Lecture 9: Blood Proteins, Hemoglobin PDF
Document Details
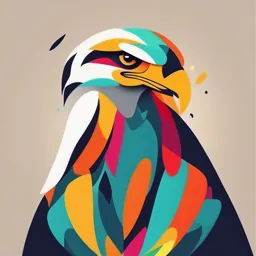
Uploaded by SimplerFoxglove
University of Minnesota
Robert Roon
Tags
Summary
This document provides a lecture overview of blood proteins, with a specific focus on human hemoglobin and other key components like albumin and various globin fractions. The lecture also details the function of these components, including their roles in blood plasma and other bodily processes.
Full Transcript
BioC 3021 Notes Robert Roon Lecture 9: Blood Proteins, Hemoglobin Slide 1. Blood Proteins, Hemoglobin In this lecture, we examine the proteins found in human blood. First, we will survey the extracellular proteins that are found in blood plasma. Then we w...
BioC 3021 Notes Robert Roon Lecture 9: Blood Proteins, Hemoglobin Slide 1. Blood Proteins, Hemoglobin In this lecture, we examine the proteins found in human blood. First, we will survey the extracellular proteins that are found in blood plasma. Then we will look at hemoglobin, the major intracellular protein in human red blood cells. Slide 2. The Major Human Plasma Proteins We will look at five classes of proteins found in human blood plasma: - At a normal concentration of about 4000 mg/100 ml, albumin constitutes about half of the protein in blood plasma. Albumin has two major roles. One is to help stabilize the osmotic pressure of the blood—in this capacity, it simply provides a particle that is retained in the blood and contributes to blood’s osmolarity. In other words, it helps maintain the volume of fluid in the bloodstream by preventing water from diffusing into other tissues. A second role of albumin is to bind fatty acids during their transport through the blood. Albumin helps to solubilize the fatty acids as they travel from one tissue to another, keeping them from forming fatty deposits in the veins and arteries. -At a concentration of 2500 mg/100 ml, the α-globin fraction has the second highest concentration of protein in the blood. Unlike albumin, the α-globin fraction contains many diverse proteins. The common feature of these proteins is that they migrate at a similar speed during certain types of electrophoresis. Proteins in the α-globin fraction include various lipoproteins, protease inhibitors, and proteins that function as hormone inhibitors and in ion transport. There are also proteins that are involved in the body’s inflammatory response. 1 BioC 3021 Notes Robert Roon -The β-globin fraction has a concentration of about 1000 mg/100 ml. This diverse protein fraction contains lipoproteins and other proteins that serve in the transport of iron ions and heme cofactors. -The γ-globulin fraction has a concentration of about 1000 mg/100 ml. There are millions of different antibody molecules in this protein fraction with specific binding sites for a very diverse population of antigen molecules. The γ-globulin antibodies serve as the body’s first line of defense against a variety of foreign organisms and molecules—bacteria, viruses, toxins etc. -Fibrinogen is a single protein species that is present in plasma at about 300 mg/100 ml. Fibrinogen is an inactive precursor protein. It is activated by proteolysis during the blood clotting process. The activated product of fibrinogen proteolysis is fibrin, which forms a fibrous protein network in blood clots. Slide 3. Saturated and Unsaturated Fatty Acids There are two major classes of fatty acids—saturated fatty acids have no double bonds, while unsaturated fatty acids have at least one double bond. An example of a saturated fatty acid is steric acid, which has 18 carbons and no double bonds. An example of an unsaturated fatty acid is oleic acid, which has 18 carbons and one double bond. Fatty acids such as these are carried in the blood by the albumin protein. Slide 4. Albumin Binds Fatty Acids The albumin protein can bind 7 fatty acid molecules. The fatty acids bind reversibly to hydrophobic areas of the albumin molecule. Binding to albumin prevents the very hydrophobic fatty acids from forming undesirable precipitates or plaques in the bloodstream. Slide 5. Lipoproteins Lipoproteins are complexes of protein and lipids that are involved in the transport of phospholipids, triglycerides, and cholesterol 2 BioC 3021 Notes Robert Roon through the bloodstream. The lipoprotein complexes vary in density depending on the percentage of protein and lipid. Proteins have an average density of 1.3 g/ml, whereas lipids have an average density of 0.8 g/ml. The complexes with the highest percentage of protein are the heaviest, and those with the highest percentage of lipid are the lightest. Lipoprotein particles are classified according to their density. -The high density lipoprotein (HDL) has the most protein and the least lipid, and thus has the highest density. -The very low density lipoprotein (VLDL) has the most lipid and the least protein, and thus has the lowest density. -In between these two extremes lies low density lipoprotein (LDL), which has an intermediate level of lipid and protein.. Slide 6. Lipid Transport in the Human Body Lipids (fats) are transported in the body by a special mechanism that prevents their deposition in unhealthy locations, such as the circulatory system. -Ingested triglycerides are transported from the intestine to the tissues in the lymph system. Triglyceride transport is accomplished by large lipoprotein particles called chylomicrons. -A variety of lipids are transported in the bloodstream from the liver to other tissues by means of VLDL particles. -The LDL particles transport lipids to various tissues or to the liver. -The HDL particles pick up lipids from tissues and return them to the liver. Slide 7. Structure of a Low Density Lipoprotein (LDL) Here we see the structure of a low density lipoprotein (LDL) particle. Cholesterol, cholesterol esters, phosphoglycerols, and 3 BioC 3021 Notes Robert Roon triacylglycerols are transported in body fluids in the form of LDL particles. Each particle consists of a core of hydrophobic lipids surrounded by a shell of more polar lipids and proteins. The protein component of these lipoproteins, called apoprotein, acts as a cell-targeting signal. Slide 8. Uptake of LDL Particles. The figure shows how LDL particles are taken up into tissues by receptor-mediated endocytosis. The LDL particles are recognized by cell surface receptors. The binding of LDL particles to these receptors triggers the uptake of the particles into the cell. Following uptake, the various LDL constituents are released into the cell and broken down into their component parts. These breakdown products are then used for biosynthetic reactions. Slide 9. Electron Micrographs of Lipoprotein Particles Lipoprotein particles vary in size, composition, density and function. Chylomicrons are the largest and least dense, while high density lipoproteins (HDL) are the smallest and most dense. Very low density lipoproteins (VLDL) and low density lipoproteins (LDL) are intermediate in size and density. Slide 10. Major Classes of Human Lipoprotein Particles This table gives the biochemical composition of the major lipoprotein particles. Chylomicrons have the highest percentage of triacylglycerols and the lowest percentage of protein. Conversely, HDL particles have the lowest percentage of triacylglycerols and the highest percentage of protein. Slide 11. Lipid Transport in the Human Body Here we see the lipid transport scheme again. We will examine some of its features in more detail. Slide 12. Lipid Transport in Lipoprotein Particles 4 BioC 3021 Notes Robert Roon Lipids are released from the liver and intestines. These lipids circulate through the body in the blood. They are initially transported in VLDL particles. The lipid contents of VLDL particles are gradually transferred to muscle and adipose cells. Thus, the density of lipoprotein particles gradually increases. As lipids are released, the VLDL particle first becomes an IDL I dont write checks 6/18/11 6:24 PM particle and then an LDL particle. Some LDL particles bind to Comment: What is an IDL? LDL receptor proteins in target cells and are taken up by endocytosis. Other LDL particles are returned to the liver for reprocessing. Slide 13. High Density Lipoprotein (HDL) The high density lipoprotein (HDL) fraction carries cholesterol in the bloodstream. A newly formed HDL particle contains no cholesterol. The HDL particle gradually picks up cholesterol during circulation. The HDL particle returns cholesterol to the liver or transfers it to LDL particles. Clinical studies have confirmed that high HDL levels correlate with a reduced risk of cardiovascular disease. Slide 14. Cholesterol Structure Cholesterol is a very non-polar lipid molecule containing three fused six-membered rings and one fused five-membered ring. It is not aromatic and not planar. The only somewhat polar features of cholesterol are its double bond and single hydroxyl group. Slide 15. Acyl-CoA Cholesterol Acyltransferase (ACAT) Cholesterol can be converted to a cholesterol ester by esterification to a fatty acid. This reaction is catalyzed by Acyl-CoA Cholesterol Acyltransferase. The cholesterol ester is even less polar than cholesterol itself. Cholesterol esters are found in the fatty plaques that cause atherosclerosis. Slide 16. Lipoprotein Levels and Cardiovascular Disease 5 BioC 3021 Notes Robert Roon Clinical studies have shown that high HDL levels correlate with a decreased risk of cardiovascular disease (more HDL means more cholesterol is removed from the circulation). For this reason, HDL is sometimes called "good cholesterol". Conversely, high LDL correlates with increased risk of cardiovascular disease. Therefore, LDL is sometimes called "bad cholesterol". (Various medical studies have shown that the ratio of HDL to LDL is a good predictor of heart disease—in fact, it is a better indicator than the total cholesterol level.) Because cholesterol is synthesized in the body and is also ingested in the diet, both sources of cholesterol must be controlled in order to minimize the level of LDL in the body. Slide 17. An Artery Partially Occluded Here is an artery with about 50% occlusion with fatty plaque that is loaded with cholesterol esters. This is not good. Slide 18. An Artery with More Occlusion Here is an artery that is about 75% occluded with cholesterol ester material. This is even more not good. Slide 19. A Totally Occluded Artery This artery is about 85% occluded with fat, and the remaining area has been blocked with a blood clot. This is what happens in heart attacks and strokes. Needless to say, you do not want this to happen in your arteries. On the positive side, this is more likely to be me than you at this time. Oh! To be young again! Slide 20. Genetic Disorders Promote Atherosclerosis -Familial hypercholesterolemia is a hereditary condition in which there is a defect in LDL receptors, which reduces or prevents the ability to carry out receptor mediated endocytosis. -Familial HDL deficiency results from a lack of the protein ABC1. In cells lacking the ABC1 protein, there is a lack of uptake of 6 BioC 3021 Notes Robert Roon cholesterol by HDL particles, and the cholesterol-poor HDL particles are rapidly destroyed. Slide 21. Red Blood Cells (RBC’s) Red Blood Cells (RBC’s) constitute about 40% of the volume of human blood. They are highly differentiated cells whose major function is to transport oxygen from the lungs to the tissues. These little biconcave discs are loaded with the hemoglobin protein. The RBC’s are red because oxygenated hemoglobin is red. Hemoglobin is red because its heme cofactor is red. Slide 22. Hemoglobin Carries Oxygen in the Blood The solubility of O2 is low in blood plasma. In order to transport O2 efficiently, some transport mechanism is required. Almost all of the O2 in the bloodstream is carried inside of Red Blood Cells by the Hemoglobin protein. The O2 is carried on an Fe+2 ion in the heme group of the hemoglobin. Hemoglobin is a tetrameric protein with four chains (α2β2) and a heme group on each chain. Hemoglobin is closely related to myoglobin, an O2 binding protein found in muscle. Unlike hemoglobin, myoglobin has only one chain and one heme group. Slide 23. Features of the Hemoglobin Protein and Its Heme Cofactor Cells constitute about 45% of the blood volume, and 90% of those cells are RBC’s. The RBC’s contain about 32 percent protein by volume, and 99 percent of that protein is hemoglobin. Hemoglobin has a molecular weight of about 65,000. Hemoglobin is a tetrameric protein with four chains (α2β2) and a heme group on each chain. Although hemoglobin’s major function is to transport oxygen, it also transports carbon dioxide and hydrogen ions. Slide 24. Heme Structure 7 BioC 3021 Notes Robert Roon We are going to be discussing the oxygen carrier proteins myoglobin and hemoglobin. Both of these proteins contain heme cofactors. This diagram shows the type of heme cofactor/prosthetic group found in these proteins. The heme group is a tetrapyrrole structure with a ferrous iron ion in its center. The tetrapyrrole group has a series of alternating single and double bonds, which give it the ability to delocalize electrons. The functional groups that are located on the corners of the pyrrole rings also contribute to the electronic structure of the heme cofactor. Interaction of the organic tetrapyrrole structure with the iron ion promotes ability of the iron ion to bind molecular oxygen. Slide 25. Myoglobin Structure Myoglobin is the first protein to have its structure determined by X-ray crystallography. The structure of myoglobin was determined by John Kendrew. The protein consists of a-helixes and turns, and does not have beta sheets. The single subunit of myoglobin has a three dimensional shape, which is very similar to both the alpha and beta subunits of hemoglobin. That similarity in structure was very helpful to Max Perutz when he investigated the much more complicated tetrameric structure of hemoglobin. He could use the data obtained for myoglobin to help visualize the structure of the four subunits of hemoglobin. Slide 26. Amino Acid Sequences of Myoglobin and Hemoglobin. The top line in this diagram shows the amino acid sequence for myoglobin. The middle line gives the sequence of the alpha subunit of hemoglobin, and the bottom line corresponds to the amino acid sequence of the beta subunit of hemoglobin. The blue boxes indicate positions in these molecules in which the amino acids in the alpha and beta chains of hemoglobin are exactly the same. The boxes outlined in red indicate positions in which the 8 BioC 3021 Notes Robert Roon amino acids in myoglobin and the alpha subunit of hemoglobin are exactly the same. When this type of sequence similarity occurs, we say that these proteins have a high degree of homology. The explanation for such homology is that all of these proteins are derived from the same evolutionary globin precursor molecule. It is thought that during evolution, myoglobin branched off from the globin precursor first, and a hemoglobin precursor branched off later. That hemoglobin precursor then was duplicated, and the two proteins gradually diverged from one another to eventually give the alpha and beta subunits. The degree of difference in the sequence homology is one measure of the time after branching off during which genetic drift occurred. Slide 27. Superimposed Structures of Myoglobin and Hemoglobin Alpha and Beta Chains The figure shows that, although there are many differences in amino acid sequence between myoglobin and the alpha and beta chains of hemoglobin, their overall structures are very similar. Slide 28. Hemoglobin Structure In this figure, we see a colorful display of the four subunits of hemoglobin. The molecule is viewed from two different angles. In the tetrameric protein structure, an α-subunit is in contact with a β-subunit, which is in contact with another α-subunit, which is in contact with another β-subunit, which finally is in contact with the original α-subunit. Thus, the α-subunits do not contact each other, and similarly the β-subunits do not contact each other. If you were sufficiently sharp of eye, you would see that each of the four subunits has its own heme ring attached to it. Slide 29. Hemoglobin: an Allosteric Protein In normal adult hemoglobin, oxygen binding is cooperative. The oxygen binding curve for hemoglobin is sigmoidal, or S-shaped, in contrast to the normal hyperbolic curve associated with the binding 9 BioC 3021 Notes Robert Roon of ligands to most proteins. When one subunit in hemoglobin binds oxygen, a conformational (allosteric) change occurs in the whole complex, and the other subunits exhibit an increased affinity for oxygen. The binding of the first oxygen thus influences the binding of the next three oxygens in a favorable way. This process is called positive cooperativity. Slide 30. Conversion of Hemoglobin from the Tense State (T) to the Relaxed State (R) This diagram shows a model that has been proposed for hemoglobin cooperativity. According to the model, all four subunits exist in either a tense (T) state or a relaxed (R) state. The T state subunits have relatively poor oxygen binding ability, whereas the R state subunits bind oxygen more tightly. This is called a concerted model for oxygen binding. In it purest form, the concerted model would demand that, upon the binding of one oxygen molecule, all four subunits of hemoglobin would switch to the R state, and all four subunits would exhibit the same very strong oxygen binding characteristics. Slide 31. Hyperbolic Oxygen Binding Curve This is a hyperbolic binding curve for oxygen that one would observe for myoglobin. It looks similar to the V vs [S] curve for a typical enzyme, although in this case, we are looking at binding vs [S]. Slide 32. Oxygen Binding Curves for Myoglobin and Hemoglobin The figure compares the oxygen binding curves for myoglobin and hemoglobin. It turns out that these two proteins have very different roles for human metabolism and very different oxygen binding characteristics. Myoglobin functions in muscle tissue. Its role is to pick up oxygen released by hemoglobin and to hold onto it until it is needed for 10 BioC 3021 Notes Robert Roon metabolic purposes. Myoglobin exhibits a classical hyperbolic oxygen binding response. In contrast to myoglobin, which holds oxygen in tissues until it is needed, hemoglobin binds oxygen when blood diffuses through the lungs and releases oxygen when blood circulates in the tissues. The hemoglobin molecule needs to be able to pick up oxygen efficiently from the capillaries of the lungs, but it also needs release oxygen efficiently to the tissues. The oxygen binding response of hemoglobin is sigmoidal (S-shaped), which is characteristic of an allosteric protein. We will see that this oxygen binding response plays a key role in oxygen binding and release. Slide 33. Partial Pressures of Oxygen in Various Compartments of the Body The data in this figure give the partial pressures of oxygen in various compartments of the body. At sea level, inspired air has a partial pressure of about 160 mm Hg. Alveolar air (in the lungs) has a partial pressure of 100 mm Hg. In venous blood, that pressure drops to 40 mm Hg (or less during vigorous exercise). In the tissues, a typical partial pressure is about 10-20 mm Hg. What this means is that hemoglobin should be able to pick up oxygen in the lungs at a partial pressure of 100 mm Hg and drop it off in the tissues at pressures that range from 10-40 mm Hg. Slide 34. Oxygen Binding Curves for Myoglobin and Hemoglobin The area shaded in orange gives the range of partial oxygen pressures in the lungs. The area shaded in blue gives the range of partial oxygen pressures in the tissues. Because of its sigmoidal oxygen binding properties, hemoglobin is excellent at binding oxygen in the lungs (almost 100% loaded) and releasing oxygen in the tissues (30-60% loaded). In contrast, myoglobin would not release much oxygen in the tissues (it would remain 90-95% loaded). 11 BioC 3021 Notes Robert Roon Slide 35. The Oxygen Binding Site of Hemoglobin The ferrous iron atom in the heme ring of hemoglobin is associated with a histidine imidazole group of the F helix. In the absence of oxygen, the iron atom lies out of the plane of the heme ring. When oxygen binds to hemoglobin, the atomic radius of the iron atom shrinks and the iron slides into the plane of the heme ring. The associated histidine imidazole group is pulled toward the heme ring, and the movement of that histidine imidazole draws the F α- helix closer to the heme ring. Slide 36. Effects of Oxygen Binding to the Heme Iron The figure illustrates how the atomic radius of the heme iron shrinks upon oxygen binding, allowing the iron to move into the plane of the heme ring. The movement of the iron ion causes the attached histidine residue to move in toward the face of the heme ring. Slide 37. Embryonic, Fetal and Adult Hemoglobin The predominant form of hemoglobin varies during different stages of human development. In embryos, the major form of hemoglobin is HbE (ζ2ε2). At a later stage during fetal development, the major form of hemoglobin is HbF (α2γ2). In adults, the major form of hemoglobin is HbA (α2β2). Slide 38. Synthesis of Hemoglobin Subunit Variants There are two α-type hemoglobin subunit variants and three β-type subunit variants. With respect to α type subunits, the ζ subunits predominate in the embryo for about three months after conception, and then they are replaced with α subunits. The α subunits persist in the adult. With respect to β-type subunits, the ε subunits predominate in the embryo for about three months after conception, and then are replaced with γ subunits during the next six months of 12 BioC 3021 Notes Robert Roon development. At about nine months of gestation, around the time of birth, the γ subunits are replaced with adult β subunits. Slide 39. Chromosomal Location of Hemoglobin Genes All of the genes for α-type hemoglobin subunits are clustered on chromosome #16. The genes for β-type subunits are clustered on chromosome #11. Slide 40. Gene structure of Hemoglobin Subunit Variants. All of the variant genes that code for hemoglobin subunits have two intervening sequences (introns). That is, they have two regions of DNA sequence that do not code for the final protein and are removed from the mRNA before protein synthesis occurs. It is interesting that the location and size of these introns are quite similar for all of these hemoglobin variant genes. Slide 41. Evolutionary Development of Hemoglobin Genes Evolutionary biologists suggest that all current hemoglobin genes are derived from the same ancestral globin gene precursor. This ancestral family tree shows where the hemoglobin variants branched off from each other during evolution. At some point, there was a gene duplication resulting in two separate globin genes, one of which developed into an a-type gene and the other into a b- type gene. At some later point, the two genes migrated to different chromosomes. Slide 42. The pH Affects Oxygen Binding by Hemoglobin A decrease in pH promotes O2 release from hemoglobin. This is called the Bohr effect after its discoverer. The physiological importance of this phenomenon is related to the acidifying influence of carbon dioxide in the tissues. Because of the high concentration of carbon dioxide in the tissues, the pH is lower than in the lungs. This lower pH decreases the affinity of hemoglobin for oxygen and promotes oxygen release in the tissues. 13 BioC 3021 Notes Robert Roon Slide 43. 2,3 Bisphosphoglycerate (BPG) Affects O2 Binding to Hemoglobin There is a small metabolite called 2,3 Bisphosphoglycerate (BPG), which is associated with hemoglobin. One BPG molecule binds per hemoglobin tetramer. The binding site is at the center of the hemoglobin tetramer. At that location, BPG is in contact with eight positively charged amino acid groups. The BPG contacts Lys82, His2, His143, and the terminal amino group of both β chains. The BPG stimulates O2 release from hemoglobin by stabilizing the quaternary structure of deoxy-hemoglobin. In effect, this increases the sigmoidal (allosteric) nature of hemoglobin. Slide 44. Interaction of BPG with Hemoglobin This figure shows the binding site for BPG at the center of the hemoglobin tetramer. The BPG interacts primarily with the β- subunits of hemoglobin. Slide 45. Mode of BPG Binding to Hemoglobin On this slide, we see the interactions that occur between the negative charges of BPG and the positive charges of Lys82, His2, His143, and the terminal amino group of both β chains. Slide 46. Influence of BPG on Oxygen Binding to Hemoglobin In essence, the presence of BPG promotes the characteristic sigmoidal oxygen binding curve of hemoglobin. In the absence of BPG, the oxygen binding curve looks more hyperbolic—much like the binding curve observed for myoglobin. Slide 47. Fetal Hemoglobin Lacks Sensitivity to BPG The role of fetal hemoglobin is to accept O2 from the maternal blood. To accomplish this, O2 must bind more tightly to fetal hemoglobin than to maternal hemoglobin. Fetal hemoglobin has an altered subunit composition (α2γ2). The γ subunit differs from the 14 BioC 3021 Notes Robert Roon β subunit at His143. The γ subunit exhibits reduced affinity for BPG, leading to better 02 binding. Slide 48. Comparison of Normal and Sickled RBC’s Before we move on, it might be instructive to consider a pathological condition that results from a genetic error in hemoglobin structure. Sickle cell anemia is the first genetic disease for which a molecular cause was determined. Under the normal label is the amino acid sequence of the N-terminal end of the beta chain from normal hemoglobin (HbA), and under the sickle-cell label is the corresponding sequence from sickle cell hemoglobin (HbS). Individuals who have the genetic disease sickle cell anemia, have a single amino acid substitution at position number six in the beta chain. Because of a genetic mutation, a negatively charged glutamate, which normally occurs at the sixth position in the beta chain, is replaced with a neutral valine in the sickle cell protein. That results in a hydrophobic or sticky patch on the surface of the sickle cell beta chains. Under certain conditions, that sticky patch can cause the hemoglobin S tetramers to polymerize and form long needles containing thousands of hemoglobin molecules. Those needles can distort the shape of the red blood cells, and in some cases can lead to extensive cell lyses. The term sickle cell anemia refers to the loss of iron that occurs when stickled blood cells burst and the hemoglobin is degraded. The slide shows three normal red blood cells with the typical biconcave disc shape, and one sickled red blood cell. The sickled cells can function normally in oxygen transport, but they are much more susceptible to lysis than normal cells. The result of continuing red blood cell lysis is that there is significantly less oxygen carrying capacity in a person with sickle cell anemia. Slide 49. Electrophoretic Profiles for Hemoglobin Fractions The upper panel shows an electrophoretic profile for normal adult hemoglobin. The major peak, which corresponds to hemoglobin A, 15 BioC 3021 Notes Robert Roon contains about 97% of the protein. The smaller peak, corresponding to hemoglobin A2, contains about 3% of the protein. Hemoglobin A2 is a variant that is encoded by an alternate gene. On the lower left is a hemoglobin profile for someone with sickle cell disease. Both of their hemoglobin A genes have been mutated into hemoglobin S genes. The major peak contains the genetic variant hemoglobin S. There is also a significant peak of fetal hemoglobin, hemoglobin F. The expression of the fetal hemoglobin gene is up regulated because of the sickle cell anemia. There is also a small peak for hemoglobin A2. On the lower right is a hemoglobin profile for someone with sickle trait. Such persons are heterozygous, carrying one normal and one sickle cell gene. Persons with sickle cell trait will synthesize slightly more that half normal levels of hemoglobin A, a significant amount of hemoglobin S, and low levels of hemoglobin. Slide 50. Polymerization of Sickle Cell Hemoglobin Under deoxygenated conditions, sickle cell hemoglobin S tends to polymerize. The substitution of a valine for glutamate in sickle cell hemoglobin S causes a hydrophobic, sticky spot on the hemoglobin, giving the molecules an abnormal affinity for each other. This effect is most pronounced under low oxygen conditions. If RBC’s get trapped in capillaries under deoxygenated conditions, the hemoglobin S molecules slowly polymerize. Once a critical mass of about ten molecules is formed, further polymerization is very rapid. Slide 51. Deoxyhemoglobin S fibers The final result of deoxyhemoglobin S polymerization is the formation of long fibrous needle-like polymers. This figure shows an artist’s rendition and an electron micrograph of deoxyhemoglobin S fibers. These fibers can become so long and numerous that they will distort the RBC’s until the cells assume 16 BioC 3021 Notes Robert Roon the characteristic sickle cell shape. Ultimately, the RBC’s lyse, leading to loss of oxygen carrying capacity in the blood—anemia results. Slide 52. Electron Micrograph of Deoxyhemoglobin S Fibers Here is a dramatic look at deoxyhemoglobin S fibers. The cross section view gives us some idea of how numerous these fibers are in a deoxygenated RBC. Slide 53. Lyses of a Sickle Cell In this electron micrograph, the RBC has become so distorted with deoxyhemoglobin S fibers that cell lyses has occurred. Some of the fibers have spilled out into the surrounding extra cellular fluid. 17