Lecture 4 BIO3147 (2024).pdf
Document Details
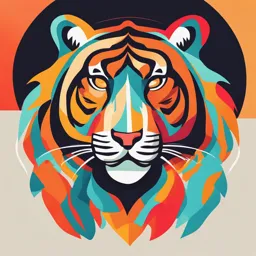
Uploaded by SafeMahoganyObsidian
2024
Tags
Full Transcript
Three Major Modes of Cell Specification 1. Autonomous Specification: Transcription factors localized in cytoplasm and are inherited by the cells containing that cytoplasm 2. Conditional Specification: Cells are influenced by their neighbors. Paracrine factors from one cell are received by another ce...
Three Major Modes of Cell Specification 1. Autonomous Specification: Transcription factors localized in cytoplasm and are inherited by the cells containing that cytoplasm 2. Conditional Specification: Cells are influenced by their neighbors. Paracrine factors from one cell are received by another cell and activate transcription factors in that cell 3. Syncytial Specification: Transcription factors form gradients within a large cell that contains many nuclei. The nuclei express genes depending on the ratios of these transcription factors. Figure 2.6 Conditional specification (A) What a cell becomes depends on its position in the embryo. Its fate is determined by interactions with neighboring cells. (B) If cells are removed from the embryo, the remaining cells can compensate for the missing part. Figure 2.7 Driesch’s demonstration of conditional specification (A) An intact 4-cell sea urchin embryo generates a normal pluteus larva. (B) When one removes the 4-cell embryo from its fertilization envelope and isolates each of the four cells, each cell can form a smaller, but normal, pluteus larva. (C) Note that the four larvae derived in this way are not identical, despite their ability to generate all the necessary cell types. (All larvae are drawn to the same scale.) Figure 2.7 Driesch’s demonstration of conditional specification Historical tangent: Driesch's goal was to explain development in terms of the laws of physics and mathematics. This strikingly modern concept of nuclear-cytoplasmic interaction and nuclear equivalence eventually caused Driesch to abandon science. Because the embryo could be subdivided into parts that were each capable of reforming the entire organism, he could no longer envision it as a physical machine. In other words, Driesch had come to believe that development could not be explained by physical forces. Harking back to Aristotle, he invoked a vital force, entelechy (“internal goal-directed force”), to explain how development proceeds. Essentially, he believed that the embryo was imbued with an internal psyche and wisdom to accomplish its goals despite the obstacles embryologists placed in its path. Unable to explain his results in terms of the physics of his day, Driesch renounced the study of developmental physiology and became a philosophy professor Figure 2.9 The syncytial blastoderm in Drosophila melanogaster (A) Schematic of the progression of blastoderm cellularization in Drosophila (nuclei are red). (B) Still frames from a timelapse movie of a developing Drosophila embryo with nuclei that are premitotic (blue) and actively dividing in mitosis (purple). Cellularization only happens at cycle 14! Nuclei are dynamically ordered within the syncytium of the early embryo, holding their positions using cytoskeletal elements. Figure 2.10 Positioning of nuclei during the interphase stage of nuclear cycle 13 of the Drosophila melanogaster syncytium Syncytium: The nuclei undergo S-phase (DNA replication) and sister chromatids get pulled apart and re-assembled into nuclei containing full sets of homologous chromosomes, but cytokinesis does not occur. Thus, the nuclei multiply in a common cytoplasmic space. Nuclei are dynamically ordered within the syncytium of the early embryo, holding their positions using the cytoskeletal elements associated with them (‘asters’). Fly Embryogenesis LA Royer Figure 2.11 Morphogen gradients during syncytial specification in Drosophila melanogaster The amounts of each morphogen differentially activate transcription of the various nuclear genes that specify the segment identities of the larval and the adult fly. Anterior-posterior specification originates from morphogen gradients in the egg cytoplasm, specifically of the transcription factors Bicoid and Caudal. Morphogen gradients during syncytial specification in Drosophila melanogaster Figure 2.12 The developmental landscape of cell fate maturation (Part 1) Figure 2.12 The developmental landscape of cell fate maturation (Part 2) (B) T-distributed stochastic neighbor embedding (tSNE) plots for each developmental time point, with cells colored according to their expressed genes of known germ layer identity. Figure 2.13 Developmental ‘trees’ (2018 Science Breakthrough of the year) (C) Visualization of a full gene expression landscape with representative cell states over the course of the first 24 hours of zebrafish embryonic development. The earliest time points are at the image’s center, with more differentiated cells emanating outward. Figure 2.14 The developmental landscape of cell fate maturation (Part 5) Figure 3.1 Key steps in the expression of a protein-coding gene (the “central dogma” of molecular biology) Figure 3.3 Cloning a mammal using nuclei from adult somatic cells (‘Power of the Genome’) Cloning a mammal using nuclei from adult somatic cells. (A) Procedure used for cloning sheep. (B) Dolly, the adult sheep on the left, was derived by fusing a mammary gland cell nucleus with an enucleated oocyte, which was then implanted in a surrogate mother (of a different breed of sheep) that gave birth to Dolly. Dolly later gave birth to a lamb (Bonnie, at right) by normal reproduction. Figure 3.2 Gene expression In situ hybridization is an immunohistochemical method to visualize gene expression in space through time (spatiotemporal). (B) mRNA expression seen here in blue, using in situ hybridization with an antisense DIG-labeled RNA probe of the odd-skipped gene in a stage-5 and a stage-9 Drosophila embryo. (C) mRNA expression of the odd-skipped related 1 gene in an 11.5-days-postconception mouse embryo (blue; important for kidney, heart, and palate development). Figure 3.5 Nucleosome and chromatin structure Histones H2A and H2B are yellow and red, respectively; H3 is purple; and H4 is green, DNA helix (gray) winds around the protein core. Histone H1 can draw nucleosomes together into compact forms. The histone “tails” that extend from the core are the sites of acetylation and methylation, which may disrupt or stabilize, respectively, the formation of nucleosome assemblages. Figure 3.6 Steps in the production of β-globin and hemoglobin Transcription of the βglobin gene creates a pre-mRNA containing exons and introns as well as the cap, tail, and 3′ and 5′ untranslated regions. Processing the premRNA into messenger RNA removes the introns. Translation on ribosomes uses the mRNA to produce a protein. The β-globin protein is inactive until it is modified and complexed with αglobin and heme to form active hemoglobin. Figure 3.7 The bridge between enhancer and promoter can be made by transcription factors The example shown here is the mouse βglobin gene. (A) Transcription factors assemble on the enhancer, but the promoter is not used until the GATA1 transcription factor binds to the promoter. (B) GATA1 can recruit several other factors, including Ldb1, which forms a link uniting the enhancer-bound factors to the promoterbound factors. Figure 3.8 Enhancer region modularity Figure 3.8 Enhancer region modularity (Part 1) (i) The top diagram shows the exons, introns, promoter, and enhancers of a hypothetical gene A, but does not show how the two enhancers are involved in the expression of the gene (see ii and iii). In situ hybridization (left) shows that gene A is expressed in limb and brain cells. Figure 3.8 Enhancer region modularity (Part 2) (ii) In developing brain cells, brain specific transcription factors bind to the brain enhancer, causing it to bind to the Mediator, stabilize RNA polymerase II at the promoter, and modify the nucleosomes in the region of the promoter. The gene is transcribed in the brain cells only; the limb enhancer does not function. Figure 3.8 Enhancer region modularity (Part 3) (iii) An analogous process allows for transcription of the same gene in the cells of the limbs. The gene is not transcribed in any cell type whose transcription factors the enhancers cannot bind. Figure 3.8 Enhancer region modularity (Part 4) (B) The Pax6 protein is critical in the development of several widely different tissues. Enhancers direct Pax6 gene expression (yellow exons 1–7) differentially in the pancreas, the lens and cornea of the eye, the retina, and the neural tube. (C) A portion of the DNA sequence of the pancreas-specific enhancer element. This sequence has binding sites for the Pbx1 and Meis transcription factors; both must be present to activate Pax6 in the pancreas.