Lecture 11 - Hearing and Vestibular Systems PDF
Document Details
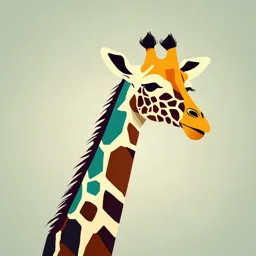
Uploaded by PortableMoscovium
McGill University
Jonathan Britt
Tags
Summary
These lecture notes cover the hearing and vestibular systems, including the anatomy of the ear, function of hair cells, and perception of pitch and loudness. Information on how the brain interprets sounds is also included. The notes also explore different types of sounds and how they're processed by the human ear.
Full Transcript
Introduction to Behavioral Neuroscience PSYC 211 Lecture 11 of 24 – Hearing & Vestibular Systems Textbook chapter 7-1 (pp. 173-188) Professor Jonathan Britt Questions? Concerns? Please write to [email protected] SOUND WAVES Wh...
Introduction to Behavioral Neuroscience PSYC 211 Lecture 11 of 24 – Hearing & Vestibular Systems Textbook chapter 7-1 (pp. 173-188) Professor Jonathan Britt Questions? Concerns? Please write to [email protected] SOUND WAVES When an object vibrates, it causes molecules in the surrounding air to alternately condense and rarefy (pull apart). These fluctuations in air pressure give rise to a sound wave that travels away from the object at approximately 700 miles per hour. AUDITION (HEARING) The human ear can transduce fluctuations in air pressure when the length of the sound wave is between 0.017 and 17 meters long. These sound waves are generated when physical objects vibrate between 20 and 20,000 times per second. SOUND Sound has three physical dimensions: Loudness corresponds to the amplitude or intensity of the molecular vibrations, the relative difference in the density of air molecules between compressed and rarified air. This dimension determines how far the sound wave will travel. Pitch (tone) corresponds to the frequency of the molecular vibrations (or the distance between neighboring peaks of compressed air). It is measured in hertz (Hz, cycles per second). Every frequency has a corresponding wavelength. Timbre corresponds to the complexity of the sound wave. Our brains learn to Non-repeating variations in air pressure are recognize the timbre of sound waves to perceived as noise, not as identifiable notes. identify the source of the sound (e.g., which instrument is playing the note middle C). ANATOMY OF THE EAR 3. The middle ear is comprised of three ossicles (small bones): the malleus, incus and stapes. Vibrations of the tympanic membrane cause the ossicles to vibrate, which in turn cause the membrane behind the oval window to vibrate. Vibrations of the oval window are transmitted to the fluid-filled cochlea (the inner ear), which is a long coiled tube-like structure that contains sensory neurons. 1. Sound is funneled 2. At the end of ear canal (still outer ear), sounds cause through the pinna the tympanic membrane (the eardrum) to vibrate. These (the outer ear) vibrations are transferred to the middle ear. ANATOMY OF THE EAR Another view of the 3 bones in the middle ear BASILAR MEMBRANE High pitched notes are detected where the basilar membrane is thick and narrow (closest to the oval window). Low notes are detected where the basilar membrane is thin and wide. The basilar membrane is kind of like a xylophone backwards, where the longest wood bars correspond to the low notes. SOUND PERCEPTION ANIMATED CROSS SECTION THROUGH COCHLEA The cells that transduce sound are called hair cells because of their physical appearance. Their hair-like extensions are called cilia. The cochlea is divided into three longtudinal divisions: scala vestibuli, scala media, and scala tympani. Outer hair cells have cilia that are physically attached to the rigid tectorial membrane. The cilia of The receptive organ is inner hair cells are not attached to the organ of Corti. It anything. They sway back and forth consists of the basilar with the movement of the solution. membrane on the bottom, the tectorial Sound waves cause the basilar membrane to move relative to membrane on the top, the tectorial membrane, which causes hair cell cilia to stretch and auditory hair cells and bend. The movement of the cilia pulls open ion channels, in the middle. which changes the membrane potential of hair cells. INNER & OUTER HAIR CELLS Although there are 3 times more outer hair cells than inner hair cells, only inner hair cells transmit auditory information to the brain. Outer hair cells act like muscles to adjust the sensitivity of the tectorial membrane to vibrations. By regulating the flexibility of the tectorial membrane, outer hair cells influence the sensitivity of inner hair cells to specific frequencies of sound (i.e., different notes). People who do not have working inner hair cells are completely deaf. People who do not have functional outer hair cells can hear, but not very well. HAIR CELL CILIA The cilia of hair cells are connected to each other by tip links – elastic filaments that attach the tip of one cilium to the side of adjacent cilium. The point of attachment of a tip link to a cilium is called an insertional plaque. Each insertional plaque has a single ion channel in it that opens and closes according to the amount of stretch exerted by the tip link. LOUD NOISE Loud noises can easily break the tip links that interconnect each cilia. And hair cells cannot transmit auditory information without tip links. Fortunately, tip links usually grow back within a few hours. Tip link breakage generally corresponds to temporary hearing loss (such as after a loud bang or loud concert). Tip link breakage is probably a protective measure, because too much glutamate release onto the cochlear nerve causes permanent cell death (excitotoxicity). 20% of 20-year-olds seem to have some noise-induced hearing loss, presumably due to cochlear nerve damage. PERCEPTION OF PITCH The major principle of auditory coding is that different frequencies of sound produce maximal stimulation of hair cells at different points on the basilar membrane. This approach to encoding sensory information is known as place coding. The position of the most active hair cell in the cochlea indicates the fundamental frequency (the pitch) of the sound wave. Moderate to high frequencies are entirely encoded by place coding. Human speech is in this frequency range. Very low frequencies are largely encoded by rate coding (also known as temporal coding). PERCEPTION OF PITCH Rate coding is used to identify Place Coding: Because of how the pitch of low frequency sounds the cochlea and basilar membrane are constructed, acoustic stimuli of different frequencies cause different amounts of movement along the basilar membrane. Higher frequency sounds cause bending of the basilar membrane closest to the stapes, resulting in more hair cell activity in that area. Rate coding: Low frequency sounds are processed using a rate coding system: the pattern of neurotransmitter release from the hair cells deepest in the cochlea (furthest from the stapes) determines the perception of low frequency sounds. INNER VS OUTER HAIR CELLS These three V-shaped tuning curves indicate the sensitivity of three individual inner hair cells, as - loudness shown by their response threshold to pure tones of varying frequency. Low points of three solid curves indicate that these inner hair cells will respond to faint sound only if it is of a specific frequency. If the sound is louder, cells will respond to frequencies above and below their preferred frequencies. Lesions targeted to outer hair cells disrupt the responsiveness of inner hair cells to specific sounds. AUDITION Pitch Perception Moderate to high frequencies are encoded by place coding. Low frequencies are partly encoded by rate coding. Loudness Loudness corresponds to the total number of hair cells that are active and their overall activity levels. Timber Timbre is perceived by assessing the precise mixture of hair cells that are active throughout the entire cochlea. (More on next couple slides.) OVERTONES The fundamental frequency of a sound wave is the lowest frequency in the wave. Natural sounds are comprised of a fundamental frequency and a collection of overtones, which are generally integer multiples of the fundamental frequency. Permitted standing waves Forbidden standing waves Because strings (and membranes) are clamped on each end, oscillations tend to only occur at integer multiples of the fundamental frequency. TIMBRE Fundamental frequency – The lowest and most intense frequency of a complex sound. This frequency is what is most often perceived as sound's basic pitch. Overtone – Sound wave frequencies that occurs at integer multiples of the fundamental frequency The timbre of sound refers to the specific mixture of frequencies (fundamental frequency plus overtones) that different instruments emit when the same note is played. It is the complexity of the sound wave. We analyze the timbre of a sound and how the timbre changes over time to identify which instrument made the sound. COCHLEAR IMPLANTS Cochlear implants for the hearing-impaired primarily take advantage of the place coding system of the cochlea. They elicit the perception of different notes by stimulating different places along the cochlea (with 20 to 24 evenly spaced electrodes). Loudness is controlled by the frequency of stimulation. Understanding human speech is often best when positions corresponding to 250 Hz to 6500 Hz are stimulated, even though the fundamental frequency of human speech is 100-250 Hz. The abundance of overtones provides the impression of the fundamental tone. IDENTIFYING THE DIRECTION OF A SOUND Interaural cues are differences in sound perception between the two ears. One of the main ways we localize sounds is by analyzing the timing difference between the 2 ears (which ear heard the sound first). Additionally, for high frequency sounds (above 800 Hz) we use interaural loudness differences to help identify the location of a sound (which ear heard it louder). This approach is possible because the loudness of a high-pitched (high frequency) sound is significantly dampened by the head. To help localize low frequency sounds (below 800 Hz, which corresponds to wavelengths that are longer than the width of the head), the brain analyzes the phase difference between the two ears. Our auditory system localizes low pitched sounds (under 800 Hz) by analyzing phase differences between the 2 ears. This approach only works for sound waves that are longer than the width of the head. IDENTIFYING THE HEIGHT OF A SOUND The approaches described on the previous slide cannot be used to identify whether a sound is coming directly in front of you, behind you, or above you. To discriminate among these options, we analyze the timbre of the sound wave. The shape of our outer ear (pinna and ear canal) creates a direction-selective filter; different frequencies are enhanced/attenuated when sound enters our ears from different directions. These effects are highly individual (depending on the shape and size of the outer ear). We are not born with this skill. We must continually learn how sound is affected by the direction it enters our ears by integrating visual and auditory perceptions. Accordingly, abrupt changes in the shape of your outer ear can make it difficult to accurately Recording of a sound played from different localize the elevation of sounds. heights, as detected by a microphone in the cat’s ear. The green trace is shown on every graph to highlight how the timbre of the sound changes. FROM THE EAR TO PRIMARY AUDITORY CORTEX 1. The organ of Corti sends auditory information to the brain via the cochlear nerve. 5 4 2. These axons synapse in the cochlear nuclei of the medulla, where copies of the signal are made to be analyzed in parallel ascending paths. 3b 3. Axons from the cochlear nuclei synapse in the superior olivary nuclei in the medulla and the inferior colliculi in the midbrain, both of which help localize the source of sounds. 4. Axons from the inferior colliculi synapse in 3a 2 the medial geniculate nucleus of the thalamus, which in turn relays the information to the … 1 5. Primary auditory cortex in the temporal lobe. TONOTOPIC REPRESENTATION Like the basilar membrane, the primary auditory cortex is organised according to frequency. Different parts of the auditory cortex respond best to different frequencies. This organisation, where different frequencies of sound are analyzed in different places of auditory cortex, is known as tonotopic representation. AUDITORY CORTEX Primary auditory cortex (core region) is in the upper section of the temporal lobe, mostly hidden in the lateral fissure. The belt and parabelt regions refer to auditory association cortex. Like visual information, auditory information is analyzed in “where” and “what” streams. The posterior (dorsal) auditory pathway is involved in sound localization. This pathway meets up with the “where” vision pathway in the parietal lobe. The anterior auditory pathway is important for recognizing what produced a sound (not the location of the sound). It is sometimes called the auditory object recognition pathway, and it extends from the temporal lobe into the frontal lobe. AUDITORY AGNOSIA Music and language are special, complex forms of auditory processing, and brain damage in auditory association cortex can cause very specific types of auditory agnosia. Different areas of auditory association cortex process the melody, rhythm, and harmony (overtones) of music. Other areas of auditory association cortex are involved in the perception of sound as pleasant (consonant) or unpleasant (dissonant), and certain combinations of musical notes can trigger emotions (happy or sad). Accordingly, there are many different types of auditory agnosias. AMUSIA Amusia is the inability to perceive or produce melodic music. People with amusia might be unable to sing or recognize the happy birthday song. People with amusia can often converse and understand speech. They can also recognize environmental sounds. They can even recognize the emotions conveyed in music, but they will typically be unable to tell the difference between consonant music (pleasant sounding harmony) and dissonant music (unstable, transitional), even though these sounds might alter their emotional state just as they do in other people. VESTIBULAR SYSTEM THE INNER EAR BEYOND THE COCHLEA Vestibular system (detects gravity and tilts and turns Cochlea (detects sound) of the head) The vestibular system doesn’t always produce readily definable, conscious sensations. But it contributes to our sense of balance, maintains an upright head position, and corrects eye movements to compensate for head movements. Disruptions in the vestibular system can cause dizziness and nausea. VESTIBULAR SYSTEM Otolith organs (vestibular sacs): two distinct structures – the utricle & saccule – that monitor the angle of the head and linear acceleration of the head ( ). Semicircular canals - three ring-like, fluid-filled structures that detect changes in head rotation (angular acceleration) In each semicircular canal is one bulge called the ampulla, where a gelatinous mass (cupula) pulls open hair cells in response to movement of fluid in the canals. OTOLITH ORGANS otolith In the otolith organs (the utricle & saccule), it is the weight of a stone of calcium carbonate (an otolith) that pulls open hair cell ion channels to indicate the angle of the head and whether it is accelerating along a linear path. One otolith sits in the horizontal plane, and the other sits in the vertical plane.