DNA Replication Lecture Notes PDF
Document Details
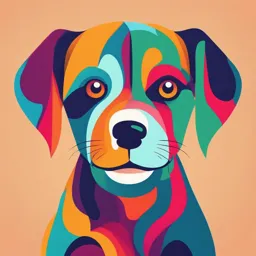
Uploaded by IdolizedLandArt
Tags
Related
- DNA Structure, Replication, and Repair (Lippincott's Illustrated Reviews Biochemistry 5th Edition) PDF
- DNA Structure, Replication, and Repair (Lippincott's Illustrated Reviews Biochemistry 5th Edition) PDF
- Deoxyribonucleic Acid (DNA) Structure and Function 2024-2025 PDF
- DNA Structure and Replication PDF
- Bio Sci H97 Lecture #2, DNA Structure and Replication PDF
- DNA Structure and Properties PDF
Summary
This document provides an overview of DNA replication, including different types of DNA. It discusses the denaturing and melting of DNA, calculations related to melting temperature, and the process of supercoiling in DNA. It also covers the structure of DNA in cells and the role of DNA polymerases in the process.
Full Transcript
Reversible denaturing of DNA When a DNA sample is heated, the helix begins to separate, in a process sometimes referred to as melting (The temperature at which half of the DNA is single stranded is called the melting temperature Tm) Since AT base pairs have only two hydrogen bonds holding them toget...
Reversible denaturing of DNA When a DNA sample is heated, the helix begins to separate, in a process sometimes referred to as melting (The temperature at which half of the DNA is single stranded is called the melting temperature Tm) Since AT base pairs have only two hydrogen bonds holding them together, regions of DNA with a high level of A and T residues will separate first. The melting temperature of all DNA molecules is not the same, and depends upon the length of DNA, and the proportion of GC and AT base pairs that it contains. Generally, short DNA molecules have low Tm values, as do those that contain a high proportion of AT base pairs. The melting temperature of a long DNA molecule can be calculated according to the following equation: Tm = 16.5(log[Na+]) + 0.41(%GC) + 81.5 ◦C short DNA molecules (15–30 bases long) are often used in genetic engineering experiments. For short regions of complementation, the equation above breaks down, and the following estimation (sometimes called the Wallace rule) is used to determine the Tm Tm = 2(AT) + 4(GC) every AT base pair in the duplex contributes 2 ◦C of stabilization the double helix, while every GC pairing contributes 4 ◦C of stabilization. Therefore, an oligonucleotide of 20 bases of single- stranded DNA containing five A residues, six T residues, three C resides and six G residues will have an annealing temperature to its complementary DNA sequence of approximately 2(11) + 4(9) = 58 ◦C. The heating of double-stranded DNA to produce the single-stranded version is an entirely reversible process. Single- stranded DNA made by heating a duplex will reform when the temperature is reduced. As the temperature falls, the thermal energy that was used to break the hydrogen bonds between the strands is reduced, and random collisions between the complementary strands will result in their re-association. Providing that the temperature is reduced relatively slowly (1–2 ◦C per second) complete duplex formation will result. The two complementary single- stranded DNA molecules will come together and reform the exact duplex that was present before the sample was heated If the temperature is dropped rapidly, for example by plunging a DNA sample at 94 ◦C directly into ice, correct base pairing will not occur and the DNA will remain in a relatively single- stranded form. Structure of DNA in the Cell Different types of nucleic acid are used to form the genome of organisms depending on the organism itself. For example, viruses have a genome composed of either double-stranded DNA, single- stranded DNA or RNA, depending on the type of virus. The chromosomes of most eukaryotes are composed of single linear double- stranded DNA molecules. The genomes of prokaryotic organisms are generally composed of a circular DNA molecule. That is, rather than having free 5- and 3- ends, the ends are joined to each other to form a continuous ring of double- stranded DNA. A number of extra-chromosomal DNA molecules, called plasmids, are found in prokaryotic cells. Plasmid DNA molecules are usually closed circles of either single-stranded or double-stranded DNA. Electron microscopy images of DNA molecules indicate that DNA is more string-like than rod- like, and will wrap around itself to form a variety of irregular structures. Inside a eukaryotic cell, DNA is associated with a vast array of proteins. Many of these proteins wrap DNA around themselves, or in other ways constrain or bend the DNA molecule. Stresses placed in one part of a DNA molecule by, for example, twisting the double helix can be relieved by untwisting another part of the DNA. The free ends of the linear DNA allow relatively free rotation of the DNA strands. For circular DNA molecules, however, these stresses can prove extremely problematic. Since the DNA has no free ends, it cannot simply untwist to counteract a twist elsewhere in the molecule. Twisting DNA molecules results in the formation of DNA supercoils Supercoiling can only be introduced into or released from a closed-circular DNA molecule by breaking at least one of the phosphodiester backbones. The level of supercoiling within a particular DNA molecule can be described by its linking number (Lk). This number corresponds to the number of doublehelical turns in the original linear molecule The process of introducing supercoils into a DNA molecule reduces or increases the number of helical turns trapped when the circle is formed, and results in changes in the linking number to the final closed- circular species. The linking difference (Lk) gives an indication of the overall levels of supercoiling within a molecule Closed-circular DNA isolated from prokaryotic cells is negatively supercoiled. Eukaryotic cells contain linear DNA molecules the state is defferent They possess enzymes, called DNA topoisomerases, which make cuts in DNA molecules – either single- or double-stranded breaks in the phosphodiester backbone – to allow local twisting and untwisting of the DNA chain. The topoisomerase enzyme then reseals the DNA backbone to reform the DNA molecule. The Eukaryotic Nucleosome Each cell within our body contains a huge amount of DNA chromosomes of the human genome contain approximately 3.2 × 10^9 base pairs of DNA. Since we are diploid organisms , the total amount of DNA in most of our cells totals 6.4 × 10^9 base pairs At 0.33 nm per base pair, this corresponds to an overall length of approximately 2.1 m The answer is that the DNA is highly compacted. It is associated with a number of proteins that results in the wrapping of DNA into nucleosomes. In eukaryotes, however, a substantial amount of protein is associated with the DNA to form chromatin , chromatin fibres are composed of linear arrays of spherical particle called nucleosomes (ν-bodies) The digestion of chromatin with certain nucleases, such as micrococcal nuclease, yields DNA fragments that are approximately 200 bp in length, If the digestion of chromatin DNA were random, then a wide range of fragment sizes would be produced. This therefore demonstrates that the DNA of chromatin consists of repeating units that are protected from enzymatic cleavage. The DNA between the units is attacked and cleaved by the nuclease, and multiples occur where two or more units are joined together. The proteins associated with DNA in chromatin are divided into basic, positively charged histones and less positively charged non- histones. Histones contain large amounts of the positively charged amino acids lysine and arginine , making it possible for them to bind through electrostatic interactions to the negatively charged phosphate groupsof the DNA nucleotides. the histones play the most essential structural role. A nucleosome core particle consists of two copies each of histones H2A, H2B, H3 and H4 to form a histone octamer around which ~150 base pairs of DNA are wrapped in a left-handed superhelix, which completes about 1.7 turns per nucleosome Almost 150 bp of DNA wrap around the octamer core, forming approximately two turns Histone H1, functions to seal the DNA around the nucleosome. The formation of nucleosomes represents the first level of packing, whereby the DNA is reduced to about one-third of its original length. Instead, the 10 nm chromatin fibre is further packed into a thicker 30 nm fibre, which was originally called a solenoid The formation of the 30 nm fibre creates a second level of packaging, in which the overall length of the DNA is reduced some two fold. In the transition to the mitotic chromosome, still another level of packing occurs. The 30 nm fibre forms a series of looped domains that further condense the structure of the chromatin fibre. The fibres are then coiled into the chromosome arms that constitute a chromatid, which is part of the metaphase chromosome. In the overall transition from fully extended DNA helix to the extremely condensed status of the mitotic chromosome, a packaging ratio of about 500:1 must be achieved. The remainder of the packing arises from the coiling and folding of the 30 nm fibre. The tight packing of the DNA into a chromosome presents an enormous challenge to both the replication of DNA and to its transcription. The Replication of DNA each of the parental DNA strands could act as a template for the synthesis of a new DNA strand that would result in the production of two identical double-stranded DNA duplexes. In this model, each of the newly synthesized DNA molecules consists of one parental and one newly synthesized DNA strand; hence, the process is known as semi-conservative replication. Two other modes of replication that also rely on the parental strands serving as templates for new DNA synthesis were also considered. In conservative replication, the parental helix would be opened to reveal the base sequence, and a newly synthesized helix would be produced. The two newly synthesized DNA strands would come together to form a helix and the parental helix would reform. In the second alternative mode, called dispersive replication, the parental DNA from one strand could be dispersed into either of the newly synthesized strands, which would then be a mixture of new and old DNA. (Meselson and Stahl, 1958) DNA Polymerases Both bacteria and eukaryotic cells possess multiple DNA polymerase enzymes. E. coli contain three such enzymes. The first to be isolated, now called DNA polymerase I, is primarily involved in DNA repair rather than DNA replication. DNA polymerase II is also involved in DNA repair, while DNA polymerase III is the main replicating enzyme DNA polymerase III is a multisubunit enzyme that functions as a dimer of these multiple subunits Many DNA polymerase enzymes have been studied at the structural level, which, combined with extensive biochemical analysis, has led to the elucidation of their molecular mechanism of action. DNA polymerase enzymes can possess three distinct enzymatic activities. All DNA polymerases direct the synthesis of DNA fragments in a 5 to 3 direction. Many DNA polymerases also possess 3 to 5 exonuclease activity. This enables the enzyme to remove nucleotides at the 3- end of the newly synthesized chain. This is often considered a ‘proof-reading activity’, which can be used to excise incorrectly incorporated nucleotides before their replacement with the correct bases. Some DNA polymerases also have a 5 to 3 exonuclease activity. This allows the enzyme to remove sequences that have already been synthesized and, is involved in joining up discontinuous DNA fragments produced on the lagging strand during DNA replication. DNA polymerases are not able to initiate DNA synthesis on their own. They have an absolute requirement for a free 3-end onto which the enzyme can add new nucleotides. This means that two primers are required to initiate DNA replication in each direction from the origin – one complementary to each DNA strand to be replicated. DNA synthesis only proceeds in a 5 to 3 direction. This leads to a problem. Both strands of the parent DNA molecule must be replicated, but only one of the strands is orientated in a 5 to 3 direction from the origin of replication. This strand, called the leading strand, can be copied in a continuous manner – DNA replication can occur without stopping as the DNA polymerase is working in its favored orientation. Replication of the other strand, the lagging strand, would appear to have to occur in the 3 to 5 direction, which is not possible. The lagging strand is replicated in a discontinuous fashion strand synthesis, a series of short DNA segments are produced in a 5 to 3 direction (Okazaki fragment) that are then ligated together to produce the intact newly synthesized strand. reverse transcriptase. This enzyme is found in certain viruses (called retroviruses) that contain RNA The Replication Process Initiation of replication Elongation. Termination. Recombination