripotent stem cells Transcription regulators can persuade various differentiated cells To de-differentiate into pluripotent stem cells Much like the embryonic stem (ES) cells A certain set of transcription regulators can reprogram cultured mouse fibroblasts To become induced pluripotent stem (iPS) cells Cells that look and behave like the pluripotent ES cells (derived from embryos) iPS cells from a variety of specialized cell types, including cells taken from humans. These human iPS cells can then be directed to generate a population of differentiated cells for use in the study or treatment of disease. Entire organs (made of different tissues) can be generated from a single transcription factor The formation of an entire organ can be triggered by a single transcription regulator Eye development in Drosophila. In this case, a single “master” transcription regulator called Ey can trigger the formation of not just a single cell type but a whole organ. In the laboratory, the Ey gene can be artificially expressed in fruit fly embryos in cells that would normally give rise to a leg. When these modified embryos develop into adult flies, some have an eye in the middle of a leg. The formation of an entire organ can be triggered by a single transcription regulator Ey is a transcription regulator Controls the expression of multiple genes Some of these genes are transcription regulators control the expression of other genes. illustration The formation of an entire organ can be triggered by a single transcription regulator Ey is a transcription regulator Controls the expression of multiple genes Some of these genes are transcription regulators control the expression of other genes. The action of a single transcription regulator illustration Produce a cascade of regulators -> formation of an organized group of many different types of cells. One can begin to imagine how, by repeated applications of this principle, a complex organism self-assembles, piece by piece. Ey is the homologue of the human transcription regulator PAX6 Conclusion Transcription regulators control gene expression by promoting or hindering the transcription of specific genes. The vast majority of genes in all organisms are regulated in this way. Post-transcriptional controls Gene expression can be regulated at various steps from DNA to RNA to protein A cell can control the proteins it contains by (1) Controlling when and how often a given gene is transcribed (2) Controlling how an RNA transcript is spliced or otherwise processed (3) Selecting which mRNAs are exported from the nucleus to the cytosol (4) Regulating how quickly certain mRNA molecules are degraded (5) Selecting which mRNAs are translated into protein by ribosomes (6) Regulating how rapidly specific proteins are destroyed (7) Activity of individual proteins can be further regulated in a variety of ways Transcriptional control can ensure that no unnecessary intermediates are synthesized. Each mRNA controls its own degradation and translation The more time an mRNA persists in the cell before it is degraded, the more protein it will produce. In bacteria, most mRNAs last only a few minutes before being destroyed. This instability allows a bacterium to adapt quickly to environmental changes. Eukaryotic mRNAs are more stable. Each mRNA controls its own degradation and translation mRNA’s lifetime is dictated by specific nucleotide sequences -> Sequences are mostly in untranslated regions (UTR) -> Untranslated regions are both upstream and downstream of the protein-coding sequence. UTR TRANSLATED UTR Each mRNA controls its own degradation and translation Bacterial mRNAs contain a short ribosome-binding sequence located a few nucleotide pairs upstream of the AUG codon where translation begins. This binding sequence forms base pairs with the RNA in the small ribosomal subunit, correctly positioning the initiating AUG codon within the ribosome. Each mRNA controls its own degradation and translation Blocking—or exposing—the ribosome-binding sequence allows the bacterium to inhibit—or promote—the translation of an mRNA. Regulatory RNAs control the expression of thousands of genes In addition to the mRNAs, which code for proteins, noncoding RNAs have various functions. Many noncoding RNAs regulate gene expression and are therefore referred to as regulatory RNAs. There are at least three major types of regulatory RNAs—microRNAs, small interfering RNAs, and long noncoding RNAs. microRNAs MicroRNAs direct the destruction of target mRNAs MicroRNAs (miRNAs) Small RNA molecules Control gene expression by base-pairing with specific mRNAs Reduce both their stability and their translation into protein. miRNA transcript undergoes a special type of processing to yield the mature, functional miRNA molecule, which is only about 22 nucleotides in length. MicroRNAs direct the destruction of target mRNAs MicroRNAs (miRNAs) Small RNA molecules Control gene expression by base-pairing with specific mRNAs Reduce both their stability and their translation into protein. This small but mature miRNA is packaged with specialized proteins to form an RNA-induced silencing complex (RISC), Which patrols the cytoplasm in search of mRNAs that are complementary to the bound miRNA molecule microRNAs direct the destruction of target mRNAs Destruction of the mRNA releases the RISC and allows it to seek out additional mRNA targets. A single miRNA can inhibit the transcription of a whole set of different mRNAs as long as all the mRNAs carry a common sequence, usually located in either their 5′ or 3′ untranslated regions. small interfering RNAs Small interfering RNAs Are produced from double- stranded, foreign RNAs to protect cells from infections RNA interference (RNAi) a powerful cell defense mechanism. -> Eliminate “foreign” RNA molecules Double-stranded RNAs produced by many viruses Small interfering RNAs Are produced from double- stranded, foreign RNAs to protect cells from infections First the double-stranded, foreign RNAs are cut into short fragments (approximately 22 nucleotide pairs in length) Protein called Dicer The resulting double-stranded RNA fragments, called small interfering RNAs (siRNAs). The RISC discards one strand of the siRNA duplex and uses the remaining single-stranded RNA to seek and destroy complementary foreign RNA molecules -> Eliminate “foreign” RNA molecules Application: RNAi method to silence endogenous genes Huntington’s disease symptoms could be improved via gene silencing Huntington’s disease Huntington’s disease is caused by alterations of Huntingtin protein Huntington’s disease An increase in numbers of CAG nucleotide segment causes the production of an abnormally long version of huntingtin protein which is toxic. -> Elongated protein is cut into smaller, toxic fragments -> Bind together and accumulate in neurons, -> Disruption of normal cellular functions. -> Neurons are dysfunctional and die in certain areas of the brain -> symptoms of Huntington’s disease. An increase in numbers of CAG nucleotide segment causes the production of an abnormally long version of huntingtin protein which is toxic. Question is how to prevent the disease? -> Silencing of HTT -> Find small DNA differences SNPs between healthy and toxic Huntingtin in the same person-> Silence only sick one. SNP allows silencing * of sick huntingtin An increase in numbers of CAG nucleotide segment causes the production of an abnormally long version of huntingtin protein which is toxic. Question is how to prevent the disease? -> Silencing of HTT -> Find small DNA differences SNPs between healthy and toxic Huntingtin in the same person-> Silence only sick one. SNP allows silencing * of sick huntingtin -> 85% of HD patients can be covered by 3SNPs. -> Targeting HD-associated SNP in HD mice gives 50% decrease in mutant huntingtin Methodology still requires some caveats and issues to overcome. Caveats -) HD mouse models may not directly translate to humans. -> Mouse brain may be better able to tolerate a decrease in normal huntingtin than the human brain. Hurdles -) Gene-silencing molecules have to be effectively delivered to the relevant parts of the body. -) The blood-brain barrier. -> Delivery of the molecules via viral based systems (immune response) -> Mesenchymal stem cells? -) High dosages of silencing molecules could have a toxic effect. -) Off-target gene silencing phenomenon -> gene silencing products accidentally bind to an undesired mRNA (mRNA coding for a protein that is not huntingtin). Conclusion -> These methods are very promising for treatments of diseases like huntington’s disease -> Still a lot of caveats and problems need to be overcome -> These strategies are still in Preclinical stages long noncoding RNAs Thousands of long noncoding RNAs may Also regulate mammalian gene Activity Thousands of long noncoding RNAs may also regulate mammalian gene activity RNA molecules that are more than 200 nucleotides in length. Xist RNA molecule, some 17,000 nucleotides long (a key player in X inactivation). Some long noncoding RNAs arise from protein-coding regions of the genome But are transcribed from the “wrong” DNA strand. Some of these antisense transcripts are known to bind to the mRNAs produced from that DNA segment, regulating their translation and stability—in some cases by producing siRNAs. RNA Double stranded RNA Conclusion Transcription Regulators Allow the regulation of gene expression Differences in gene expression -> Response of cells to various cues