Respiratory Physiology: Pulmonary Mechanics PDF
Document Details
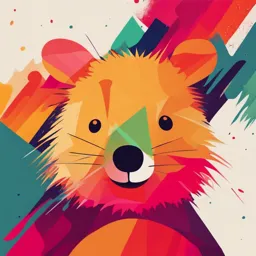
Uploaded by UnquestionableKremlin
Rutgers University
Krista N. Blackwell, Ph.D.
Tags
Summary
This document is a lecture or presentation on respiratory physiology, specifically focusing on pulmonary mechanics. It covers topics like cellular respiration, external respiration, the respiratory system's components, gas exchange, and pulmonary function testing.
Full Transcript
Respiratory Physiology Krista N. Blackwell, Ph.D. Email: [email protected] At the end of this lecture you should be able to: Define cellular and external respiration and list the functions of the respiratory system Recognize the components of the upper and lower airways of the respiratory sy...
Respiratory Physiology Krista N. Blackwell, Ph.D. Email: [email protected] At the end of this lecture you should be able to: Define cellular and external respiration and list the functions of the respiratory system Recognize the components of the upper and lower airways of the respiratory system Identify the components of the respiratory system and describe their role in normal breathing – the muscles of respiration and thoracic cage – the visceral and parietal pleura – the pleural space Categorize the branching of the lungs as described by the conducting zone and the respiratory zone Describe the composition and structure of the alveoli Identify the role of the alveolar-capillary membrane in gas exchange of the lungs Define Dalton’s Law and be able to calculate the partial pressures of gases in the atmosphere Recognize the partial pressures of oxygen and carbon dioxide in the lungs, arterial and venous blood At the end of this lecture you should be able to: Describe Charles Law and its relationship to lung volumes and normal breathing Explain Boyle’s Law and describe its relationship to pressure and volume changes when air moves into and out of the lungs Define intra-alveolar (intrapulmonary) pressure, intrapleural pressure and transmural(transpulmonary) pressure and identify their normal values during a normal inspiration and expiration Identify the changes in intra-alveolar and intrapleural pressures and the changes in airflow and lung volumes during the normal breathing cycle List the muscles of inspiration and expiration ; identify their role during normal breathing or with a forced (active) inspiration or expiration Define and describe the volumes and capacities of the lung Identify the volumes and capacities that can be measured using spirometry Describe the FEV1/FVC ratio in pulmonary function testing Identify the indirect methods for the measurement of FRC and RV – Helium Dilution – Body Plethysmography At the end of this lecture you should be able to: Diagram the alveolar and intrapleural pressure changes that occur during inspiration and expiration. Define anatomic dead space and diagram how it affects ventilation. Compare and contrast total pulmonary ventilation and alveolar ventilation. Explain why gas composition in the alveoli remains relatively constant during normal breathing and demonstrate how it might change during other breathing patterns. Identify the static compliance of the lungs. Give examples of disease states can increase or decrease lung compliance. Explain the role of pulmonary surfactant in normal lung function. Identify the factors can affect airway resistance and describe the local factors and autonomic control mechanisms that can alter airway resistance(bronchodilation and bronchoconstriction). Identify the normal flow-volume loop and the effort-dependent and effort independent portions of the expiratory flow curve Identify the factors can affect airway resistance and describe the local factors and autonomic control mechanisms that can alter airway resistance (bronchodilation and bronchoconstriction). Identify the normal flow-volume loop and the effort-dependent and effort independent portions of the expiratory flow curve Respiration in Physiology Cellular respiration: the biochemical pathway by which cells release energy from the chemical bonds of food molecules and provide that energy for the essential processes of life External Respiration: the movement of gases between the environment (atmosphere) and the body’s cells The exchange of air between the atmosphere and the lungs (ventilation) The exchange of oxygen and carbon dioxide between the lungs and the blood The transport of oxygen and carbon dioxide by the blood The exchange of gases between blood and the cells Other functions of the Respiratory System Acid-Base Balance: increases in carbon dioxide lead to increases in hydrogen ion concentration (and vice versa) Pulmonary Defense Mechanisms: the lungs protect the body from atmospheric environmental assaults (bacteria, dust, particles of silica or asbestos, toxic gases, smoke (cigarette and other types), and other pollutants) Upper Airways Components of the Respiratory System Tracheobr onchial Tree (Lower Airways) Components of the Respiratory System Components of the Respiratory System Conducting and Respiratory Zones of the Lungs The lungs are the “human exchange organ” which consist of gas – A vascular tree (the arteries and veins connected by the capillaries) – An airway tree (a series of hollow branching tubes that decrease in diameter with each branching) The airway tree consists of a conducting zone and a respiratory zone The conducting zone (trachea, bronchi and bronchioles) has the following functions – To warm and humidify inspired air – To distribute air equally to the deeper parts of the lungs – To serve as a part of the body’s defense system Conducting and Respiratory Zones of the Lungs The conducting zone has its own circulation, the bronchial circulation No gas exchange occurs in the conducting zone, which makes this portion of the lung the anatomic dead space The respiratory zone is the site of gas exchange and is composed mainly of alveolar ducts and alveoli The respiratory zone has its own distinct circulation, the pulmonary circulation – 70 – 80% of the pulmonary capillaries occupy the alveolar surface area Conducting and Respiratory Zones of the Lungs Gas exchange in the respiratory units occur at the alveolar-capillary membrane, which forms the blood gas-interface In the respiratory zone, a group of alveolar ducts and their alveoli merge with the pulmonary capillaries to form respiratory units (~60,000 respiratory units in the lungs) There are several physical laws that describe changes in volume and pressure as air moves into and out of the respiratory system The physical laws that govern the respiratory system are: 1. Boyle’s Law: At constant temperature, the pressure of a given quantity of gas is inversely proportional to its volume. 2. Charle’s Law: the volume of a given quantity of gas is directly proportional to its absolute temperature 3. Dalton’s Law: The total pressure of a gas mixture is the sum of the partial pressures of the individual gases 4. Henry’s Law: At the air-water interface, for a given temperature, the amount of gas that dissolves in water is determined by its solubility in water and its partial pressure in the air. These physical laws can be applied to help explain the mechanics of air movement into and out of the lungs, as well as the diffusion of gases across the blood-gas interface Dalton’s Law, Atmospheric Pressure and Gases in the Atmosphere The air we breathe and live in is the atmosphere and it exerts a pressure known as the barometric pressure – At sea level the barometric pressure is 760 mm Hg (0 cm H20) The total barometric pressure is the sum of the individual partial pressures of the gases in the atmosphere – Dalton’s Law: is the relationship that governs the total pressure exerted by a mixture of gases PB = PN2 + PO2 + PCO2 + PH20 (Outside of the body) – The partial pressures of the individual gases is determined by the fractional concentration of the individual gases (% of gases present) – 21% of the atmospheric air is made of oxygen – 79% of the atmospheric air is made of nitrogen – 0.039% of the atmospheric air is made of carbon dioxide (usually considered 0) – There is no water vapor pressure in the atmospheric air When air is inspired, it is warmed and humidified (water vapor pressure increases) (Inside the body) PB = PN2 + PO2 + PH20 + PCO2 Partial Pressures of Gases in the Body PO 2 PCO2 PN2 PH20 Atmospheric Air 160 0.3 600 0 Inspired Air 150 0.3 563 47 Alveolar Air 104 40 569 47 Expired Air 120 27 566 47 Dalton’s Law: PB = PN2 + PO2 + PCO2 + PH20 Calculation of the Partial Pressures of gases in the atmospheric air PB = 760 mm Hg PN2 =.79(760) = 600 mm Hg PH20 = 0 PO2 =.21(760) = 160 mm Hg PCO2 =.00039(760) = 0.30 mm Hg Calculation of the Partial Pressures of gases in the humidified air (inside the body) PB = 760 mm Hg PH20 = 47 mm Hg PB - PH20 = 760 mm Hg - 47 mm Hg = 713 mm Hg PN2 =.79(713) = 563 mm Hg PO2 =.21(713) = 150 mm Hg PCO2 =.00039(713) = 0.30 mm Hg Partial Pressures of Oxygen and Carbon Dioxide in the Body Gas Arterial Blood Venous Blood O2 95 mm Hg 40 mm Hg CO2 40 46 mm Hg H2 O Vapor 47 mm Hg 47 mm Hg N2 571 mm Hg 571 mm Hg Total 760 mm Hg 704 mm Hg The Normal Breathing Cycle and Lung Volumes and Capacities Charles’ Law and the Mechanic of Breathing Charles Law states the volume of a given quantity of gas is directly proportional to its absolute temperature This law relates to the volume of air that enters the lung during a normal inspiration – As the gas enters the lung, the pressure of the gas is the same but the volume of that gas increases when the temperature increases (as it moves from the atmosphere into the body) Boyle’s Law and the Mechanics of Breathing Boyle’s Law states that at a constant temperature, the pressure of a given quantity of gas is inversely proportional to its volume. This law explains the process of pulmonary ventilation that predicts the relationship between pressure and volume changes in the lung with inspiration and expiration When a person inspires, the volume of air in the lungs increases, then the pressure of the gas in the lung decreases (and vice versa) This law is also used to estimate the amount of trapped gas in the lungs using a clinical test known as body plethysmography Pressure Gradients and Normal Breathing During inspiration air enters the lung because the atmospheric pressure is greater than the pressure inside the alveoli (intra-alveolar pressure or intrapulmonary pressure) – Atmospheric pressure = 0 cm H20 – Intra-alveolar (intrapulmonary) pressure = sub-atmospheric (~ -1 cm H20) During expiration, the intra-alveolar (intrapulmonary) pressure becomes greater than atmospheric pressure (+1 cm H20) Pressures During Inspiration and Expiration Pressure Gradients and Normal Breathing During inspiration the intrapleural pressure becomes more negative because the thoracic cavity expands – Intrapleural pressure = -6 cm H20 During expiration the intrapleural pressure becomes less negative because the thoracic cavity decreases in size Intrapleural pressure = -3 cm H20 – Pleural pressures become important when the chest wall has been punctured (pneumothorax), air will enter the pleural space, the lung will recoil immediately and the rib cage will expand outward Pressures Gradients and Normal Breathing Because of the differences in intrapulmonary and intrapleural pressures, a pressure difference exists across the walls of the lung (transmural or transpulmonary pressure) – Transpulmonary/transmural pressure = intra-alveolar (intrapulmonary) pressure – intrapleural pressure The transpulmonary pressure is positive during inspiration and expiration, which helps maintain the coordinated movements of the lung and the chest wall as the lung volume changes Pressure Changes during a Normal Inspiration Events during a Normal Inspiration Inspiratory muscles contract Thoracic cavity expands Pleural pressure becomes more negative Transpulmonary pressures increase Lungs Inflate Alveolar pressure becomes sub-atmospheric Air flows into the lungs until alveolar pressure equals atmospheric pressure Mechanics of a Normal Inspiration Movements of the diaphragm and rib cage change the thoracic volume, which allows the lungs to inflate during inspiration and deflate during expiration During a normal inspiration, – The diaphragm contracts, causing the thoracic cavity to expand and the lungs to inflate – The diaphragm is innervated by the phrenic nerve With a forced inspiration, – The diaphragm and the accessory muscles, the scalene muscles, the sternocleidomastoid and the external intercostals are used to inhale a larger volume of air Inspiratory Muscles of Breathing: – – – – Sternocleidomastoid Scalene muscles Diaphragm (main muscle of breathing) External Intercostals Pressure Changes during a Normal Expiration Events during a Normal Expiration Inspiratory muscles relax Thoracic cavity deflates Pleural pressure becomes less negative Transpulmonary pressure increases (to a lesser degree than during inspiration) Lungs deflate Alveolar pressure becomes atmospheric supra- Air flows out of the lungs until alveolar pressure equals atmospheric pressure Mechanics of a Normal Expiration Expiratory Muscles of Breathing: Expiration is a passive process With a normal expiration, – – – – the diaphragm relaxes the rib cage drops the thoracic volume decreases the lungs deflate With exercise or a forced expiration, the expiratory muscles become active The accessory muscles of expirations are also used when coughing, straining, vomiting and defecating – Internal Intercostals – Internal and External Oblique Muscles – Rectus and transverse abdominis muscles Pneumothorax Pneumothorax occurs when air enters the pleural cavity – This breaks the connection between the visceral and parietal pleura – As a result the chest wall expands and the lungs recoil – Airflow into and out of the lungs is decreased – The intrapleural pressure (normally subatmospheric) becomes atmospheric Pneumothorax can occur from a – Stab wound – Broken rib – Congenital Bleb: rupture of a weakened area of lung tissue that enters the pleural cavity The pneumothorax can be corrected by – Removing the air (via suction pump) – Seal opening (to block the air from entering) and any air that remains will be absorbed into the blood This also restores the pleural fluid bond and reinflating the lung Pulmonary Function TestingSpirometry, FVC and Indirect lung volume measurements The Spirometer Tracing and Lung Volumes Spirometry measures the volume of air that is breathed in or out of the lungs Lung Volumes and their definitions VT (tidal volume): the volume of air that leaves the lungs with a single breath IRV (inspiratory reserve volume): the air that can be inhaled after a normal inhalation ERV (expiratory reserve volume): air that can be exhaled after a normal exhalation RV (residual volume): the volume of air that stays in the lungs The Spirometer Tracing and Lung Capacities Spirometry measures the volume of air that is breathed in or out of the lungs Lung Capacities and their definitions FRC (functional residual capacity): air in the lungs after a normal exhalation IC (inspiratory capacity) : air that can be inhaled into the lungs after a normal exhalation VC (vital capacity) : the volume of air that can be exhaled after a maximal inspiration (TLC – RV) TLC (Total lung capacity): the maximal amount of air in the lungs after a maximal inspiration FEV1/FVC Ratio and Lung Disease A pulmonary function test to estimate the forced vital capacity (FVC) is used in the diagnosis of pulmonary disease This pulmonary function tests estimates how much air can be moved into and out of the lungs The vital capacity can decrease with age, as the muscles weaken and as the lungs become less elastic The FVC can also be used to diagnose two types of chronic pulmonary diseases – Restrictive Lung Disease – Obstructive Lung Disease During the FVC testing, the forced expiratory volume (FEV) at one second can also be determined as the patient blows all of the air out of the lungs In a normal individual, the FEV1/FVC ratio is about 80% (80% of all of the air can be moved out of the lungs in 1-second) In restrictive lung disease, the FEV1/FVC is normal, while with obstructive lung disease the FEV1/FVC is decreased How can residual volume be determined? Indirect Measurements of FRC: Helium Dilution Because the lungs cannot be emptied completely following a forced expiration, neither RV or FRC can be measured directly by spirometry The subject is connected to a spirometer, filled with 10% helium The lungs contain no helium at the start, but as the patient breathes the helium-oxygen mixture, it will equilibrate with the spirometer The volume of air that remains in the lungs (after a normal expiration) is the FRC Indirect Measurements of FRC: Body Plethysmography Because the lungs cannot be emptied completely following a forced expiration, neither RV or FRC can be measured directly by spirometry The helium-dilution and nitrogen washout methods cannot measure trapped gas With body plethysmography, an airtight container is used and the patient breathes in and out through a mouthpiece and tubing As the patient breathes through the closed airway, the chest expands and the pressure in the plethysmograph increases Respiratory Physiology: Pulmonary Mechanics Ventilation of the Lungs Patterns of Breathing Total pulmonary ventilation and minute ventilation are the same The volume of air moving into and out of the lungs each minute, is the total pulmonary ventilation (minute ventilation). VE = VT (tidal volume) X f (breathing rate) = 500 mL X 12 breaths/minute = 6000 mL/minute Total pulmonary ventilation is the air that moves into the anatomical dead space (conducting airways) and into the alveoli The air that remains in the conducting airway, does not reach the alveoli and is known as the dead space volume (VD) Alveolar ventilation is the amount of inspired air that enters the alveoli per minute (the total amount of fresh inspired air available for gas exchange).V = (V – V ) X f A T D. VA = (VT – VD) X f = (500-150)* 12 = 350*12 = 4200 mL/min Alveolar ventilation changes with hyperventilation and hypoventilation Alveolar ventilation – can be changed drastically by altering the rate or depth of breathing Hyperventilation increases alveolar PO2 and decreases alveolar PCO2 Hypoventilation decreases alveolar PO2 and increases alveolar PCO2 – can be changed by altering the composition of inspired air (changes in altitude) Ascent to high altitude, reduces PO2 in the atmospheric air (because barometric pressure decreases) The alveolar air equation estimates alveolar ventilation with hyperventilation and hypoventilation With changes in ventilation, the alveolar air equation can estimate alveolar ventilation using the partial pressures of oxygen, carbon dioxide and the respiratory exchange ratio (R) Alveolar Ventilation can be determined by the simplified alveolar air equation – PAO2 = PIO2 – ( PA CO2 / R) – PIO2 = FIO2 ∙ (PB – 47 mm Hg) PAO2 = alveolar oxygen content FIO2 = fraction of inspired oxygen R = respiratory exchange ratio PIO2 = percent of inspired oxygen PB = barometric pressure Respiratory Exchange Ratio (R): is the output of CO2 by the lungs to the rate of O2 uptake by the lungs – – – – R = VCO2/ VO2 Normal Oxygen uptake (250 mL/min): Normal carbon dioxide uptake (200 mL/min) R = 200 mL/min ⁄ 250 mL/min = 0.8 With hyperventilation R increases because the amount of CO2 exhaled is greater, while with hypoventilation R decreases because CO2 levels increase Physiologic dead space is the anatomic dead space and the alveolar dead space combined In the lungs, the anatomic dead space may not be the only inspired air that does not participate in gas exchange Alveolar dead space is the alveoli that are ventilated but not perfused with pulmonary capillary blood – No gas exchange occurs in these alveoli – A healthy person has little or no alveolar dead space, but a person with a low cardiac output or a pulmonary embolus might have significant alveolar dead space The physiologic dead space is the anatomic dead space and the alveolar dead space combined The Bohr equation uses CO2 levels to determine the physiological dead space – All expired CO2 comes from alveolar gas and none from dead space (negligible amounts of carbon dioxide in the inspired air) X VD(CO2)- physiological dead space VT - tidal volume Pa(CO2) - arterial PCO2 PE(CO2) - expired pCO2 Factors that influence alveolar gas exchange There are two primary factors that affect ventilation of the lungs, lung compliance and resistance within the airways Adequate ventilation of the lung depends on the ability of the lungs to expand normally In order for inspiration to occur, the lungs must be able to expand Lung compliance is the ease of which the lung can be stretched or expand Compliance = change in volume (ΔV) change in transpulmonary pressure (ΔP) The compliance of the lung is determined by the slope of the lung pressure-volume curve Compliance can change with pathophysiology of the lungs – Emphysema (Obstructive Lung Disease) can result in an increased lung compliance – Fibrosis (Restrictive Lung Disease) can result in a decreased lung compliance With inspiration, the lungs have a greater resistance to overcome to expand This diagram illustrates the difference in the compliance of the lung with inspiration and expiration – This difference in the compliance for inflation and deflation is called hysteresis – Hysteresis is due to the recoil from the surface tension of the alveoli At low lung volumes the lungs expand easily, but at high lung volumes, the alveolar walls are already stretched – Thus, at high lung volumes, large changes in pressure yield small increases in lung volumes Respiratory Physiology: Pulmonary Mechanics Surface tension and elastic resistance work to prevent stretching of the lungs In the lungs there is a thin film of fluid on the alveolar surface The water molecules in this fluid are highly attracted to one another and generate the surface tension that must be overcome with breathing In a sphere, like an alveolus, surface tension produces a force that pulls inwardly and creates an internal pressure This relationship between surface tension and pressure is demonstrated by the Law of Laplace T = Pr ÷ 2 According to the Law of Laplace, smaller alveoli have a greater surface tension When two alveoli are connected by a common airway and the surface tension of the two alveoli are equal, then the pressure in the smaller alveolus is greater than that of the large alveolus Because of the higher pressure in the smaller alveolus, the air will empty into the larger alveolus – This phenomenon would lead to airway collapse of the smaller airways Two factors make the alveoli more stable and prevent the collapse of the airway – Pulmonary surfactant – Structural dependence of the alveoli Structural Interdependence occurs because of the anatomical organization of alveoli Because the alveoli are interconnected with surrounding alveoli, they support each other structurally This alveolar interdependence may play a role in the prevention of atelectasis (lung collapse) and keeps the alveoli open Pulmonary surfactant reduces surface tension that occurs with changes in alveolar diameter Pulmonary surfactant is a substance that contains phospholipids (dipalmitoylphosphatidylcholine) and proteins and is continuously produced by type 2 alveolar cells It reduces the surface tension of the fluid that lines the lung and equalizes the pressure differences between small and large alveoli Surfactant is produced during fetal development, from the 24th to the 34th week of gestation (~ 6 weeks prior to delivery) – After 34 weeks surfactant production is sufficient In premature infants (born before 34 weeks), the lack of surfactant can result in lung collapse and cause neonatal respiratory distress syndrome Surfactant reduces the surface tension of smaller alveoli and prevents their collapse Neonatal Respiratory Distress Syndrome (NRDS) Neonatal Respiratory Distress Syndrome: – is caused by a deficiency in pulmonary surfactant in premature infants (low surfactant synthesis, secretion or utilization) – results in atelectasis (lung collapse) and a decreased lung compliance – can lead to the development of pulmonary edema (fluid accumulation) in these premature infants Treatment of NRDS: aerosol administration of artificial surfactant and/or mechanical (artificial) ventilation (positive-pressure ventilation) Testing for NRDS: Amniotic fluid testing in mothers 1. 2. To assess the lecithin: sphingomyelin ratio (tests the levels of glycoproteins, lecithin, sphingomyelin and other proteins which compose surfactant) The PG assay (measures phosphatidylglycerol levels) to assess the risk of respiratory distress syndrome