Gene Regulation 2023 PDF
Document Details
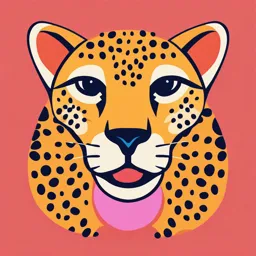
Uploaded by GleefulBowenite6939
UNAM
2023
Dr. J Sheehama
Tags
Summary
These lecture notes cover gene regulation at the University Of Namibia. They detail the processes of DNA replication, transcription, RNA translation, gene regulation in prokaryotes and eukaryotes, and the different levels of eukaryotic gene regulation.
Full Transcript
MBChB II/BPHARM II, Dent, OT & PHy 2023 DNA (GENETICS) AND INFORMATION TRANSFER DNA Replication, Transcription, RNA Translation and Gene- regulation S BIOCHEMISTRY II - MOLECULAR GENETIC S...
MBChB II/BPHARM II, Dent, OT & PHy 2023 DNA (GENETICS) AND INFORMATION TRANSFER DNA Replication, Transcription, RNA Translation and Gene- regulation S BIOCHEMISTRY II - MOLECULAR GENETIC Second Semester By: Dr. J Sheehama UNAM HG Campus@2023 Regulation of gene expression Second Semester LEARNING OUTCOMES At the end of this theme, you should be able to do the following: 1. Recognize the significance of post-translational modification in shaping the structure and function of polypeptides. 2. Analyze the impact of mutations on protein synthesis and identify human disorders resulting from such mutations. 3. Discuss the use of antibiotics as inhibitors of bacterial protein synthesis. 4. Recognize the mechanisms involved in regulating gene expression. Genes regulation and control Gene expression is regulated only at the transcriptional level in prokaryotic cells, whereas in eukaryotic cells, gene expression is regulated at the epigenetic, transcriptional, post-transcriptional, translational, and post- translational levels. Regulation of gene expression in eukaryotes and prokaryotes Introduction: Why regulate gene expression? ▪ It takes a lot of energy to make RNA and protein. ▪ Therefore some genes are active all the time because their products are in constant demand. ▪ Others are turned off most of the time and are only switched on when their products are needed. Differences between prokaryotes and eukaryotes gene expression : Prokaryote gene expression typically is regulated by an operon, the collection of controlling sites adjacent to polycistronic protein-coding sequences. Eukaryotic genes also are regulated in units of protein-coding sequences and adjacent controlling sites, but operons are not known to occur. Eukaryotic gene regulation is more complex because eukaryotes possess a nucleus. (transcription and translation are not coupled). Two “categories”of eukaryotic gene regulation exist: Short-term - genes are quickly turned on or off in response to the environment and demands of the cell. Long-term - genes for development and differentiation. Regulation of gene expression in prokaryotes Control of transcription Prokaryotic cells often respond initiation can be: to their environment by changes in gene expression. – positive control – increases transcription when activators Genes involved in the same metabolic pathway are organized bind DNA in operons. – negative control – reduces Some operons are induced when transcription when the metabolic pathway is repressors bind to DNA needed. regulatory regions called Some operons are repressed operators when the metabolic pathway is no longer needed. Lac operon The lac operon contains genes for the use of lactose as an energy source. Regulatory regions of the operon include the CAP binding site, promoter, and the operator. The coding region contains genes for 3 enzymes: – β-galactosidase, permease, and transacetylase The lac operon is negatively regulated by a repressor protein: – lac repressor binds to the operator to block transcription – in the presence of lactose, an inducer molecule binds to the repressor protein – repressor can no longer bind to operator – transcription proceeds In the presence of both glucose and lactose, bacterial cells prefer to use glucose. Glucose prevents induction of the lac operon. – binding of CAP – cAMP complex to the CAP binding site is required for induction of the lac operon – high glucose levels cause low cAMP levels – high glucose → low cAMP → no induction Trp operon The trp operon encodes genes for the biosynthesis of tryptophan. The operon is not expressed when the cell contains sufficient amounts of tryptophan. The operon is expressed when levels of tryptophan are low. The trp operon is negatively regulated by the trp repressor protein – trp repressor binds to the operator to block transcription – binding of repressor to the operator requires a corepressor which is tryptophan – low levels of tryptophan prevent the repressor from binding to the operator Eukaryote gene expression is regulated at six levels: 1. Transcription 2. RNA processing 3. mRNA transport 4. mRNA translation 5. mRNA degradation 6. Protein degradation 1. Transcription General transcription factors bind to the promoter region of the gene. RNA polymerase II then binds to the promoter to begin transcription at the start site (+1). Enhancers are DNA sequences to which specific transcription factors (activators) bind to increase the rate of transcription. Coactivators and mediators are also required for the function of transcription factors. – coactivators and mediators bind to transcription factors and bind to other parts of the transcription apparatus Chromosome structure, eukaryote chromosomes are packed with histones: Eukaryotes possess histones, and histones repress transcription because they interfere with proteins that bind to DNA. Transcriptionally active genes possess looser chromosome structures than inactive genes. Histones are acetylated and phosphorylated, altering their ability to bind to DNA. Enhancer binding proteins competitively block histones if they are added experimentally with histones and promoter-binding TFs. RNA polymerase and TFs “step-around” the histones/nucleosomes and transcription occurs. a) Chromatin remodeling Acetylation of histones enhances access to promoter region and facilitates transcription. DNA methylation and transcription control: Small percentages of newly synthesized DNAs (~3% in mammals) are chemically modified by methylation. Methylation occurs most often in symmetrical CG sequences. Transcriptionally active genes possess significantly lower levels of methylated DNA than inactive genes. A gene for methylation is essential for development in mice (turning off a gene also can be important). Methylation results in a human disease called fragile X syndrome; FMR-1 gene is silenced by methylation. 2. RNA processing control: RNA processing regulates mRNA production from precursor RNAs. Two independent regulatory mechanisms occur: Alternative polyadenylation = where the polyA tail is added Alternative splicing = which exons are spliced Alternative polyadenylation and splicing can occur together. Examples: Human calcitonin (CALC) gene in thyroid and neuronal cells. - Alternative polyadenylation and splicing of the human CACL gene in thyroid and neuronal cells. 3. mRNA transport control: Eukaryote mRNA transport is regulated. Some experiments show ~1/2 of primary transcripts never leave the nucleus and are degraded. Mature mRNAs exit through the nuclear pores. 4. mRNA translation control: Unfertilized eggs are an example, in which mRNAs (stored in the egg/no new mRNA synthesis) show increased translation after fertilization). Stored mRNAs are protected by proteins that inhibit translation. Poly(A) tails promote translation. Stored mRNAs usually have short poly(A) tails (15-90 As vs 100-300 As). Specific mRNAs are marked for deadenylation (“tail-chopping”) prior to storage by AU-rich sequences in 3’-UTR. Activation occurs when an enzyme recognizes AU-rich element and adds ~150 As to create a full length poly(A) tail. 5. mRNA degradation control: All RNAs in the cytoplasm are subject to degradation. tRNAs and rRNAs usually are very stable; mRNAs vary considerably (minutes to months). Stability may change in response to regulatory signals and is thought to be a major regulatory control point. Various sequences and processes affect mRNA half-life: AU-rich elements Secondary structure Deadenylation enzymes remove As from poly(A) tail 5’de-capping Internal cleavage of mRNA and fragment degradation 6. Post-translational control - protein degradation: Proteins can be short-lived (e.g., steroid receptors) or long-lived (e.g., lens proteins in your eyes). Protein degradation in eukaryotes requires a protein co-factor called ubiquitin. Ubiquitin binds to proteins and identifies them for degradation by proteolytic enzymes. Degradation of proteins marked with ubiquitin occurs at the proteasome. Amino acid at the N-terminus is correlated with protein stability and determines rate of ubiquitin binding. Arg, Lys, Phe, Leu, Trp 1/2 life ≤3 minutes Cys, Ala, Ser, Thr, Gly, Val, Pro, Met 1/2 life ≥ 20 hours Conclusion: ▪ Controlling gene expression is often accomplished by controlling transcription initiation. ▪ Regulatory proteins bind to DNA to either block or stimulate transcription, depending on how they interact with RNA polymerase. ▪ Prokaryotic organisms regulate gene expression in response to their environment. ▪ Eukaryotic cells regulate gene expression to maintain homeostasis in the organism.