Introduction To Fatty Acid Synthesis And Breakdown PDF
Document Details
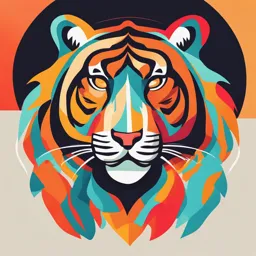
Uploaded by EffortlessCello8490
Ross University School of Medicine
Tags
Summary
This document provides a high-level overview of fatty acid synthesis and breakdown, covering topics such as the physiological sources of lipids, the role of lipoproteins, the importance of adipose tissue, the process of β-oxidation, and the synthesis of ketone bodies. It's suitable for a biological science course.
Full Transcript
Introduction to Fatty Acid Synthesis and Breakdown 1 1. Identify the physiological sources of lipids and fatty acid. 2. Describe the role and hormonal control of lipoprotein lipase and hormone sensitive...
Introduction to Fatty Acid Synthesis and Breakdown 1 1. Identify the physiological sources of lipids and fatty acid. 2. Describe the role and hormonal control of lipoprotein lipase and hormone sensitive lipase in fat metabolism. 3. Describe the importance of adipose tissue Learning in fat metabolism. Objectives 4. Describe the synthesis of fatty acids and triglycerides and their hormonal control. 5. Describe the process of β‐oxidation, the enzymes of importance, and its regulation. 6. Describe the role and synthesis of ketone bodies. 2 Lecture Outline 1. Basics of fat uptake and distribution 2. Fat and fatty acid synthesis 3. Overview of fat mobilization 4. b‐oxidation 5. Regulation of fat mobilization and storage 6. Ketone bodies 3 3 Some Dietary Lipids motor 4 Dietary lipids include mainly: 1. Triglycerides, containing saturated fatty acids (FAs) and unsaturated FAs. 2. Cholesterol and cholesterol esters (the hydroxyl group on cholesterol can be esterified by fatty acids) 3. Phospholipids, from membranes 4. Fat-soluble vitamins (A, D, E, and K) 5. Other hydrophobic compounds and xenobiotics Structures of Some Common Lipids and Detergents Fatty acids arrive on: Triglycerides Cholesterol esters Phospholipids Fats are emulsified by detergents to give enzymes access for degradation. Since fatty acids act as detergents, this is a self‐propagating mechanism. Ferrier: Figures 18.8, 15.1 5 Key points: 1. Cholesterol can be modified to make detergents. This occurs in the liver and futher modifications take place in the gut. One of these is chenodeoxycholic acid. 2. Fatty acids (FAs) are hydrocarbon chains with a carboxylic acid group on one end. 1. This carboxylic acid is often joined to other molecules in ester bonds. 3. Phospholipids are the principal constituents of membranes- see Membrane lipids lecture. Detergents from cholesterol facilitate solubilization (emulsification) of lipids. These include: 1. Cholate 2. Deoxycholate 3. Cholate and deoxycholate modified by taurine and glycine 4. Mono-acyl glycerol (MAG) – formed from breakdown of tryglycerides. MAG can also act as a detergent 5. Lyso-phosphatidylcholine (and other lyso-phospholipids) with only one carbohydrate chain. 6. These detergents, including cholate, bile salts, MAG, FAs, form mixed micelles that helps solubilize fat 7. The detergents provide access for lipases to cleave FAs from triglycerides and cholesterol and phospholipids. 8. This facilitates absorption of the FAs and MAGs into the enterocytes. 5 Fatty Acids Are Liberated From Triglycerides By Lipases Lipase Lipase Triglyceride Diglyceride + Fatty acid Monoglyceride + 2 Fatty acid TG or TAG DG or DAG MG or MAG 6 Lipolysis in the intestine made in Golgi apparatus The major source of fat in the diet is triglycerides, esters of glycerol and fatty acids. Lipases in the intestine degrade triglycerides to free fatty acids and mono- and diacylglycerides. Free fatty acids and MAG are transported into the intestinal epithelium Note: cleavage all the way to glycerol and three free fatty acids is not generally done in the intestines. Instead, in the intestines, the middle position fatty acid is typically the one left on the glycerol, and this MAG is imported into enterocytes. 6 Fat Absorption in the Intestines Intestinal Lumen Fatty Acids Fatty Acids MAG Lipases Chylomicrons TG MAG Bile Acids TAG DAG Proteins Intestinal Epithelial Cell 7 Summary of Fat Absorption 1. Bile, which contains high concentrations of the Bile Acids is delivered to the small intestine. The Bile Acids are cholesterol-like molecules that have polar side chains attached and serve to emulsify the insoluble triglycerides. 2. The triglycerides are broken down to fatty acids and 2-monoacylglyceride by lipases in the intestines and the intestinal epithelium absorbs them. 3. Small fatty acids (FA) can penetrate the membrane without transporters and move into the bloodstream. 4. Triglycerides with short to medium chain fatty acids, such as in coconut oil, also appear to be able to be absorbed directly into the epithelial cells and transferred to the bloodstream. 5. However, most FAs are re-esterified to triglycerides and secreted into the lymph as chylomicrons. 6. Chylomicrons are lipoproteins that are very triglyceride-rich and contain specific apo-lipoproteins that mediate the uptake of chylomicrons by adipose and liver. 7. Chylomicrons carry principally fat (triglycerides). They also carry dietary cholesterol, lipid-soluble vitamins, and other dietary hydrophobic compounds. 7 Chylomicrons Move Lipids from Intestine to Rest of Tissues Chylomicrons: – One type of lipoprotein – Core of triglycerides and cholesterol esters – Monolayer of phospholipid – Apolipoproteins exist in the monolayer: they are structural, but also facilitate delivery of lipids to tissues. http://www.vivo.colostate.edu/hbooks/pathphys/digestion/smallgut/absorb_lipids.html http://en.wikipedia.org/wiki/Chylomicron 8 Chylomicrons: 1. Large lipoproteins that traffic dietary lipids. 2. Chylomicrons are synthesized in the intestine and sent through the lymph system. 3. Delivers triglycerides to adipose and other tissues for storage or direct usage. Remaining lipids, including cholesterol and cholesterol esters get delivered to the liver after most triglycerides are depleted. 4. Digestion and uptake of fat is typically slower than glucose. While insulin remains high, chylomicrons will deliver fat principally to adipose tissue. 5. However, chylomicrons can be made and remain in circulation while insulin is falling, and this can provide fat as an energy source. 6. Triglycerides delivered to the liver in chylomicron remnants are repackaged in VLDL lipoprotein particles and returned to circulation. FED STATE ANAEROBIC (Blood) ENERGY Direct Storage STORAGE Insulin BRAIN MUSCLE TG lipoprotein Lipase LIVER TG CHYLO KIDNEY lipoprotein Lipase ADIPOSE TG INTESTINE Lipoprotein Lipase Action in Capillaries 9 Insulin stimulates fat uptake into adipose tissue. This is done by: 1. Suppressing fatty acid release from existing fat stores 2. Accelerating glycolysis which makes DHAP for glycerol for triglyceride synthesis. 3. Enhancing the level of lipoprotein lipase in the adipose tissue capillary bed (lowered in muscle) Role of lipoprotein Lipase – located in capillaries Role of lipoprotein Lipase – digest triglycerides to free fatty acids – FFAs and releases them for uptake. Lipoprotein lipase is up and downregulated in a highly tissue specific manner in response to insulin, glucagon, and adrenaline. Digest lipoprotein triglycerides to free fatty acids (FFAs) and releases them for uptake. 1. Lipoprotein lipase (LPL) degrades TAG to glycerol and free fatty acids. 1. In the fed state, in the presence of insulin, LPL isozymes are regulated differently: 1. LPL is upregulated in adipose 2. LPL is downregulated in muscle 2. Cardiac muscle has higher LPL than skeletal reflecting its principal 9 reliance on FA for energy. 3. In the fasted state, in the absence of insulin: 1. LPL is upregulated in muscle, by epinephrine 2. LPL is downregulated in adipose 4. LPL is extracellular and is anchored by heparan sulfate to the endothelial cell capillary walls. It is in the lumen of the capillaries. 9 FED STATE ANAEROBIC (Blood) ENERGY Direct Storage STORAGE Insulin BRAIN MUSCLE VLDL TG lipoprotein Lipase TG LIVER Glc TG ADIPOSE AA Gal Fru KIDNEY Pro CHO INTESTINE 10 Fat synthesis during the fed state High Insulin Fat synthesized in the liver from sugars or excess amino acids Generally VLDL production is relatively constant in the liver. It occurs in both the fed and fasted states. Note on VLDL VLDL stands for very low density lipoprotein. It is a lipoprotein, much like chylomicrons with a similar structure, containing a lipid core of triglycerides and cholesterol esters that is surrounded by a phospholipid monolayer with apolipoproteins embedded in it. VLDL serves to deliver triglycerides to peripheral tissues for energy and for storage in adipose tissue. VLDL is made constantly by the liver in both the fed and fasted states. 10 Lecture Outline 1. Basics of fat uptake and distribution 2. Fat and fatty acid synthesis 3. Overview of fat mobilization 4. b‐oxidation 5. Regulation of fat mobilization and storage 6. Ketone bodies 11 11 Energy Metabolism Carbohydrates in the Liver: Fat anabolism (Fed): Fatty acid synthesis NADPH Hexose Monophosphate shunt VLDL (HMP or PPP) Reducing Equivalents (e‐) (NADH, FADH, CoQH2) e‐, Reducing Eq. NADH, CoQH2. Oxidative ATP Phosphorylation 12 Fatty Acid Synthesis 1. Occurs principally in the liver 2. Occurs when there is sufficient carbohydrate influx to raise Acetyl CoA concentrations beyond what is needed for local energy needs. 3. Acetyl CoA is turned into fatty acids and combined with glycerol phosphate to make triglycerides. 4. Triglycerides are packaged into lipo-protein particles called VLDL (very low density lipoprotein). 5. VLDL is exported to the circulation where it will traffic the triglycerides to adipose tissue (when fed). 6. Fatty acid synthesis also requires the following: 1. ATP – from oxidative phosphorylation 2. NADPH – from the Hexose Monophosphate shunt (HMP; also called the pentose phosphate pathway or PPP) 1. Note: the HMP or PPP produces NADPH and also can produce ribose, as ribose-5-phosphate 12 TG Synthesis placeei is Liver Fatty acid synthesistakes Movement of substrate to the cytosol Prepping substrate: made in Acetyl CoA to Malonyl CoA mitochondria Elongation step chemistry Carried out on large enzyme ‐ FAS Glycerol synthesis Assembly of TG and export by VLDL 13 Triglyceride Synthesis Occurs in the Liver The principal place of Triglyceride (TG or TAG) synthesis is the liver. Other tissues can synthesize fat, but do it to a lesser extent Many tissues switch between fat synthesis and beta-oxidation (fatty acid degradation), as a regulation of beta-oxidation. The actual amount of fat made in other tissues is minor compared to liver and to the amount stored in adipose tissue. Fatty Acids Made from Glucose via 00 Acetyl CoA Citrate/Pyruvate shuttle moves Acetyl CoA from mitochondrion to cytosol. o go Fig. 16.11 in Ferrier 14 Substrate Preparation 1. Fatty Acid synthesis occurs in the cytosol. 2. Pyruvate from glycolysis is transported into the mitochondrion as usual. 3. Acetyl CoA is made in the mitochondrial matrix and transported to the cytosol by the Citrate/Pyruvate shuttle. Each turn of the shuttle costs two high-energy phosphate bonds and transfers two electrons from NADH to cytoplasmic NADP+ to generate one cytoplasmic NADPH. The NADPH is used later by the enzyme that makes fatty acids. 4. The shuttle uses the anaplerotic pyruvate carboxylase reaction to make oxaloacetate and then citrate. The pyruvate used for this is regenerated in the cytosol from the exported citrate and re-imported back to the mitochondrion. Note – This supplies SOME of the NADPH! 14 TG Synthesis Liver Fatty acid synthesis Movement of substrate to the cytosol Prepping substrate: Acetyl CoA to Malonyl CoA Elongation step chemistry Carried out on large enzyme ‐ FAS Glycerol synthesis Assembly of TG and export by VLDL 15 Fatty Acid Synthesis Begins from Acetyl CoA Prepping the Substrates Fatty acid synthesis – not synthesis Acetyl CoA to Malonyl CoA – Addition of CO2 – – Uses ATP ↓ Enzyme: Acetyl CoA carboxylase – Highly regulated step 16 Acetyl CoA carboxylase 1) Fatty acid synthesis begins with acetyl-CoA (AcCoA). 2) AcCoA is converted to Malonyl-CoA by acetyl-CoA carboxylase. 3) This is a biotin-containing enzyme and catalyzes the ATP-dependent formation of the carbon-carbon bond between CO2 and Acetyl-CoA. 4) This is the main regulatory point in the switch between fatty acid synthesis and beta-oxidation of fatty acids. 5) Regulation by Insulin and Glucagon! 6) Regulation by AMP Kinase, (AMPK; not shown here). AMPK is a master regulator of metabolism. 16 TG Synthesis Liver Fatty acid synthesis Movement of Substrate to the cytosol Prepping substrate: AcCoA to Malonyl CoA Elongation step chemistry Carried out on a large enzyme ‐ FAS Glycerol synthesis Assembly of TG and export by VLDL 17 Fatty Acids Made in the Cytosol by Fatty Acid Synthase: Active Site and ACP Pantothenic acid vitamins ACP Acyl‐Carrier Protein actiont Coenzyme A 18 Pantothenic Acid: Vitamin B5 Fatty Acid synthesis is catalyzed by a large multi-active site protein, Fatty acid synthase (FAS). To carry the growing fatty acid around to the various activities, the fatty acid chain is synthesized while attached to a long, flexible chain, phosphopantetheine. The phosphopantetheine moiety is also contained in AcCoA, but in FAS, it is bound covalently to the enzyme in the Acyl Carrier Protein (ACP) domain. This long cofactor serves as a flexible arm to carry the synthetic intermediates between the various active sites. Pantothenic acid is Vitamin B5 18 1. Priming with AcCoA Fatty Acid Synthesis: Chemistry ACP 2. Add Malonyl CoA 3.Condensation 4. Reduction 5. Dehydration 6. Reduction 7. Translocation 19 Fatty Acid Synthesis Chemistry – Only know the broad strokes, not the details. 1. Prime the enzyme cysteine site. 2. Add the substrate – Malonyl, to the ACP (acyl carrier protein). 3. Condensation: lengthen the chain by and adding it to the malonyl moiety and loss of CO2. The new chain is on the ACP and has grown by 2 carbons. 4. Ketoreductase: Reduction by NADPH 5. Dehydratase: remove water to form a double bond 6. Enoyl reductase: Reduce the double bond to a single bond using NADPH. 7. Translocation of the new chain to the enzyme cys-SH 8. Repeat steps 2 through 7 until you have a C16 fatty acid. 9. Liberate the chain with thiolesterase. Reduction Dehydration Reduction Note: Fatty acid synthetase makes saturated fatty acids, typically C16. Unsaturated fatty acids and varying lengths are made by other enzymes that can 19 extend the chains and introduce double bond (desaturases). This gives a large variety of fatty acids. However, the enzymes are limited and cannot produce all the required unsaturated fatty acids for arachidonic acid metabolism. So, some poly- unsaturated fatty acids are required in the diet. 19 Synthesis of Triglycerides Liver only: Glycerol Kinase Liver and Adipose 20 Triglyceride synthesis from glycerol and fatty acids: Triglycerides are synthesized in liver and adipose from dihydroxyacetone phosphate (DHAP) and fatty acyl CoA. DHAP, which is an intermediate in the glycolytic pathway, represents an important connection between the glycolytic pathway and triglyceride synthesis. In the Fed State, the flow of glucose through the glycolytic pathway ensures that there are sufficient amounts of DHAP to support triglyceride synthesis. In Adipose tissue, glycerol must be made from glycolysis via DHAP. Liver, but not adipose, can also phosphorylate glycerol to make glycerol phosphate. Glycerol is derived from the breakdown of triglycerides in chylomicrons and VLDL particles in tissues in the Fed State. Like lactate, glycerol can enter the core pathways of energy metabolism in the liver and be used for immediate energy production or for energy storage for future needs. The body reserves the use of glycerol for the liver as a potential gluconeogenic precursor and so that triglycerides will only be made in adipose a tissue while glycolysis is active (fed state with high insulin). Triglyceride synthesis 20 1. The first steps are the same as on phosphoglyceride synthesis. 2. Glycerol 3-phosphate is the start point, after which two fatty acyl chains are added using FA-CoA as the substrate. 3. Phosphatidic acid is converted to diacylglycerol 4. The last fatty acid is added. 20 Fasted STATE ANAEROBIC ENERGY inte (Blood) ‐ Insulin STORAGE + Glucagon + norepi/Epi CO2 BRAIN MUSCLE VLDL TG FA CO2 ADIPOSE LIVER TG CO2 KIDNEY CO2 INTESTINE 21 Fatty acids are stored in adipose as triglycerides and oxidized in most other tissues with the notable exception of brain and anaerobic tissues. 1. FA released by action of Hormone Sensitive Lipase 2. FA carried in blood stream by serum albumin 3. Some FA taken up in target tissues 4. Some FA taken up in liver repackaged to VLDL used for energy and Ketone Body Synthesis VLDL – TG converted to FA by lipoprotein lipase (VLDL LDL) (lipoproteins lecture in S2 AND S4) targets cardiac, skeletal muscle, adipose. Note – How much FA re-esterification to TG and VLDL occurs in the liver is under debate. It may occur in other tissues as well. It is thought as much as 30% is repackaged. 21 Fatty Acids Are Liberated from Triglycerides by Lipases +3 ATGL HSL MAG Lipases Hormone‐sensitive lipase (HSL) is regulated by hormone (Epi/cAMP/PKA mediated). 22 This is the rate‐limiting and determining step of FA release from adipocytes. Enzymes responsible for triglyceride breakdown to fatty acids and glycerol in adipocytes: 1. TAG (TG) is turned to DAG by ATGL (Adipose TriGlyceride Lipase) 2. HSL is regulated by hormone (Epi/cAMP/PKA mediated) – Hormone Sensitive Lipase 3. MAG degraded by monoglyceride lipases. 22 And Release of Free FA from Norepinephrine Adipose Tissue Hormone‐regulated release of FA – FA release stimulated by epinephrine/norepinephrine via PKA‐ mediated phosphorylation. – Release blocked by insulin. 23 Regulation of Free Fatty Acids (FFAs) Fatty acids are stored as triglycerides in adipose. The first step in their oxidation is controlled by hormone-sensitive lipase, which is activated by phosphorylation in response to norepinephrine signaling in the Fasted State (and by epinephrine signaling in the Stressed State). The removal of Insulin is thought to be a key activator (loss of phosphatase action). The decline in insulin also tends to increase triglyceride breakdown in two ways: by decreasing the rate of phosphate removal from hormone-sensitive lipase and by decreasing DHAP, which is the source of glycerol for synthesis of triglycerides. Note: Human adipocytes do not have an appreciable response from glucagon 23 Fatty Acids Are Detergents at High Concentrations: micelle Fatty acids transported in blood bound to albumin albumin FA up to 2 mM 1–20 μM above 2 mM SAFE HAZARD CELLS 24 Fatty acids are carried in the blood bound to the surface of albumin at concentrations up to 2 mM. The concentration of unbound fatty acid ranges from about 1 M to 20 M. Above a concentration of about 2 mM fatty acids exceed the binding capacity of albumin and begin to compromise membrane function. 24 Energy Metabolism in a Typical Cell: Fat Catabolism: (fasting) - b‐oxidation TCA cycle Fatty Acids Reducing Equivalents (e‐) (NADH, FADH, CoQH2) e‐, Reducing Eq. NADH, CoQH2. Oxidative ATP Phosphorylation 25 Major Metabolic Pathways for Fatty Acid Degradation: 1. Beta-Oxidation: The breakdown of fatty acids to Acetyl CoA; this takes place in the mitochondria. It ultimately makes energy (ATP) from fat stores. 2. TCA cycle – Breakdown of Acetyl (Acetyl CoA) to CO2 with production of reducing equivalents (NADH and CoQH2. 3. Oxidative Phosphorylation – electron transport. Converts high energy electrons (reducing power) to ATP and makes water from oxygen. Acetyl CoA is the substrate for the TCA cycle – also a central intermediary: Sources of AcCoA: a. Pyruvate b. Beta-oxidation of fatty acids c. Ketone bodies 25 CARNITINE SHUTTLE Getting FAs to the Mitochondrion Carnitine + FA‐CoA + CoASH Fig. 16.16 Note – Carnitine shuttle inhibited by Malonyl CoA 26 Fatty acids are picked up from blood by fatty acid binding proteins on cell surfaces and transported through membranes. In the cytoplasm fatty acids are converted to fatty acyl CoA to lower the level of free fatty acids and to activate them for transport into the mitochondrial matrix by the carnitine shuttle. Carnitine Palmitoyl Transferase 1 = CPT1 (inhibited by malonyl CoA) Carnitine Palmitoyl Transferase 2 = CPT2 26 B-Oxidation FA being used directly for energy FA AcCoA TCA OxPhos Carnitine shuttle Breakdown in 2‐carbon steps Variations to deal with unsaturation and odd number carbon chains. 27 Mitochondrial Fatty Acid - Oxidation Oxidation: to double bond Hydration: addition of water across the double bond Oxidation: Beta‐carbon hydroxyl to ketone Cleavage of AcCoA group Repeat on shortened fatty acyl chain 28 In the matrix fatty acids are cleaved to acetyl CoA by a repetitive sequence of reactions known as oxidation. As mentioned previously, It resembles the synthesis of fatty acid synthesis in reverse; however, the enzymes are not in a multi-functional protein and are located in the mitochondrial matrix. Basic steps: 1. Acyl CoA dehydrogenase – 1. Oxidizes to a double bond between the alpha and beta carbons. 2. This is a family of chain-length specific enzymes. 3. The product is FADH2 4. FADH2 reducing equivalents are eventually transferred to CoQ via other proteins. 5. There are 4 of these enzymes (described as very long-chain, long-chain, medium-chain, and short-chain variants of Acyl CoA dehydrogenase. There are important deficiencies in each of these. 2. Enoyl CoA hydratase: Hydration across the double bond. 3. 3-hydroxyacyl CoA dehydrogenase: Oxidation of the beta-carbon hydroxyl 4. Beta-Ketoacyl-CoA thiolase: produces Acetyl CoA 5. Go back to step 1 and repeat the cycle until you are done. 28 Very long chain fatty acids are not processed initially by beta-oxidation but oxidized in a less efficient process in the peroxisomes to octanoic acid chains. Peroxisomal disorders can lead to accumulation of very long-chain FA acids (Zellweger Syndrome). 28 Unsaturated Fatty Acids Odd double bonds H H O H3C C C C (CH2)5 (CH2)7 9 SCoA repeated cycles H H O can’t use acyl-CoA H3C dehydrogenase C C C (CH2)5 CH2 SCoA cis 3 enoyl CoA isomerase H O (CH2)5 C C H3C C SCoA CH2 H trans‐2 Enoyl‐CoA same as in saturated fatty acids 29 Dealing with unsaturated FAs. Unsaturated fatty acids can be accommodated in -oxidation pathways, but they require additional enzyme steps that depend on whether the double bond initiates on an odd or even numbered carbon atom. For odd double bonds (C9), when the chain shortens to the place where acylCoA dehydrogenase would desaturate, the double bond prevents desaturation. Instead, the cis 3 enoyl CoA isomerase simply moves the double bond to the 2-position giving the same intermediate as found in the normal -oxidation pathway. One additional enzyme enables us to oxidize unsaturated fatty acids. When a double bond occurs at an even position after the normal pathway has removed two carbons at a time, two double bonds will occur at the 2 and 4 positions. One more enzyme, a 2,4 dienoyl-CoA isomerase will use NADPH to reduce the two double bonds to one and the isomerase will move it into a position and geometry that can be handled by the normal oxidation process. For all unsaturated fatty acids, it requires only two additional enzyme activities to enable us to oxidize any of them, regardless of the double bond positions. 29 Odd-Chain Length Fatty Acids Rare in diet, produced by bacteria in gut O C C15:0 SCoA O H3C C CH2 SCoA propionyl CoA CO2 + ATP TCA Cycle propionyl CoA biotin carboxylase ADP + Pi O H3C methylmalonyl CoA CO2- O C CH SCoA mutase HC 2 C CO2- CH2 SCoA Coenzyme B12 methylmalonyl CoA succinyl CoA 30 Odd-chain fatty acids, which occur at low levels in the diet but are made by intestinal bacteria, yield propionyl (C3)-CoA as a product. Some amino acids are also metabolized to propionyl-CoA. Typically 50-60% of the propionyl CoA comes from amino acid metabolism and much of the rest from odd-chain length fatty acids made by intestinal bacteria. A biotin and vitamin B12 dependent series of conversions metabolizes it to succinyl CoA, an intermediate in the TCA cycle. Sometimes branched chain fatty acids also occur. This can happen because the breakdown of valine, leucine, isoleucine can lead to their incorporation into the ends of fatty acyl chains. This is a rare occurence. The breakdown of these occurs in a similar manner to the breakdown of the amino acids. 30 Lecture Outline 1. Basics of fat uptake and distribution 2. Fatty acid synthesis 3. Overview of fat mobilization 4. b‐oxidation 5. Regulation of fat mobilization and storage 6. Ketone bodies 31 31 Regulation of Fatty Acid Synthesis: Acetyl CoA Carboxylase 32 Fatty acid synthesis begins with acetyl-CoA (AcCoA) which is converted to Malonyl- CoA by acetyl-CoA carboxylase. This is a biotin-containing enzyme and catalyzes the ATP-dependent formation of the carbon-carbon bond between CO2 and Acetyl- CoA Note Regulation by Insulin and Glucagon! through phosphonglation AMPK is considered one of the master kinases due to the plethora of substrates it regulates. See your book for more details. 32 Fatty Acid Synthesis/Oxidation: Regulated by Hormonal Control of Metabolite Signaling via Malonyl CoA Malonyl‐CoA Acetyl‐CoA ADP ATP CO2 ACC2 Fig. 16.16 Note – Carnitine shuttle inhibited by Malonyl CoA 33 Fatty acids are picked up from blood by fatty acid binding proteins on cell surfaces and transported through membranes. In the cytoplasm fatty acids are converted to fatty acyl CoA to lower the level of free fatty acids and to activate them for transport into the mitochondrial matrix by the carnitine shuttle. Regulation of Beta-oxidation occurs by the effect of malonyl CoA on CPT1. When FA synthesis is active, beta-oxidation is inhibited by blocking the action of CPT1; FAs don’t get into the mitochondria. When FA synthesis is not active, beta-oxidation can proceed normally as the default pathway. ACC1 and ACC2 are both highly expressed in the liver where both fatty acid oxidation and synthesis are important. The differences in tissue distribution indicate that ACC1 maintains regulation of fatty acid synthesis whereas ACC2 mainly regulates fatty acid oxidation (beta oxidation). cof down maionly shuts oxidation betr 33 Fatty Acid Release in the FASTED STATE Free fatty acids VLDL 1.0 mM After (FFA or NEFA) overnight FA 0.6 mM Release due to HSL fast KB 0.2 mM activation in adipose Rises steadily once insulin levels drop up to about 0.4 – 0.6 mM in serum after a long fast (overnight). Frayn 34 In the post absorptive state, plasma levels of non-esterified fatty acids rise between meals due to lipolysis of the adipose triglycerides. Non-esterified fatty acids (NEFA, or free fatty acids) 34 Ketone Ketone bodies are made in Bodies response to FFA rise for long periods of time. FFA AcCoA Ketone bodies 35 Glucose/Ketone Use During Fasting amount (g) in 24 hr Fasting Time: 24 hr 3d 40d Brain Glucose Utilization 120 100 40 Ketone Bodies -- 50 100 Other Body Uses of Glucose 50 50 40 Fuel Mobilization Liver Glucose Output 170 150 80 Liver Ketone Body Output ---- 150 150 Table from Stryer Harvey and Ferrier, 5th Ed., Fig 24.12 36 Ketone body utilization by brain decreases the amount of glucose that must be derived from protein degradation and indirectly switches the body energy supply more toward fatty acids. Note – dashes indicate no data. At 24 hours, lipolysis and protein degradation is active. Physiologic ketosis: up to 3 mM without acidosis Ketoacidosis: Higher levels, and can reach over 10 mM (total) 36 Ketone Body Synthesis MCT1,2 Metabolites that feed into AcCoA are ketogenic. Frees up CoA pool in the liver needed to maintain ‐oxidation. Carries electrons and electron equivalents to peripheral tissues. Contribute to ketoacidosis (diabetic ketoacidosis) if unused. 37 The molecules 3-Hydroxy butyrate and acetoacetate are known as ketone bodies. They are an alternative way to move energy among organs during a prolonged fast. They are made only in the liver and burned instead of fat in most other tissues, including brain. As brain begins to use ketone bodies for fuel rather than glucose, the use of proteins to make glucose is spared, resulting in an increased survival time during starvation. After a 2-3 day fast, ketone body production is induced fully in the liver by rising levels of fatty acids and induction of the synthesis of the enzyme HMG-CoA synthase that is the rate-limiting enzyme in ketone body production. Ketone body synthesis occurs only in the liver. Two acetyl CoA molecules are condensed to make acetoacetyl-CoA and a third molecule of AcCoA is used to make 3-hydroxymethylglutaryl-Coenzyme A, a reaction catalyzed by HMG-CoA synthase (mitochondrial). HMG-CoA lyase then catalyzes the conversion of HMG-CoA to AcCoA and Acetoacetate. Most of the acetoacetate is reduced to 3-hydroxybutyrate and the acetoacetate and 3-HB are delivered into the blood. The ketone bodies then travel in the blood to be taken up in the tissues. There the hydroxybutyrate and acetoacetate are converted to AcAcCoA by an enzyme known as CoA transferase or thiophorase. The resulting AcAcCoA is then converted back to AcCoA and burned in the TCA cycle. The effect of ketone body synthesis in the liver is to increase the capacity for fat metabolism. As more and more AcCoA accumulate, the availability of CoASH to make more AcCoA is diminished. Ketone body synthesis in the liver and the export of the carbon skeletons into the blood frees up the CoASH pool and enables a higher capacity of fat metabolism. In effect, ketone body synthesis in the liver transfers some of the use of CoASH from the liver to the tissues, since the net result is to free up liver CoASH and utilize CoA in the tissues. Organs decide to use ketone bodies when they are available. As the concentrations of ketone bodies rise in the blood the transporter in the brain and other tissues has a high Km so that ketone body import rises as the serum concentration rise. There is some evidence that with time, there is an increase in the transporters MCT1 and MCT2 in brain with time that allows more entry of 3HB along with an increase in 3-hydroxybutyrate dehydrogenase, which is the slow enzyme in ketone body utilization in brain. In the blood, Acetoacetate spontaneously decarboxylates to form acetone. Acetone is a volatile compound and is exhaled through the lungs giving people who are making lots of ketone bodies a characteristic odor. In starvation as well as un- or under treated diabetes, or alcoholism, or a combination of these, ketone bodies accumulate in the blood and significantly decrease the pH leading to ketoacidosis, the consequences of which can be coma or death. Ketone fuels are metabolized by all aerobic tissues except liver. Acetoacetate is activated for oxidation by attachment of a CoA group that is transferred from succinyl- CoA, an intermediate in the citric acid cycle. There are two good reasons for switching to ketone fuels. Brain will adapt to using them to replace part of its glucose demands. In liver, the overall reaction, 2AcCoA + NADH + H+ 3-hydroxybutyrate + 2 CoASH frees up some CoASH so as to increase the capacity of the liver for AcCoA and fat metabolism. 37