Introduction to Molecular and Cellular Methods PDF
Document Details
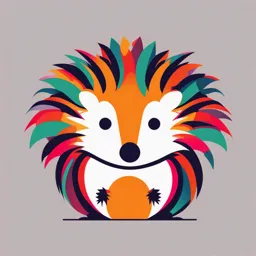
Uploaded by IntriguingCongas
Tags
Related
- Modulo 04 Fisiopatología General PDF
- Animal Models in Biomedical Research PDF
- UNIDAD 4 HIPERSENSIBILIDAD PDF
- Molecular and Cellular Methods in Biomedicine Lecture 1 PDF
- M1 TEMA 4. Gestión de Muestras Biológicas PDF
- CFGS Laboratorio Clínico y Biomédico - Gestión de Muestras Biológicas - Past Paper PDF
Summary
This document provides an introduction to molecular and cellular methods in biomedicine. It details the techniques and approaches used to study biological processes at the molecular and cellular levels, including examples like cancer diagnostics. The document also discusses advanced approaches like functional genomics.
Full Transcript
1. INTRODUCTION Dato: torsdag d. 7. sep. 2023 Topic: 1 Aim: To provide an overview of the course contents and general principles of experimental measurement. Learning outcomes - Recognize the relevance of molecular and cellular approaches in biomedicine - Revise the basics of measurement science - R...
1. INTRODUCTION Dato: torsdag d. 7. sep. 2023 Topic: 1 Aim: To provide an overview of the course contents and general principles of experimental measurement. Learning outcomes - Recognize the relevance of molecular and cellular approaches in biomedicine - Revise the basics of measurement science - Reflect about the importance of an appropriate experimental design Aim of the course: The goal of this module is to learn about modern methods used in a biomedical laboratory to investigate and diagnose disease processes, with a focus on methods used to study the diseases at molecular and cellular levels. MOLECULAR AND CELLULAR METHODS Molecular and cellular methods in biomedicine refer to the techniques and approaches used to study biological processes at the molecular and cellular levels. These methods play a crucial role in understanding the fundamental mechanisms of various diseases, developing new therapeutic strategies, and advancing medical research. Methods can be used to study a disease at a molecular and cellular level but there is a difference between cellular and molecular methods Example: methods for cancer diagnosis MOLECULAR METHODS 3 Focus: Molecular methods primarily focus on the study of molecules within cells, particularly nucleic acids (DNA and RNA) and proteins. Techniques: Techniques falling under molecular methods include - PCR (Polymerase Chain Reaction) - DNA sequencing - Gene expression analysis - Various methods for studying individual molecules such as DNA, RNA, and proteins. Applications: Molecular methods are often used to investigate genetic information, gene expression patterns, mutations, and various molecular processes occurring within cells. CELLULAR METHODS Focus: Cellular methods, on the other hand, are more concerned with the study of entire cells and their functions within tissues and organisms. Techniques: Cellular methods encompass a broader range of techniques, including - Cell culture - Microscopy (light microscopy, confocal microscopy) - Flow cytometry - Immunohistochemistry. These techniques allow researchers to observe and manipulate cells in their entirety. Applications: Cellular methods are often applied to investigate cell behavior, cellular interactions, responses to stimuli, and the overall structure and function of cells within tissues. ADVANCED DIAGNOSTICS: FUNCTIONAL GENOMICS Methods in biomedicine has become advanced and is becoming more advanced Advanced diagnostic techniques are being employed, and specifically, the focus is on utilizing functional genomics in these diagnostics. Functional genomics is the study of how genes, intergenic regions of the genome, proteins and metabolites work together to produce a particular phenotype. Functional genomics is being applied to enhance diagnostic methods. This could involve using genomic information to understand the underlying molecular mechanisms of diseases, identify specific genetic markers associated with certain conditions, and develop more targeted and personalized diagnostic approaches. The integration of functional genomics into advanced diagnostics signifies a more comprehensive and detailed analysis of genetic, molecular, and cellular factors to improve our understanding of diseases and enhance diagnostic capabilities. 4 RELEVANCE OF MOLECULAR AND CELLULAR METHODS IN BIOMEDICINE Molecular and cellular methods can be used to get a better understanding of the pathophysiology but it can also be used for diagnosis and to figure out what treatment the patient needs 5 THE GENERAL WORKFLOW The typical steps involved in conducting research or investigations using molecular and cellular methods in the field of biomedicine. 1. Problem definition In the context of molecular and cellular methods in biomedicine, problem definition involves identifying a specific biomedical question or issue that requires investigation. This could be related to understanding the molecular mechanisms of a disease, identifying biomarkers, exploring cellular responses to stimuli, or other biomedical research questions. Look at questions like: o What is the unknown? o What accuracy is required? o Is there a time (or money) limit? o How much sample is available? o What is the concentration range? o What components of the sample may cause an interference? o How many samples are to be analyzed? o Has anyone tried this before? 2. Method selection Once the problem is defined, researchers need to choose the appropriate molecular and cellular methods to address the specific aspects of the problem. This step involves selecting techniques such as PCR, DNA sequencing, cell culture, microscopy, or other relevant methods based on the nature of the biomedical question. Look at questions like: o What type of information is required? (quantitative or qualitative) o What type of samples are needed? (tissues, cells, biomolecules) o What is the amount of sample needed? o What will be the fate of the sample? (destructive or nondestructive test) o Does the technique provide a functional assessment? o Are there safety issues? 3. Measurement, data analysis and interpretation The selected methods are then employed to collect data or measurements. Following data collection, researchers engage in data analysis, using statistical or computational methods to make sense of the information. The interpretation of results involves understanding the biological implications of the data within the context of the biomedical problem being addressed. Look into these things: o Use of appropriate calibrations and controls o Careful record of the results o Identification of the variable to be studied and knowledge about other variables that need to be controlled o Replication of the measurements o Application of appropriate statistical tests (quantitative data) o Comparison with existing results/standards o Are there specific guidelines? 4. Report The final step is to communicate the findings and outcomes of the research. Researchers typically create a report that details the problem, the methods used, the results obtained, and the conclusions drawn. Remember: o Reports must be clear, concise, accurate, and fully interpretative. o Use tables/figures for complex datasets o When relevant, provide units, scale bars, information about statistical tests, normal ranges. o Follow guidelines when available 6 QUALITATIVE ASSAYS Qualitative assays refer to laboratory methods or tests that are designed to determine the presence or absence of a particular substance, analyte, or property in a sample without providing a quantitative measurement. These assays are primarily focused on providing a "yes" or "no"/ “positive” or “negative” answer rather than a precise numerical value. Here are some key characteristics of qualitative assays: Presence/absence determination Qualitative assays are used to identify the presence or absence of a specific substance, analyte, or property in a sample. They are particularly useful for screening purposes. Visual or binary results The results of qualitative assays are often visual or binary. For example, a color change, the appearance of a band on a test strip, or the presence of fluorescence may indicate the presence of the target substance. Rapid screening Qualitative assays are commonly used for rapid screening of samples. They provide a quick and simple way to determine whether a particular characteristic is present, making them suitable for point-of-care testing and initial assessments. Limitations Qualitative assays do not provide information about the quantity or concentration of the substance in the sample. If precise quantification is needed, quantitative assays are more appropriate. EXAMPLES OF QUALITATIVE ASSAYS Immunochromatographic tests (e.g., Pregnancy Tests): These tests use antibodies that produce visible lines or color changes when they bind to specific target molecules in a sample. Agarose gel electrophoresis (for DNA separation): It visually separates DNA fragments based on size, allowing researchers to determine the presence or absence of specific DNA fragments. Enzyme-linked Immunosorbent Assay (ELISA): While ELISA can be used for quantitative purposes, it can also be adapted for qualitative detection of specific proteins or antibodies. o Example: A test whose results are reported as either positive or negative, like a Qualitative ELISA for detection of antibodies in serum, yellow = positive and blue = negative 7 VALIDITY, SENSITIVITY AND SPECIFICITY In qualitative assays and methods regarding this, it's crucial to evaluate its validity. Validity encompasses both sensitivity and specificity to ensure that the test provides accurate and reliable information about the presence or absence of a particular medical condition. Valid tests contribute to more confident and informed medical decisions. Test validity Test validity refers to the degree to which a test accurately measures what it is intended to measure. In the context of medical diagnostics, it means assessing whether the test can correctly identify the presence or absence of a specific condition or disease. Sensitivity It is the ability of a diagnostic test to correctly identify individuals or samples who have the disease (true positives). In other words, sensitivity measures how well the test can detect true cases of the condition. A highly sensitive test minimizes false negatives, meaning it is effective at identifying individuals with the condition, reducing the likelihood of missing true positive cases. High sensitivity is crucial when a false negative result could have serious consequences, such as missing a true case of a severe medical condition. Specificity It is the ability of a diagnostic test to correctly identify individuals or samples who do not have the disease (true negatives). Specificity measures how well the test can exclude individuals who do not have the condition. A highly specific test is good at correctly identifying individuals without the disease when the test result is negative. High specificity is important in situations where a false positive result could lead to unnecessary interventions or treatments. Example For a diabetes diagnostic test, a sensitive test would correctly identify a high percentage of individuals with diabetes, minimizing the chances of false negatives. A specific test would correctly identify a high percentage of individuals without diabetes, minimizing the chances of false positives. High sensitivity is preferable because with low sensitivity we are not able to measure the small changes Example: Consider a diagnostic test for a specific biomarker associated with a disease. High sensitivity would mean that the test is effective at identifying individuals with even low levels of this biomarker, allowing for the detection of small changes that might occur early in the disease process. This allows for the detection of even subtle changes, supporting early intervention and improving the overall effectiveness of medical management. 8 QUANTITATIVE ANALYSIS Quantitative analysis refers to the process of determining the quantitative amount or concentration of a given substance or component (the analyte) in a sample, yielding specific numerical results in measurable units, such as grams and liters, or other relevant units depending on the nature of the substance being analyzed. Quantitative analysis is contrasted with qualitative analysis, which is concerned with identifying the presence or absence of substances without specifying the amount. EXAMPLES OF QUANTITATIVE ANALYSIS qPCR (Polymerase Chain Reaction): Quantifying the amount of DNA or RNA in a biological sample. Application: Gene expression studies, viral load measurements, and genetic testing. ELISA: Quantifying the concentration of specific proteins in a sample. Application: Detecting biomarkers, measuring cytokine levels, and assessing antibody responses. Flow Cytometry: Quantifying and analyzing characteristics of individual cells in a fluid suspension. Application: Cell sorting, immune cell profiling, and apoptosis detection. Magnetic Resonance Imaging (MRI) and Spectroscopy: Quantitative imaging of tissues to assess structure, function, and metabolite concentrations. Application: Diagnosis and monitoring of diseases, such as cancer and neurological disorders. Mass Spectrometry: Identifying and quantifying molecules based on their mass-to-charge ratios. Application: Proteomics, metabolomics, and analysis of biomolecules in biological samples. Positron Emission Tomography (PET): Quantitative imaging of radioactive tracers to visualize metabolic processes. Application: Cancer staging, brain function studies, and monitoring treatment responses. Western Blotting: Quantifying the expression levels of specific proteins in a sample. Application: Protein analysis, biomarker detection, and validation of gene expression studies. Microarray Analysis: Quantifying gene expression levels for thousands of genes simultaneously. Application: Studying gene regulation, identifying biomarkers, and understanding disease mechanisms. PRECISION AND ACCURACY Analytical methods can be characterized by a number of performance indicators: – Precision – Accuracy – Analytical specificity – Analytical sensitivity – Limit of detection Quantitative analysis aims for both precision (reproducibility of results) and accuracy (closeness of measurements to the true value). Precise measurements have low variability when repeated, while accurate measurements are close to the true or expected value. 9 Precision Precision in quantitative assays refers to the reproducibility and consistency of measurements when the same sample is analyzed multiple times. It assesses the degree of variability in repeated measurements. Refers to the reproducibility of analytical results. When a result is precise, numerical results agree closely. Precision can be estimated by repeating the measurement n times (when possible). Precision is usually expressed either as one standard deviation of the mean or as coefficient of variation of the mean o Example: measurements of fasting serum glucose from a patient. If a dataset has a mean of 2.42 mM units and a standard deviation of 0.165 mM, the Coefficient of Variation (CV) ".$%& () would be ! *.+* () , 𝑥 100 = 6.82%. This means that approximately 6.82% of the mean represents the relative variability within the dataset. § In practical terms, a lower CV is often associated with higher precision. It indicates that individual measurements are relatively close to the mean. § A higher CV suggests lower precision, with more variability among individual measurements. Accuracy Accuracy describes the correctness of a result by its closeness of measured values to an accepted, ‘true’, or reference value. It reflects the ability of the assay to provide results that agree with the actual quantity being measured. Accuracy is usually expressed in terms of confidence intervals, which provide the range of values where there is a given probability that the ‘true’ value lies o Example: If a researcher measures the concentration of a substance in a sample and reports an accuracy of 2.42 ± 0.20 mM with a 95% confidence interval, it means that there is a 95% probability that the true concentration lies within the range of the reported value 2.42 ± 0.20 mM. 10 Analytical specificity (also called selectivity) The extent to which a method can determine particular analytes under given conditions without interferences from other components. Analytical sensitivity This is a measure of the change in the response (output) to a defined change in the measured quantity (input) Limits of detection LLOD (lower) the lowest amount of analyte in the sample, which can be detected but not necessarily quantitated. Also known as minimal detection dose (MDD). ULOD (upper) the highest amount that can be detected before reaching saturation Limits of quantitation LLOQ (lower) and ULOQ (upper) the lowest and highest amounts of analyte that can be quantitatively determined with suitable precision and accuracy. Dynamic range The range over which quantitative measurements can be made Working range The range in which the measurements have the same level of accuracy and precision Robustness A measure of the ability to give consistent results in spite of small changes in experimental conditions such as temperature, pH, etc. 11 EXERCISES TOPIC 1 EXERCISE 1 In the article of Hafid et al, the authors compared three qualitative methods for the detection of Toxoplasma gondii infection in mice. The aim was to determine sensitivity and specificity of the methods at different timepoints post-infection 1A. WHAT WAS THE SENSITIVITY OF EACH METHOD AT THE DIFFERENT TIME POINTS ANALYZED? Sensitivity refers to the ability of a test to correctly identify individuals who have the disease. It is a measure of how well a test can detect true positive cases among those who are actually infected. Higher sensitivity indicates a test's ability to minimize false negatives, ensuring that individuals with the condition are correctly identified by the diagnostic method. In this case the sensitivity would be a measure of the ability to correctly identify mice that were infected with T. gondii at different time points for each method (PCR, capture ELISA, and immunoblotting) Which test was most sensitive? Time 3-15 hours 18 h 21 h 1-7 days Number of sera tested 25 5 5 35 Capture ELISA 0/25*100 = 0% 0/5*100 = 0% 0/5*100 = 0% 35/35*100 = 100% Immunoblotting 0/25*100 = 0% 0/5*100 = 0% 0/5*100 = 0% 35/35*100 = 100% PCR 0/25*100 = 0% 3/5*100 = 60% 5/5*100 = 100% 35/35*100 = 100% Conclusion PCR had an earlier and higher sensitivity compared to capture ELISA and immunoblotting in the experimental model of T. gondii infection in mice. PCR detected parasite DNA as early as 18 hours post-infection, while capture ELISA and immunoblotting were positive only at 24 hours or later. 1B. WHAT WAS THE SPECIFICITY OF EACH OF THE THREE METHODS? The specificity of a diagnostic test is the ability of the test to correctly identify individuals without the condition or disease as true negatives. 12 Control information: Sera from five uninfected mice were used as negative controls. Implication: If these control five mice were truly uninfected (true negative) and all diagnostic tests (capture ELISA, immunoblotting and PCR) yielded negative results for these control mice, it would indicate a high specificity for each method. Specificity calculation: Assuming that the control mice were indeed uninfected and all tests were negative for them, then: 𝑇𝑟𝑢𝑒 𝑛𝑒𝑔𝑎𝑡𝑖𝑣𝑒𝑠 𝑆𝑝𝑒𝑐𝑖𝑓𝑖𝑐𝑖𝑡𝑦 = × 100 𝑇𝑟𝑢𝑒 𝑛𝑒𝑎𝑡𝑖𝑣𝑒𝑠 + 𝐹𝑎𝑙𝑠𝑒 𝑝𝑜𝑠𝑖𝑡𝑖𝑣𝑒𝑠 In this case, false positives would be 0, and true negatives would be the number (5) of control mice. 𝑆𝑝𝑒𝑐𝑖𝑓𝑖𝑐𝑖𝑡𝑦 = 5 × 100 = 100% 5+0 That gives a specificity of 100% This implies that the tests were specific in correctly identifying non-infected mice. EXERCISE 2 In the article of Tassignon et al, the authors compare four different methods to detect T-cell mediated immune responses in human subjects. 2A. OUTLINE THE BASIC PRINCIPLE OF THE FOUR DIFFERENT METHODS Enzyme-linked immunosorbent assay (ELISA) Principle ELISA is a plate-based assay technique used for detecting and quantifying substances, in this case, IFN-gamma. It involves the use of antibodies and enzymes to measure the concentration of a specific antigen. Steps 1. Coating: A microplate is coated with an antigen (in this case, likely IFN-gamma) or an antibody specific to the cytokine of interest. The antigen or antibody is immobilized on the surface of the plate. 2. Blocking: Any remaining surface on the microplate is blocked to prevent nonspecific binding. Common blocking agents include bovine serum albumin (BSA) or non-fat milk. 3. Incubation with Samples: Samples containing the cytokine of interest are added to the plate. If the antigen is coated, the samples may contain antibodies against the cytokine. If an antibody is coated, the samples may contain the cytokine itself. 4. Binding: If the cytokine is present in the samples, it binds to the coated antibody or antigen on the plate, forming an immune complex. 5. Washing: The plate is washed to remove unbound substances. This step helps reduce background noise and ensures specificity. 6. Enzyme-Conjugated Antibodies: An enzyme-linked antibody specific to the cytokine is added. This secondary antibody binds to the cytokine if it is part of the immune complex. Common enzymes used are horseradish peroxidase (HRP) or alkaline phosphatase. 7. Substrate Addition: A substrate specific to the enzyme is added. The enzyme catalyzes a reaction with the substrate, producing a detectable signal. Common substrates include chromogenic substrates (yielding color changes) or chemiluminescent substrates (emitting light). 8. Signal Detection: The intensity of the signal is proportional to the amount of cytokine present in the sample. The reaction is stopped, and the absorbance or luminescence is measured using a spectrophotometer or a luminometer. 9. Data Analysis: The measured signal is compared to a standard curve generated using known concentrations of the cytokine. This allows for the quantification of the cytokine concentration in the original sample. Application in the Study In the study, ELISA was used to measure the bulk amount of IFN-gamma secreted in the culture supernatant. 13 EnzymeLinked Immunospot Assay (ELISPOT) Intra-Cellular Cytokine Detection (ICCD) Quantitative Real-Time Reverse Transcription Polymerase Chain Reaction (q-PCR) ELISPOT is a technique for detecting and quantifying the frequency of cytokinesecreting cells. It involves capturing the secreted cytokines on a solid support, forming spots that can be counted. Steps 1. Cell capture: Cells, usually peripheral blood mononuclear cells (PBMCs), are isolated from the sample of interest. These cells are then added to a microtiter plate that has been pre-coated with a capture antibody specific for the cytokine of interest. The plate is incubated to allow the cells to settle and adhere to the plate, with each well capturing the secretions of an individual cell. 2. Stimulation: Cells are stimulated with specific antigens or mitogens to induce cytokine production. In the context of the study you provided, the cells are likely stimulated with tetanus toxoid (TT) to elicit an immune response. 3. Cytokine Secretion: Activated cells secrete cytokines such as interferon-gamma (IFN-g) into the surrounding environment. 4. Detection: Unbound cells are removed by washing, leaving behind the captured cytokines on the plate. A detection antibody specific for the cytokine is added. This antibody is typically conjugated to an enzyme (e.g., alkaline phosphatase or horseradish peroxidase). 5. Enzyme Substrate Reaction: A substrate for the enzyme is then added. If the cytokine is present (indicating a cytokine-secreting cell), the enzyme catalyzes a reaction that produces a visible spot or "dot" at the location of the cytokinesecreting cell. 6. Spot Enumeration: The spots represent individual cells that have secreted the cytokine of interest. The spots can be counted, and the number of spots correlates with the frequency of cytokine-secreting cells in the original sample. ICCD involves the detection of cytokines within individual cells by using flow cytometry. Cells are stained with antibodies against specific cytokines, allowing the identification of cytokine-producing cell populations. Steps 1. Cell Stimulation: Peripheral blood mononuclear cells (PBMCs) or other cell populations are isolated from the sample. Cells are typically stimulated with specific antigens, mitogens, or other activating agents to induce cytokine production. In the study you provided, the cells may be stimulated with tetanus toxoid (TT) to trigger an immune response. 2. Cell Fixation and Permeabilization: Following stimulation, cells are fixed and permeabilized to allow antibodies to penetrate the cell membrane and access intracellular compartments. Fixation locks the cellular structures in place, and permeabilization allows antibodies to reach the cytokines inside the cells. 3. Antibody Staining: Fluorescently labeled antibodies specific to the cytokine of interest are added to the fixed and permeabilized cells. In the case of the study, antibodies against interferon-gamma (IFN-g) are likely used. 4. Flow Cytometry Analysis: The stained cells are passed through a flow cytometer, a specialized instrument that can detect and quantify fluorescent signals. As cells pass through the cytometer, lasers excite the fluorochromes attached to the antibodies, and detectors capture the emitted fluorescence. 5. Identification and Enumeration: The flow cytometer analyzes the fluorescent signals, allowing for the identification and enumeration of cells expressing the intracellular cytokine (e.g., IFN-g). Different subpopulations of cells, such as CD4+ T cells, CD8+ T cells, etc., can be distinguished based on additional surface marker staining. q-PCR is a molecular biology technique used to quantify the amount of specific RNA molecules in a sample. It involves reverse transcription of RNA into cDNA, followed by PCR amplification with real-time monitoring. Steps 1. RNA Extraction: Total RNA is extracted from the biological sample, often using specialized kits or methods that preserve the integrity of the RNA. 2. Reverse Transcription (cDNA Synthesis): The extracted RNA is reverse transcribed into complementary DNA (cDNA) using a reverse transcriptase enzyme. This step converts RNA into a stable DNA form, allowing for subsequent amplification. 3. Primer Design: Specific primers are designed to target the gene or RNA of interest. Primers are short DNA sequences that flank the region to be amplified. ELISPOT in this study enumerated the number of cells secreting IFN-gamma in response to the antigen. ICCD in this study focused on detecting intracellular IFN-gamma in CD4+ T cells, providing a more detailed analysis of the immune response. q-PCR in this study assessed the levels of IFNgamma mRNA, providing information about the transcriptional activity related to IFN-gamma. 14 One primer binds to the forward (sense) sequence, and the other primer binds to the reverse (antisense) sequence of the target gene. 4. Polymerase Chain Reaction (PCR): The q-PCR reaction mixture includes the cDNA template, primers, DNA polymerase, and fluorescent DNA-binding dyes or probes. The reaction goes through multiple cycles of denaturation, annealing, and extension, resulting in the exponential amplification of the target cDNA. 5. Fluorescent Detection: As the PCR progresses, the fluorescent signal increases proportionally to the amount of amplified DNA. The fluorescence can be monitored in real-time during each PCR cycle using a specialized instrument called a q-PCR machine. 6. Threshold Cycle (Ct): The cycle number at which the fluorescence signal crosses a defined threshold is called the threshold cycle (Ct). Ct values are inversely proportional to the initial amount of target RNA in the sample—the fewer cycles needed to reach the threshold, the higher the initial RNA concentration. 7. Standard Curve and Absolute Quantification: A standard curve is generated using known concentrations of a reference RNA or synthetic cDNA. The Ct values of the unknown samples are then compared to the standard curve to quantify the initial amount of the target RNA in the original sample. 8. Data Analysis: The q-PCR machine software analyzes the data, providing information on the expression level of the target gene in the sample. Normalization using internal reference genes helps correct for variations in RNA input and reverse transcription efficiency. 2B. CLASSIFY THE METHOD AS CELLULAR OR MOLECULAR. ARE THE METHODS QUANTITATIVE OR QUALITATIVE? Method ELISA Cellular/molecular ELISA is a molecular technique because it relies on the detection of molecules (usually proteins) using antibodies. ELISPOT ELISPOT is primarily cellular, as it detects individual cells secreting a particular cytokine. However, it also involves molecular components in the detection process. ICCD is primarily a cellular method as it assesses cytokine production within specific cell populations. ICCD q-PCR q-PCR is a molecular method that involves the amplification and quantification of nucleic acids (RNA in this case). Quantitative/qualitative ELISA is quantitative because it measures the concentration of a specific protein. This is achieved by comparing the signal from the sample to a standard curve generated from known concentrations. ELISPOT is quantitative because it counts the number of spots, representing individual cells producing a cytokine. ICCD is quantitative because it measures the percentage of cells within a population that produce a particular cytokine. q-PCR is quantitative, providing precise measurements of the amount of specific RNA in a sample. 2C. ANALYZE THE SENSITIVITIES OF THE METHODS FOR BOTH TIMEPOINTS (BEFORE AND AFTER BOOSTER VACCINATION). WHICH OF THE FOUR METHODS SHOULD BE PREFERABLY USED TO ACHIEVE OPTIMAL SENSITIVITY AND WHY? 15 Time Before booster vaccination After booster vaccination ELISA 57% ELISpot 93% ICCD 50% RT-qPCR 71% 43% 29% 79% 0% Before booster vaccination, ELISPOT demonstrated the highest sensitivity (93%), followed by q-PCR (71%), ELISA (57%), and ICCD (50%). After the booster vaccination at day +7, ICCD showed the highest sensitivity (79%), followed by ELISA (43%), ELISPOT (29%), and q-PCR (0%). Preference for optimal sensitivity: Before Booster Vaccination: ELISPOT showed the highest sensitivity, making it preferable for assessing the memory response before in vivo challenge with TT. After Booster Vaccination (Day +7): ICCD demonstrated the highest sensitivity, making it preferable for visualizing the vaccine effects at the cellular level after in vivo challenge with TT. Reasoning: ELISPOT is highly sensitive in detecting the memory response before vaccination, possibly due to its ability to capture low levels of cytokine secretion from individual cells. ICCD, which measures cell frequencies and captures the cellular response at a specific time point, showed high sensitivity after booster vaccination, making it suitable for assessing the cellular effects. In conclusion, the choice of the optimal method depends on the specific goal of the analysis— ELISPOT for memory response assessment before vaccination and ICCD for visualizing cellular effects after booster vaccination. EXERCISE 3 A study aims to compare two methods for detection for glucose in serum. The results of six measurements are given below: Measurements (in mmoL/L) Replicate number 1 2 3 4 5 6 Method A 2,5 2,6 2,3 2,3 2,4 2,5 Method B 2,8 3,0 3,1 2,4 2,1 2,2 3A. WHICH OF THE TWO METHODS IS MORE PRECISE? To determine which method is more precise, you can calculate the standard deviation for each method and compare them. The standard deviation is a measure of the dispersion of values in a set of data. A smaller standard deviation indicates greater precision. Method A Formula to use: 16 Σ(𝑥O − 𝑥̅ )* 𝑆K = L 𝑛−1 First calculate the mean (x̄ ) for method A: (2,5 + 2,6 + 2,3 + 2,3 + 2,4 + 2,5) 𝑚𝑚𝑜𝑙 𝑥̅ = = 2,433 6 𝐿 Calculate the standard deviation (SD) for method A: (2,5 − 2,433)* + (2,6 − 2,433)* + (2,3 − 2,433)* + (2,3 − 2,433)* + (2,4 − 2,433)* + (2,5 − 2,433)* 𝑆𝐷 = L 6−1 𝑆𝐷 = 0,1211 Find the coefficient of variation (CV): 𝐶𝑉 (%) = 𝐶𝑉 (%) = [ 𝑆𝐷 × 100 𝑥̅ 0,1211 \ × 100 = 4,9773% 2,433 Method B: Calculate the mean (x̄ ) for method B: (2,8 + 3,0 + 3,1 + 2,4 + 2,1 + 2,2) 𝑥̅ = = 2,6 𝑚𝑚𝑜𝑙/𝐿 6 Calculate the standard deviation (SD) for method B: (2,8 − 2,6)* + (3,0 − 2,6)* + (3,1 − 2,6)* + (2,4 − 2,6)* + (2,1 − 2,6)* + (2,2 − 2,6)* 𝑆𝐷 = L 6−1 𝑆𝐷 = 0,4242 Find the coefficient of variation (CV): 𝐶𝑉 (%) = 𝐶𝑉 (%) = [ 𝑆𝐷 × 100 𝑥̅ 0,4242 \ × 100 = 16,32% 2,6 Conclusion In terms of precision, Method A appears to be more precise because it has a smaller standard deviation (0,12), indicating less variability in the measured values. Method B, with a larger standard deviation (0,42), shows more variability among its measurements, suggesting lower precision compared to Method A. 17 3B. WHICH OF THE TWO METHODS IS MORE ACCURATE AT THE 95% INTERVAL? EXERCISE 4 A group of researchers has developed the “Albumin Tester”, a portable testing device for patients suffering hypertension. The device could be used to monitor the levels of albumin in urine, to help detecting early occurrence of kidney disease. As part of the assessment of the device’s performance, the researchers have measured the concentration of albumin in six replicates from a urine sample. A desktop device was used to perform control measurements. For both devices, six blank measurements were also performed using a saline solution. The results are summarized in the following table: Albumin tester Blank Urine sample Control instrument Blank Urine sample 1 Measurements (in µg/mL) Replicate number 2 3 4 5 6 0,5 205,1 0,4 213,2 0,2 191,4 0,3 196,4 0,4 210,9 0,5 206,3 0,2 199,1 0,2 202,2 0,1 198,8 0,3 202,3 0,3 202,2 0,1 198,3 Based on the results of these measurements, calculate and compare the instruments in terms of: 4A. THE LOWER LIMIT OF DETECTION (LLOD) AND THE LOWER LIMIT OF QUANTIFICATION (LLOQ) Lower Limit of Detection (LLOD): The LLOD is the lowest concentration of an analyte that can be reliably detected but not necessarily quantified. It is typically calculated as three times the standard deviation of the blank divided by the slope of the calibration curve. Lower Limit of Quantification (LLOQ): The LLOQ is the lowest concentration of an analyte that can be 18 reliably quantified with acceptable precision and accuracy. It is often calculated as ten times the standard deviation of the blank divided by the slope of the calibration curve. For the Albumin tester: LLOD Calculate the mean (x̄ ) of blank: 𝑥̅ = 0,5 + 0,4 + 0,2 + 0,3 + 0,4 + 0,5 6 = 0,383 Calculate the standard deviation (SD): (2,8 − 2,6)* + (3,0 − 2,6)* + (3,1 − 2,6)* + (2,4 − 2,6)* + (2,1 − 2,6)* + (2,2 − 2,6)* 𝑆𝐷 = L 6−1 𝑆𝐷 = 0,117 𝑳𝑳𝑶𝑫 = 𝟎, 𝟑𝟖𝟑 + 𝟑 ∗ 𝟎, 𝟏𝟏𝟕 = 𝟎, 𝟕𝟑𝟒 LLOQ: Calculate the mean (x̄ ) of blank: 𝑥̅ = 0,5 + 0,4 + 0,2 + 0,3 + 0,4 + 0,5 6 = 0,383 Calculate the standard deviation (SD): (2,8 − 2,6)* + (3,0 − 2,6)* + (3,1 − 2,6)* + (2,4 − 2,6)* + (2,1 − 2,6)* + (2,2 − 2,6)* 𝑆𝐷 = L 6−1 𝑆𝐷 = 0,117 𝑳𝑳𝑶𝑸 = 𝟎, 𝟑𝟖𝟑 + 𝟏𝟎 ∗ 𝟎, 𝟏𝟏𝟕 = 𝟏, 𝟓𝟓𝟑 For the control instrument LLOD: Calculate the mean (x̄ ) of blank: 𝑥̅ = 0,2 + 0,2 + 0,1 + 0,3 + 0,3 + 0,1 6 = 0,2 Calculate the standard deviation (SD): (0,2 − 0,2)* + (0,2 − 0,2)* + (0,1 − 0,2)* + (0,3 − 0,2)* + (0,3 − 0,2)* + (0,1 − 0,2)* 𝑆𝐷 = L 6−1 𝑆𝐷 = 0,0894 𝑳𝑳𝑶𝑫 = 𝟎, 𝟐 + 𝟑 ∗ 𝟎, 𝟎𝟖𝟗𝟒 = 𝟎, 𝟒𝟔𝟖𝟐 19 LLOQ: Calculate the mean (x̄ ) of blank: 𝑥̅ = 0,2 + 0,2 + 0,1 + 0,3 + 0,3 + 0,1 6 = 0,2 Calculate the standard deviation (SD): (0,2 − 0,2)* + (0,2 − 0,2)* + (0,1 − 0,2)* + (0,3 − 0,2)* + (0,3 − 0,2)* + (0,1 − 0,2)* 𝑆𝐷 = L 6−1 𝑆𝐷 = 0,0894 𝑳𝑳𝑶𝑸 = 𝟎, 𝟐 + 𝟏𝟎 ∗ 𝟎, 𝟎𝟖𝟗𝟒 = 𝟏, 𝟎𝟗𝟒𝟎 4B. PRECISION For the Albumin tester: First calculate the mean (x̄ ) for urine sample: (205,1 + 213,2 + 191,4 + 196,4 + 210,9 + 206,3) 𝑥̅ = = 203,883 6 Calculate the standard deviation (SD): 𝑆𝐷 = L (205,1 − 203,883)* + (213,2 − 203,883)* + (191,4 − 203,883)* + (196,4 − 203,883)* + (210,9 − 203,883)* + (206,3 − 203,883)* 6−1 𝑆𝐷 = 8,428 Find the coefficient of variation (CV): 𝐶𝑉 (%) = [ 8,428 \ × 100 = 4,13% 203,883 For the control instrument First calculate the mean (x̄ ) for urine sample: (199,1 + 202,2 + 198,8 + 202,3 + 202,2 + 198,3) 𝑥̅ = = 200,483 6 Calculate the standard deviation (SD): 𝑆𝐷 = L (199,1 − 200,483)* + (202,2 − 200,483)* + (198,8 − 200,483)* + (202,3 − 200,483)* + (202,2 − 200,483)* + (198,3 − 200,483)* 6−1 𝑆𝐷 = 1,934 Find the coefficient of variation (CV): 𝐶𝑉 (%) = [ 1,934 \ × 100 = 0,9646% 200,483 20 4C. ACCURACY AT THE 95% INTERVAL For the Albumin tester: Degrees of freedom = n-1 Degree of freedom = 6-1 = 5 Look at Student’s t values for the desired confidence interval For 95% confidence interval is t = 2,571 𝐶𝑜𝑛𝑓𝑖𝑑𝑒𝑛𝑐𝑒 𝑖𝑛𝑡𝑒𝑟𝑣𝑎𝑙 = 𝑥̅ ± 𝐶𝑜𝑛𝑓𝑖𝑑𝑒𝑛𝑐𝑒 𝑖𝑛𝑡𝑒𝑟𝑣𝑎𝑙 = 203,883 ± 𝑡 ∗ 𝑆𝐷 √𝑛 2,571 ∗ 8,428 √6 𝑪𝒐𝒏𝒇𝒊𝒅𝒆𝒏𝒄𝒆 𝒊𝒏𝒕𝒆𝒓𝒗𝒂𝒍 = 𝟐𝟎𝟑, 𝟖𝟖𝟑 ± 𝟖, 𝟖𝟒𝟔 For the control instrument Degree of freedom = 6-1 = 5 For 95% confidence interval is t = 2,571 𝐶𝑜𝑛𝑓𝑖𝑑𝑒𝑛𝑐𝑒 𝑖𝑛𝑡𝑒𝑟𝑣𝑎𝑙 = 𝑥̅ ± 𝐶𝑜𝑛𝑓𝑖𝑑𝑒𝑛𝑐𝑒 𝑖𝑛𝑡𝑒𝑟𝑣𝑎𝑙 = 200,483 ± 𝑡 ∗ 𝑆𝐷 √𝑛 2,571 ∗ 1,934 √6 𝑪𝒐𝒏𝒇𝒊𝒅𝒆𝒏𝒄𝒆 𝒊𝒏𝒕𝒆𝒓𝒗𝒂𝒍 = 𝟐𝟎𝟎, 𝟒𝟖𝟑 ± 𝟐, 𝟎𝟐𝟗𝟗 EXERCISE 5 Read the application note from the NanoVue spectrophotometer. 5A. WHAT ARE THE WORKING RANGES OF THE INSTRUMENT FOR EACH OF THE PROTEIN ASSAY METHODS? 21 5B. WHAT COULD BE THE REASON WHY THE NANOVUE HAS A SMALLER LLOQ THAN THE NANODROP IN THE BIURET ASSAY? NanoVue may have a higher sensitivity to changes in absorbance at lower concentrations of proteins. This increased sensitivity allows for accurate measurements at concentrations lower than those achievable by NanoDrop. 5C. HOW IS THE ANALYTICAL SENSITIVITY OF THE NANOVUE AS COMPARED TO THE NANODROP INSTRUMENT? PROVIDE AN ANSWER FOR EACH OF THE FIVE ASSAYS REPORTED IN THE DOCUMENT. The analytical sensitivity is closely tied to how steep or flat the curves are on concentration-absorbance graphs. Steeper curves indicate higher sensitivity, allowing for precise measurements at lower concentrations. o Low analytical sensitivity = flat curves o High analytical sensitivity = steep curves Graphs in spectrophotometry: in spectrophotometry, a graph typically represents the relationship between the concentration of a substance (analyte) and the measured absorbance. This relationship is often depicted as a curve on a graph. Steepness of curves: the steepness or slope of the curve on the graph indicates how rapidly the absorbance changes with respect to changes in concentration. Steeper curves suggest a more rapid increase in absorbance for each incremental change in concentration. Analytical sensitivity: analytical sensitivity refers to the ability of the instrument to detect and respond to changes in concentration. A more sensitive instrument can effectively capture small variations in absorbance, especially at lower concentrations. o High analytical sensitivity: a spectrophotometer with a steeper curve is considered to have high analytical sensitivity. This means that even small changes in concentration result in a noticeable and significant change in absorbance. It can accurately measure low concentrations of analytes with precision. o Low analytical sensitivity: a flatter curve indicates lower analytical sensitivity. In this case, larger changes in concentration are needed to produce noticeable differences in absorbance. Such instruments may be less effective at quantifying lower concentrations with precision. Bradford NanoVue and NanoDrop are very close to each other - not a significant difference in sensitivity 22 Micro BCA NanoVue is slightly steeper = a bit more analytical sensitivity Lowry NanoVue has a steeper slope (going up to approximately 2.5, while NanoDrop only goes up to approximately 1) - Thus, higher sensitivity with NanoVue. 23 Biuret assay NanoVue is slightly steeper = a bit more analytical sensitivity Direct UV measurement NanoVue is slightly steeper = a bit more analytical sensitivity 24 EXERCISE 6 A researcher wants to evaluate the performance of a new microliter-based spectrophotometer in comparison to the well-established NanoDrop from Thermo Scientific. To do this, the researcher performs measurements on a set of diluted protein standards using a Bradford assay. After performing absorbance measurements at 595 nm, the following average values are obtained: The following figure is the graphical representation of the data: The researcher performs some calculations to determine the lower and upper limits of quantification. The lower limits of quantification (LLOQ) were 50 µg/mL for the NanoDrop and 125 µg/mL for the new instrument, while the upper limits were the same for both instruments (1500 µg/mL) 6A. FOR BOTH INSTRUMENTS, INDICATE THE WORKING RANGES OF PROTEIN CONCENTRATION THAT THEY CAN MEASURE USING THE BRADFORD ASSAY The working range is defined as from the lower limits of quantification (LLOQ) to the upper limits of detection (ULOQ) The working range for Nanodrop is 50 µg/mL to 1500 µg/mL The working range for the new instrument is 125 µg/mL to 1500 µg/mL 25 6B. USING THE REGRESSION EQUATIONS IN THE GRAPH, ANALYZE AND COMPARE THE ANALYTICAL SENSITIVITY OF THE INSTRUMENTS The analytical sensitivity is the coefficient of the slope. For Nanodrop the analytical sensitivity is 0.0004 while the analytical sensitivity for the new instrument is 0.0002. That means that Nanodrop is more sensitive than the new instrument because it can both detect a smaller sample and a more precise result, than the new instrument. 6C. IF THE PRICE OF THE NEW INSTRUMENT IS COMPARABLE TO THE NANODROP, WHICH INSTRUMENT WOULD YOU RECOMMEND THE RESEARCHER PURCHASE? JUSTIFY YOUR ANSWER If the price is comparable to NanoDrop, then they should keep the NanoDrop because its more sensitive and with a larger working range than the new instrument. The new instrument would have to be a lot cheaper to compensate for the poorer performance to make any argument for buying that over NanoDrop. EXERCISE 7 A researcher would like to compare the performance of a new microliter-based spectrophotometer against the well-established NanoDrop from Thermo Scientific. For that purpose, a BCA protein quantification assay was set up. The following measurements were obtained after performing a series of measurements from known albumin standards: 7A. FOR EACH DATASET PLOT THE STANDARD CURVES AND PERFORM A LINEAR REGRESSION USING A SPREADSHEET PROGRAM (E.G. MS EXCEL) 7B. ACCORDING TO THE STANDARD CURVE, WHAT IS THE ANALYTICAL SENSITIVITY OF THE NEW INSTRUMENT? IS THE ANALYTICAL SENSITIVITY OF THE NEW INSTRUMENT BETTER OR WORSE, AS COMPARED TO THAT OF THE NANODROP? 7C. OUTLINE WHICH PROCEDURE WOULD YOU FOLLOW TO QUANTIFY THE LOWER LIMIT OF DETECTION (LLOD) OF THE INSTRUMENTS. 26