Exercise Physiology - Muscle Metabolism PDF
Document Details
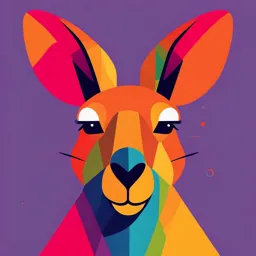
Uploaded by NourishingPorcupine
Faculty of Physical Therapy - MSA University
Tags
Summary
This document covers exercise physiology and muscle metabolism. It discusses various energy sources, including carbohydrates, lipids, and proteins, and their roles during exercise. Understanding how muscles use different energy sources allows tailoring exercise intensity to optimize training outcomes.
Full Transcript
Exercise Physiology Muscle metabolism Muscle metabolism Energy sources ENERGY STORES CONTROL OF ENERGY SOURCES The Effect of Exercise Intensity and Exercise Duration on Substrate Metabolism and Muscle Recruitment during Acute Exercise Skeletal muscle comprises ~...
Exercise Physiology Muscle metabolism Muscle metabolism Energy sources ENERGY STORES CONTROL OF ENERGY SOURCES The Effect of Exercise Intensity and Exercise Duration on Substrate Metabolism and Muscle Recruitment during Acute Exercise Skeletal muscle comprises ~40% of total body mass in mammals and accounts for ~30% of the resting metabolic rate in adult humans. How do muscle cells obtain the energy to perform exercise? Introduction Energy sources during exercise ATP and CP – alactic anaerobic source (PCr + ADP Creatine kinase ATP +Cr) Glucose from stored glycogen in the absence of oxygen – lactic anaerobic source Glucose, lipids, proteins in the presence of oxygen – aerobic source Muscle metabolism Exercise intensity Low ATP and creatine phosphate stimulate glycolysis creatine and oxidative ADP, Pi, phosphate in skeletal muscle phosphorylation. cells Exercise can increase rates of ATP formation and Glycolysis Oxidative phosphorylation breakdown more than tenfold ENERGY STORES Carbohydrates Lipids Muscle Carbohydrates Carbohydrates are an important energy source for both intense and prolonged exercise, and are stored as glycogen in both the muscle where it is needed as an energy source and in liver where the glucose is pushed out into the blood. In comparison with lipid stores, carbohydrates are limited with a total amount of approximately 500 g in the body. The muscle contains around 300 g of glycogen, although this store can increase to 500 or 600 g when carbohydrate loaded, whilst the liver glycogen stores are about 150 g in total. The liver glycogen stores are also affected by diet and exercise in so far as these stores are enhanced by high carbohydrate feeding and depleted by either prolonged exercise or fasting. Indeed, after an overnight fast the liver glycogen stores can be severely depleted The amount of energy contained within the carbohydrate stores can be estimated by multiplying the total amount in grams by 3.75 or 16, which are the energy content of a carbohydrate in kcal or kJ respectively. Therefore a 300 g muscle glycogen content contains 1125 kcal or 4800 kJ of energy. Lipid stores Lipid stores Lipid stores are, in contrast to carbohydrates, a major store of energy, since each fat molecule contains 9 kcal (38 kJ) of energy. This is more than twice the energy density found in carbohydrates and so makes lipids a useful, compact storage source. Lipids are stored as triglycerides, which are essentially a glycerol molecule that is attached to three fatty acids. (Fig. 2). Major stores of triglycerides can be found in adipose tissue, although they may also be found inside muscle cells. Although triglycerides are the storage form of lipids, fatty acids are the useable form from a metabolic perspective. Triglycerides are produced when there is an excess amount of fat intake or when there is an excessive amount of carbohydrate ingested. The fatty acids from the digested food become attached to a glycerol molecule to produce triglycerides. In the case of excessive amounts of carbohydrate ingested, the glucose is used to produce both glycerol and fatty acids, which together make up a triglyceride molecule To estimate the total amount of energy in fat stores it is first necessary to determine how much fat there is. This can be done by estimating the % body fat using either skinfold measures or DEXA scan or by underwater weighing. The average % body fat for young males varies between 10 and 20% and for females between 20 and 30%. If we take a male weighing 70 kg and with an estimated body fat of 15%, then the estimated total fat content is 10.5 kg (i.e. 15% of70 kg). To find out the energy content it is necessary to multiply the fat mass in grams by 9 or 38 to get values in kcal or kJ. Such a calculation shows that the energy content is 94 500 kcal or 399 000 kJ, a plentiful supply of energy. Even in those athletes with extremely low body fat % such as elite male road cyclists with values around 5% body fat, there is sufficient energy to undertake extremely prolonged exercise. Fatty acids are catabolized via β-oxidation and then entry to the Krebs cycle and the ETC. If it is fully oxidized a typical fat (palmitate) yields 129 molecules of ATP. However, the rate of ATP resynthesizes from fat is too slow to be of great importance during high intensity activity. protein The major stores of metabolic protein are muscles. Of course proteins include all enzymes, peptide hormones, molecules such as hemoglobin and myoglobin, and are an integral part of all membranes. However, these sources are not broken down to be used as energy. The total mass of potentially usable protein is about 8 kg, although this depends on the muscle mass of an individual. Protein provides 4 kcal (17 kJ) of energy per gram, and so if there were 8 kg of protein, this would provide 32 000 kcal (136 000 kJ) of energy. The problem with using protein as an energy source is that it means the muscle is cannibalizing itself to provide energy. Traditionally, protein is not considered to contribute to energy provision except under conditions of starvation or in ultra- endurance events (Ultra-endurance is the term given to events that last for over 6 hours). CONTROL OF ENERGY SOURCES Metabolic regulation: Hormones and cyclic AMP Allostatic regulation: Some molecules found in cells are able to promote or inhibit the activity of regulatory enzymes. Metabolic regulation: Metabolism is regulated through control of key enzymes involved in energy producing or energy-storage processes. The control is mediated by hormones which, via cAMP, activate inactive enzymes. However, enzyme activity can also be affected by allosteric effectors. Hormones and cyclic AMP: Hormones are chemical substances originating from glandular cells which are then transported through blood to a target cell to influence physiological activity. Examples of key hormones which influence the metabolic activity of a cell include adrenaline (epinephrine), noradrenaline (norepinephrine), insulin, and glucagon. Cyclic AMP (cAMP) is a ubiquitous intracellular effector molecule produced from ATP by the enzyme adenylate kinase. Cyclic AMP activates a protein kinase within cells, which in turn activates inactive enzymes. In some instances (e.g. glycogen synthase) cAMP production inhibits the activity of an enzyme. Production of cAMP occurs as a consequence of hormonal activation. Allostatic regulation: Some molecules found in cells are able to promote or inhibit the activity of regulatory enzymes. Such molecules, known as allosteric effectors, do not bind to the active site of an enzyme and as such are distinct from competitive inhibitors ENERGY FOR EXERCISE OF VARYING INTENSITIES At lower intensities lipid oxidation dominates, but with increasing exercise intensity there is greater reliance on muscle glycogen and blood glucose. During moderate-intensity exercise, the contributions from carbohydrate and fat are roughly equal (50/50). High-intensity exercise :Carbohydrates are the only macronutrients that can be used as an energy source during high-intensity exercise. Muscle glycogen is broken down in the cytoplasm during intense exercise to produce energy and lactic acid Prolonged steady-state exercise :Muscle and liver glycogen stores are used to provide energy during prolonged exercise. Depletion of liver glycogen can result in hypoglycemia, whereas depletion of muscle glycogen results in the inability to sustain an exercise intensity above 55% V· O2max Intermittent exercise :Carbohydrates are an important source of energy during intermittent bouts of exercise, in particular the more intense intervals. At low-to-moderate intensities of exercise, the primary fuel sources supplying skeletal muscle are glucose, derived from hepatic The partitioning of glycogenolysis (or gluconeogenesis) or oral ingestion, and free fatty acids (FFAs) liberated by adipose tissue lipolysis. The contributions of liver and fuel sources adipose tissue to substrate provision to contracting muscle during exercise utilized from extra- are largely under the influence of hormonal responses to the onset of exercise (e.g., adrenaline, noradrenaline, glucagon, insulin, cortisol). or intramuscular With respect to extra muscular fuel sources, as exercise intensity substrates increases, muscle utilization of circulating FFAs declines modestly, is coordinated whereas utilization of circulating glucose increases progressively up to quantitatively and near-maximal intensities. This coincides with increasing absolute rates of CHO oxidation and relative contribution to energy provision (Figure 3A), with temporally to meet a majority of energy at high intensities of exercise being provided by muscle the metabolic glycogen. demands of When exercise at a fixed intensity is prolonged (>60 min) (Figure 3B), an increasing energy contribution is derived from lipid oxidation. exercise. Consequently, the proportion of energy derived from muscle glycogen ed from extra- or declines and is replaced by a progressive increase in plasma FFA oxidation. intramuscular substrates is coordinated 21 1-High-intensity exercise High-intensity exercise is exercise that is non-steady-state exercise, and as such is likely to be maintained for slightly longer than 5 minutes before fatigue ensues. This means that such exercise can be maximal and last for a few seconds or slightly more prolonged. The major sources of energy for high intensity exercise are ATP, PCr, and muscle glycogen. All these energy sources reside in the cytoplasm and can be used rapidly from processes that take place in the cytoplasm. Fats are unable to be used for such intense exercise because they are only broken down in the mitochondria. Fatigue, which may be defined as the inability to sustain the necessary exercise intensity associated with high-intensity exercise is most often considered to be due to lactic acid accumulation and the associated increase in acidity and reduction in pH due to H+ ions. This is because lactic acid dissociates into lactate ions and hydrogen ions, and it is the hydrogen ions that cause the decrease in pH: lactic acid (Hla) → La− + H+ Introduction Muscle fatigue Lactic acid ↓ATP (accumulation of ADP and Pi, and reduction of creatine phosphate) → → ↓ Ca++ pumping and release to and from SR→↓ contraction and relaxation Ionic imbalances →muscle cell is less responsive to motor neuron stimulation Introduction Lactic acid ↓ the rate of ATP hydrolysis, ↓ efficiency of glycolytic enzymes, ↓Ca2+ binding to troponin, ↓ interaction between actin and myosin (muscle fatigue) during rest is converted back to pyruvic acid and oxidized by skeletal muscle, or converted into glucose (in the liver) lactate threshold The lactate threshold occurs for a number of reasons. 1. As exercise intensity increases so does the recruitment of fast glycolytic fibers which favor lactate production. 2. As exercise intensity increases the rate of glycolysis increases above that of the TCA cycle and hence more of the pyruvic acid formed is converted to lactic acid. 3. As exercise intensity increases there is a limited delivery of oxygen to muscle and hence a greater chance of anaerobiosis lactate threshold is used in prediction of performance and as a marker of exercise intensity Mechanisms to Explain the Lactate Threshold Fig 4.10 26 Other Mechanisms for the Lactate Threshold Failure of the mitochondrial Type of LDH hydrogen shuttle to keep pace with glycolysis Excess NADH in sarcoplasm favors Enzyme that converts pyruvic acid to conversion of pyruvic acid to lactic acid lactic acid LDH in fast-twitch fibers favors formation of lactic acid 27 lactate threshold The reasons why the lactate threshold graph shifts to the right are because endurance training results in: 1. increased lactate removal and reduced lactate production 2. increased capillarization of muscle to enable greater oxygen delivery 3. increased mitochondrial density and hence more oxidative enzymes to reduce lactate production and enhance lactate removal 4. slow oxidative and intermediate fibers are particularly influenced in being more oxidative. 2-Prolonged steady state exercise The exercise intensity enabling prolonged activity is dependent on the level of training. The major energy source during such exercise is initially carbohydrate from muscle glycogen and blood glucose, but as the exercise progresses there is a greater dependence on fatty acids either from intramuscular triglyceride stores from adipose tissue. Fig. 2 shows the changes in fat and carbohydrate use during exercise lasting for 60 minutes, and clearly demonstrates the switch from predominant carbohydrate to predominant fat oxidation after around 20 minutes. It takes this long because of the need to stimulate lipolysis and then release the fatty acids under the influence of the hormones adrenaline (epinephrine) and insulin. Increases in adrenaline (epinephrine) and a fall in insulin result in these changes Respiratory exchange ratio (RER). (Respiratory quotient (RQ). ) A simple way of determining the contribution of carbohydrate and fat to total oxidation is by measuring oxygen uptake and the respiratory exchange ratio (RER). The latter is also referred to as the respiratory quotient (RQ). The distinction between RQ and RER is that the former reflects CO2 production and O2 consumption in homogeneous tissue whereas the latter reflects whole body CO2 production and O2 consumption. RER = An RER of 1 reflects exclusive use of carbohydrates whilst an RER of 0.7 reflects only fats being used as an energy source. Fig. 3 shows how the relationship between RER and exercise intensity affects RER and thereby carbohydrate and fat use. volume of carbon dioxide produced volume of oxygen consumed Estimation of Fuel Utilization During Exercise Indicates fuel utilization 0.70 = 100% fat 0.85 = 50% fat, 50% CHO 1.00 = 100% CHO During steady-state exercise VCO2 and VO2 reflective of O2 consumption and CO2 production at the cellular level 33 Fatigue for prolonged exercise is due to hypoglycemia, muscle glycogen depletion, or dehydration. The first two reflect the important contribution of carbohydrates for exercise. Elevated muscle glycogen level after 3 days of a high carbohydrate diet results in an enhanced exercise time to exhaustion. Also a low muscle glycogen concentration results in an attenuated time to exhaustion Fig. 5 shows that hypoglycemia may be a cause of fatigue as can be seen at point A where the blood glucose level had fallen to around 3 mM. At this time the subject was given 100 grams of glucose and then was able to exercise for a further 40 minutes before fatigue ensued. At the second point of fatigue, blood glucose was above 4 mM and so hypoglycemia could not have been the cause 3-Intermittent exercise Many sports require the athlete to engage in repeated bouts of high-intensity activity with lower levels of activity. A game of soccer for example consists of 1% sprinting, 4% cruising, 32% jogging, 48% walking, and 15% standing still. In other words, the game of soccer draws approximately 95% of its total energy from aerobic sources and 5% from anaerobic sources. This means that the majority of the energy arises from carbohydrate and fat stores After the cessation of exercise, the metabolic rate declines but remains slightly elevated (