Lecture 4: Biotechnology (PMP-404) PDF
Document Details
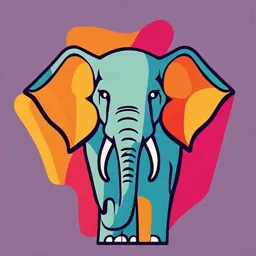
Uploaded by WarmerCerium
New Mansoura University
Dr. Enas Yasser Sultan
Tags
Summary
This document is a lecture on biotechnology, focusing on microbial enzymes and their applications in various industrial processes. Specific examples of enzymes and their uses in different industries like dairy and detergents are detailed.
Full Transcript
Biotechnology (PMP-404) Level 4 Pharm D Lecture 4 Dr. Enas Yasser Sultan Lecturer of Microbiology and Immunology Industrial processes and products I. Microbial enzymes Enzymes from microbial sources 1. Microorganisms are the most significant and conv...
Biotechnology (PMP-404) Level 4 Pharm D Lecture 4 Dr. Enas Yasser Sultan Lecturer of Microbiology and Immunology Industrial processes and products I. Microbial enzymes Enzymes from microbial sources 1. Microorganisms are the most significant and convenient sources of commercial enzymes. 2. Abundant quantities of enzymes under suitable growth conditions. 3. Microorganisms can be cultivated by using inexpensive media and production can take place in a short period. 4. In addition, it is easy to manipulate microorganisms in genetic engineering techniques to increase the production of desired enzymes. 5. Recovery, isolation and purification processes are easy with microbial enzymes than that with animal or plant sources. Industrial processes and products I. Microbial enzymes Different organisms contribution in the production of enzymes 1. Fungi: 60% 2. Bacteria: 24% 3. Yeast: 4% 4. Streptomyces: 2% 5. Higher animals: 6% 6. Higher plants: 4% Aspergillus niger There are well over 40 commercial enzymes that are conveniently produced by A. niger. These include a-amylase, cellulase, protease, lipase, pectinase, catalase and insulinase. Application of bulk microbial enzymes A. Detergent enzymes The incorporation of enzymes into detergents provides several benefits: 1. Energy savings (lower wash temperature can be used) 2. Reduction of levels of less desirable detergent chemicals. 3. Environmentally safe. 1959, The first commercial use of microbial enzymes in detergents: a new product containing a bacterial protease, which replaced the animal trypsin that had previously been incorporated into these detergent products. 1960, Subtilisin: a bacterial alkaline serine protease from B. subtilis Protein-engineered subtilisins: This enzyme has now been engineered to improve pH and temperature characteristics Proteases are not the only enzymes used in detergents; since the late 1980s, amylases and lipases have been available for incorporation. Lipolase: the first detergent enzyme to be produced through recombinant DNA technology. Cutinase: from Fusarium solani. Superior fatty acid digesting enzymes have now been discovered B. Dairy products 1. Production of cheeses, yogurt and other dairy products 2. Improve texture or flavor. The more common types of enzymes and their role in the dairy industry are: 1. Coagulant Enzymes 2. Lactase 3. Lipases 4. Bio protective Enzymes 1. Coagulant Enzymes (proteases): Break down the milk protein (kappa casein), resulting in the formation of a cheese curd. 1.1. Microbial Coagulants are proteases such as Mucor pepsin (Rhizomucor miehei) and endopesin (Cryphonectria parasitica). more difficult process and give cheese a bitter flavor Adv.: Low-priced coagulants Break down additional milk proteins other than kappa casein. 1.2. Fermentation-Produced Chymosin (FPC) Highly-purified chymosin A. niger Adv.: Lower cost Reduced bitterness Higher production yield than other coagulants. 2. Lactase: Milk contains the sugar lactose which must be broken down by the enzyme lactase prior to digestion. Lactose intolerance 1. Lactose intolerance may be overcome by consuming dairy products in smaller quantities 2. Consuming dairy products containing low levels of lactose such as yoghurt. 3. Lipase: Unpasteurized milk Breaking down milk fat to free fatty acids (aged cheese). Inactivated by pasteurization, resulting in a more bland flavor in aged, pasteurized cheeses. 4. Bio-protective enzymes: Improve food safety Reduce microbial contamination, so increase shelf life Types: 1. LYSOZYME: antimicrobial enzyme which limits growth of Clostridia in aged cheeses. 2. NISIN: antimicrobial peptide effective against Gram+ ve and spore-forming bacteria in cheese 3. LACTOPEROXIDASE: antimicrobial enzyme used to preserve raw milk. C. Starch processing enzymes and related carbohydrases 1. α-Amylase: is one of the most important of these industrial enzymes. This endo-enzyme generates glucose, maltose and maltotriose units. Species of Aspergillus and Bacillus. Uses: Starch processing and baking, where starch liquefaction and dextrin hydrolysis are required. 2. Glucoamylase: A. niger Starch and dextrins glucose 3. Glucose isomerase: Many bacteria, including species of Bacillus and Streptomyces. Glucose fructose Fructose has the same calorific value as glucose, but its sweetening effect is approximately twice as high. 4. Invertase : S. cerevisiae preparations. Other yeasts or filamentous fungi: A. niger and A. oryzae. Sucrose glucose + fructose Uses: 1. Apart from syrup production, 2. Employed in manufacturing of candy. Soft centered chocolates (solid sucrose- based filling, containing some invertase, and coating with chocolate). Within 2 weeks the center becomes converted into a fructose/glucose syrup. 5. Lactase: Lactose glucose + galactose Applications: 1. Syrup production: 4 fold sweetness 2. Milk and dairy product processing: lactose reduction/removal for those who are lactose intolerant 3. Ice-cream manufacture: prevention of ‘sandy’ texture caused by lactose crystals. Industrial processes and products II. Health-care products The other major health-care products derived from microbial fermentations and/or biotransformation are alkaloids, steroids, toxins and vaccines; along with vitamins and certain enzymes, a wide variety of mammalian proteins and peptides (insulin, interferon, GH and monoclonal antibodies). Most antibiotics are secondary metabolites. The best known and probably the most medically important antibiotics are the β-lactams, penicillins and cephalosporins; along with aminoglycosides, such as streptomycin, and the broad-spectrum tetracyclines. 1. Antibiotics A. Penicillin The basic structure of the penicillins is 6-aminopenicillanic acid (6-APA), composed of a thiazolidine ring fused with a β-lactam ring whose 6-amino position carries a variety of acyl substituents. Commercial production of penicillin Condition source Operating fed batch , submersion, STR mode Organism Penicillum chrysogenum temperature 25–27°C 6.5–7.7 by calcium carbonate (1%, w/v) and a pH phosphate buffer to neutralize the medium carbon glucose, lactose sources Nitrogen Corn steep liquor sources vegetable oils Ammonia, mineral salts and specific side-chain others precursors, e.g. phenyl acetic acid or phenoxyacetic acid, may also be added Commercial production of penicillin Phase I: Growth phase (vegetative phase) Adding lyophilized spores to a small fermenter at a concentration of 5x10^3 spores/ml. Glucose as carbon source Fungal mycelium is grown up through one or two further stages until there is sufficient to inoculate the production fermenter This high growth rate is maintained for the first 2 days. Phase II: Production phase The mycelium is transferred to another medium containing lactose which is fed at a low rate. penicillin production increases. This continues for a further 6–8 days Penicillin is excreted into the medium and is recovered at the end of this phase otherwise it starts to degrade. Phase III: penicillin recovery 1. Penicillin recovery by removal of mycelium using rotary vacuum filters. 2. Recovered mycelium is then washed to remove residual penicillin, prior to its use as animal feed or fertilizer. 3. Antibiotic recovery is often by solvent extraction of the cell- free medium, which gives yields of up to 90%. This involves reducing the pH of the filtered medium to 2.0–2.5 by addition of sulphuric or phosphoric acid, followed by a rapid extraction at 0– 3°C using amyl acetate. Alternatively, ion-pair extraction may be used at pH 5–7. Any pigments and trace impurities are removed by treating with activated charcoal. 4. The penicillin is then precipitated from the solvent by addition of sodium or potassium acetate and it precipitates as a sodium or potassium salt. Resultant penicillin crystals are separated by rotary vacuum filtration. 6. Penicillin crystals are washed with a volatile solvent (ETOH, isopropanol, butanol), to remove further impurities, then dried. 7. Purity (99.9%) The basic structure of the penicillins is 6-aminopenicillanic acid (6-APA), composed of a thiazolidine ring fused with a β- lactam ring whose 6-amino position carries a variety of acyl substituents. In the absence of added side-chain precursors to the fermentation medium of P. chrysogenum : a mixture of natural penicillins is obtained from culture filtrates, notably penicillin G (benzyl penicillin) and the more acid-resistant penicillin V (phenoxymethyl penicillin). These penicillins are most active against G +ve bacteria. By the addition of side-chain precursors to the fermentation medium: different biosynthetic penicillins. These semisynthetic penicillins, such as methicillin, carbenicillin and ampicillin, exhibit various improvements: Resistance to stomach acids to allow oral administration Degree of resistance to penicillinase Extended activity against some Gram -ve bacteria. Production of semi-synthetic penicillins It involves removal of the side chain of the base penicillin to form 6-APA. This is achieved by passage through a column of immobilized penicillin acylase, usually obtained from E. coli, at neutral pH. Penicillin G: gives 6-APA and phenyl acetic acid. The 6-APA is then chemically acylated with an appropriate side chain to produce a semisynthetic penicillin. B. Production of cephalosporins Consequently, as 6-APA can also serve as a precursor of cephalosporins, it is often used as the starting material for their semisynthetic production. A base natural penicillin is converted to 6-APA, as described before, followed by its conversion to the preferred precursor, 7-amino-deacetoxy-cephalosporic acid (7-ADCA), by ring expansion. A suitable side chain can then be readily attached Ring expansion 2. Steroid biotransformation WHY FUNGAL TRANSFORMATION? 1. Growth Rate: Higher growth rate of fungal immobilized cells reduces the time of biomass transformation. 2. Metabolism Rate: Higher rate of the metabolism in fungal cells due to specific enzymes regulate efficient transformation of substrate. 3. Sterility: It is easier to maintain sterile conditions when fungal cells are used. 4. FUNGAL transformations cleave the complex side chains of precursor steroids in one single step and incorporate desirable modifications in steroid nucleus. (hydroxylations at positions 11 and 17; various side- chain cleavages, hydrogenations and dehydrogenations; and ring expansions from a five-membered to a six-membered ring). 5. Easy Purification and Isolation Biotechnology