Introduction to Muscles and Nerves PDF
Document Details
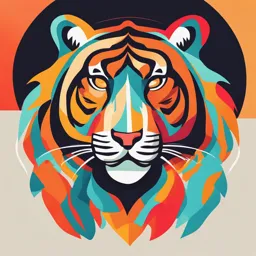
Uploaded by CohesiveBandura2383
Merit University
Tags
Summary
This document provides an introduction to the human physiology of different muscle types including skeletal, cardiac, and smooth muscles. It covers muscle function, anatomy, and properties.
Full Transcript
Introduction to Physiology of the muscles& Nerve I- MUSCLES Muscles are the effector which enable movement to be carried out. Classification of muscles: 1. Skeletal muscles 2. Smooth muscles 3. Cardiac muscles A- Skeletal (striated) muscles T...
Introduction to Physiology of the muscles& Nerve I- MUSCLES Muscles are the effector which enable movement to be carried out. Classification of muscles: 1. Skeletal muscles 2. Smooth muscles 3. Cardiac muscles A- Skeletal (striated) muscles They form most of the muscles. Called Skeletal because attached to skeleton. Called Striated because show striations under microscope. Voluntary Eg. Muscles of back, limbs, etc. Page 1 of 38 Attachment of skeletal muscles Skeletal muscles usually have two attachments’: 1. Origin: proximal end fixed or less mobile. 2. Insertion: distal end more mobile. Attachments may be via: 1. Fleshy fibers. 2. Tendon- round, fibrous cord. 3. Aponeurosis- flat fibrous sheet. 4. Raphe – interdigitating tendinous ends. Skeletal Muscle Tissue Microscopy: Each skeletal muscle fiber is long, cylindrical and multi- nucleated. The nuclei are situated peripherally and immediately below the sarcolemma Each fiber shows cross-striations. Voluntary movement Page 2 of 38 Connective Tissue Coverings of Muscle Endomysium: Loose connective layer covering each muscle fiber. Each group called a fascicle. Perimysium. Dense connective layer surrounding a group of muscle fibers. Epimysium. Connective tissue layer that surrounds a whole muscle (many fascicles). Smooth Muscle Tissue Page 3 of 38 B- Smooth muscle Characteristics: Smooth muscle fibers are Spindle-shaped cells with a central ovoid nucleus in each fiber Arranged close to each other with only minimal connective tissue and fibroblasts in between. No striations Involuntary control Location: Mostly walls of hollow organs. Page 4 of 38 C-Cardiac Muscle Tissue Characteristics: Cross striations similar to skeletal muscle. Contain single central nucleus Cardiac muscle fibers show branching Dark staining structures called Intercalated discs are present. (Specialized junctional complexes between adjacent cardiac muscle fibers). Location: Occurs in walls of heart MUSCLES-STRUCTURE AND FUNCTION FUNCTIONS AND PROPERTIES OF MUSCLE TISSUE Functions of muscle tissue 1. Movement: Our body's skeleton gives enough rigidity to our body that skeletal muscles can yank and pull on it, resulting in body movements such as walking, chewing, running, lifting, manipulating objects with our hands, and picking our noses. 2. Maintenance of posture: Without much conscious control, our muscles generate a constant contractile force that allows us to maintain an erect or seated position, or posture. 3. Respiration: Our muscular system automatically drives movement of air into and out of our body. Page 5 of 38 4. Heat generation: Contraction of muscle tissue generates heat, which is essential for maintenance of temperature homeostasis. For instance, if our core body temperature falls, we shiver to generate more heat. 5. Communication: Muscle tissue allows us to talk, gesture, write, and convey our emotional state by doing such things as smiling or frowning. 6. Constriction of organs and blood vessels: Nutrients move through our digestive tract, urine is passed out of the body, and secretions are propelled out of glands by contraction of smooth muscle. Constriction or relaxation of blood vessels regulates blood pressure and blood distribution throughout the body. 7. Pumping blood: Blood moves through the blood vessels because our heart tirelessly receives blood and delivers it to all body tissues and organs. 8. This isn't a complete list. Among the many possible examples are the facts that muscles help protect fragile internal organs by enclosing them, and are also critical in maintaining the integrity of body cavities. For example, fetuses with incompletely formed diaphragms have abdominal contents herniate (protrude) up into the thoracic cavity, which inhibits normal lung growth and development. Even though this is an incomplete list, an appreciation of some of these basic muscle functions will help you as we proceed. Properties of muscle tissue All muscle cells share several properties: contractility, excitability, extensibility, and elasticity: 1. Contractility is the ability of muscle cells to forcefully shorten. For instance, in order to flex (decrease the angle of a joint) your elbow you need to contract (shorten) the biceps brachii and other elbow flexor muscles in the anterior arm. Notice that in order to extend your elbow, the posterior arm extensor muscles need to contract. Thus, muscles can only pull, never push. 2. Excitability is the ability to respond to a stimulus, which may be delivered from a motor neuron or a hormone. 3. Extensibility is the ability of a muscle to be stretched. For instance, let's reconsider our elbow flexing motion we discussed earlier. In order to be able to flex the elbow, the elbow extensor muscles must extend in order to allow flexion to occur. Lack of extensibility is known as spasticity. 4. Elasticity is the ability to recoil or bounce back to the muscle's original length after being stretched. Page 6 of 38 Skeletal Muscle Cells-Gross and Microscopic Structure Each skeletal muscle cell, also called a muscle fiber, develops as many embryonic myocytes fused into one long, multi-nucleated skeletal muscle cell. These muscle fibers are bound together into bundles, or fascicles, and are supplied with a rich network of blood vessels and nerves. The fascicles are then bundled together to form the intact muscle. Let's dissect a skeletal muscle, beginning with the muscle as a whole externally, and continuing internally down to the submicroscopic level of a single muscle cell. Connective tissue covering: The epimysium ("epi"-outside, and "mysium"-muscle) is a layer of dense fibrous connective tissue that surrounds the entire muscle. This layer is also often referred to as the fascia. Each skeletal muscle is formed from several bundled fascicles of skeletal muscle fibers, and each fascicle is surrounded by perimysium ("peri"-around). Each single muscle cell is wrapped individually with a fine layer of loose (areolar) connective tissue called endomysium ("endo"-inside). These connective tissue layers are continuous with each other and they all extend beyond the ends of the muscle fibers themselves, forming the tendons that anchor muscles to bone, moving the bones when the muscles contract. Deep to the endomysium, each skeletal muscle cell is surrounded by a cell membrane known as the sarcolemma (You will see the prefixes sarc- and myo-quite a bit in this discussion, so you should understand that these are prefixes that refer to "muscle"). The cytoplasm, or sarcoplasm contains a large amount of glycogen (the storage form of glucose) for energy, and myoglobin-a red pigment similar to hemoglobin that can store oxygen. Most of the intracellular space, however, is taken up by rod-like myofibrils-cylindrical protein structures. Each muscle fiber contains hundreds or even thousands of myofibrils that extend from one end of each muscle fiber to the other. These myofibrils take up about 80% of the intracellular space, and are so densely packed inside these cells that mitochondria and other organelles get sandwiched between them while the nuclei get pushed to the outside and are located on the periphery right under the sarcolemma. Page 7 of 38 Each myofibril is comprised of several varieties of protein molecules that form the myofilaments, and each myofilament contains the contractile segments that allow contraction. These contractile segments are known as sarcomeres ("sarc-" - muscle, "mere" - part). The striations seen microscopically within skeletal muscle fibers are formed by the regular, organized arrangement of myofilaments-much like what we would see if we painted stripes on chopsticks and bundled them together with plastic wrap, with the plastic representing the sarcolemma. The striations microscopically visible in skeletal muscle are formed by the regular arrangement of proteins inside the cells. Notice that there are light and dark striations in each cell. The dark areas are called A bands, which is fairly easy to remember because "a" is the second letter in "dark." The light areas are called I bands, and are also easy to remember because "i" is the second letter in "light." ("A" actually stands for anisotropic, and "I" stands for isotropic. Both of these terms refer to the light absorbing character of each band. However, we'll stick to A and I bands.) The image below shows a micrograph of a sarcomere along with a drawing representing the different parts of the sarcomere. Notice that in the middle of each I band is a darker line called the z line or z disc. The Z lines are the divisions between the adjacent sarcomeres. Sarcomeres are connected end to end along the entire length of the myofibril. Also, in the middle of each A band is a lighter H zone (H for "helle"-"bright"), and each H zone has a darker M line (M for "middle") running right down the middle of the A band. Page 8 of 38 Page 9 of 38 Each myofibril, in turn, contains several varieties of protein molecules, called myofilaments. The larger, or thick myofilaments are made of the protein, myosin, and the smaller thin myofilaments are chiefly made of the protein, actin. Let's discuss each myofilament in turn. Each actin molecule is composed of two strands of fibrous actin (F actin) and a series of troponin and tropomyosin molecules. Each F actin is formed by two strings of globular actin (G actin) wound together in a double helical structure, much like twisting two strands of pearls with each other. Each G actin molecule would be represented by a pearl on our hypothetical necklace. Each G actin subunit has a binding site for the myosin head to attach to the actin. Tropomyosin is a long string-like polypeptide that parallels each F actin strand and functions to either hide or expose the "active sites" on each globular actin molecule. Each tropomyosin molecule is long enough to cover the active binding sites on seven G-actin molecules. These proteins run end-to-end the entire length of the F actin. Associated with each tropomyosin molecule is a third polypeptide complex known as troponin. Troponin complexes contain three globular polypeptides (Troponin I, Troponin T, and Troponin C) that have distinct functions. Troponin I binds to actin, Troponin T binds to tropomyosin and helps position it on the F actin strands, and Troponin C binds calcium ions.There is one troponin complex for each tropomyosin. When calcium binds to Troponin C, it causes a conformational change in the entire complex that results in exposure of the myosin binding sites on the G actin subunits. More on this later. The thick myofilaments are composed chiefly of the protein myosin, and each thick myofilament is composed of about 300 myosin molecules bound together. Each myosin is made up of 6 proteins subunits, 2 heavy chains and 4 light chains. The heavy chains have a shape similar to a golf club, having a long shaft-like structure, to which is connected the globular myosin head. The shafts, or tails wrap around each other and interact with the tails of other myosin molecules, forming the shaft of the thick filament. The globular heads project out at right angles to the shaft. Half of the myosin molecules have their heads oriented toward one end of the thick filament, and the other half are oriented in the opposite direction. It is the myosin heads that bind to the active sites on the actin. The connection between the head and the shaft of the myosin molecules functions as a hinge, and as such is referred to as the hinge region. The hinge region can bend, and as we shall see later, creates the power stroke when the muscle contracts. The center of the thick filaments are composed only of the shaft portions of the heavy chains. Additionally, each myosin head has an Page 10 of 38 ATPase that binds to and hydrolyses ATP during muscle contraction. It is the ATP that provides the energy for muscle contraction. Each of the myosin heads is associated with two myosin light chains that play a role in regulating the actions of the myosin heads, but the exact mechanism is not fully understood. The three dimensional arrangement of the myosin heads is very important. Imagine that you were looking at a thick filament from the end, and that there is a myosin head sticking straight up. As you moved around the circumference of the thick filament, you would see myosin heads every 30 degrees. This allows each thick filament to interact with 6 thin filaments. Likewise, each thin filament can interact with three thick filaments. This arrangement requires that there be two thin filaments for every thick filament in the myofibril (see image below). During muscle contraction, the myosin heads link the thick and thin myofilaments together, forming cross bridges that cause the thick and thin myofilaments to slide over each other, resulting in shortening of each sarcomere, each skeletal muscle fiber, and the muscle as a whole-much like the two parts of an extension ladder slide over each other. To summarize, in order Page 11 of 38 for the shortening of the muscle to occur, the myosin heads have three important properties: 1. The heads can bind to active sites on G-actin molecules, forming cross bridges. 2. The heads are attached to the rod-like portions of the heavy myosin molecules by a hinge region as already discussed, and 3. The heads have ATPase enzymes that can break down ATP, using the resulting energy to bend the hinge region and allowing detachment of the myosin heads from actin. The Z-line (or Z-disc) is composed of proteins (alpha actinin) which provide an attachment site for the thin filaments. Likewise, the M-line is composed of proteins (myomesin) that hold myosin molecules in place. The A band is formed by myosin molecules, and the I band is the location where thin filaments do not overlap the thick filaments. The H zone is that portion of the A band where the thick and thin filaments do not overlap. There is another important structural protein that extends from the Z disc to the M line, running within the thick filament. Due to its large size, this protein is called titin (titin is the largest known protein in the human body and has roughly 30,000 amino acids). It forms the core of the thick myofilaments, holding it in place, and thus keeping the A band organized. In addition, titin has the ability to stretch and recoil and functions to prevent overstretching and damage to the muscle as well as to return it to its normal length when the muscle is stretched beyond its normal resting length. Recall that one of the properties of muscle is its elasticity, titin is the protein responsible for this property. There are several other important structural proteins, but we will only discuss one more: dystrophin. Dystrophin is a protein located between the sarcolemma and the outermost myofilaments. It links actin to an integral membrane protein, which in turn links the muscle cell to the endomysium of the entire muscle fiber. Genetic mutation of the gene coding for dystrophin is one of the root causes of a class of muscle diseases known collectively as muscular dystrophy (MD). The most common form of MD is Duchene muscular dystrophy (DMD), which is inherted in a "sex-linked" fashion and affects boys. Most DMD patients become wheelchair bound early in life, usually by age 12 or so. Page 12 of 38 Difficulty breathing usually become problematic by age 20, and is often the cause of their sadly premature death. Sarcoplasmic reticulum and T tubules Page 13 of 38 There are two sets of tubules within skeletal muscles fibers that carry out critical functions during muscle contractions: the sarcoplasmic reticulum and the T tubules. T tubules (transverse tubules) are invaginations, or indentations of the sarcolemma. They are formed much like a young picky-eater poking holes in his mashed potatoes. T tubules communicate with the extracellular space, and are filled with extracellular fluid. They are located on the sarcomere at the points that the A band and I band overlap. The T tubules are flanked on either side by dilated regions of the cell's endoplasmic reticulum-the sarcoplasmic reticulum. Sarcoplasmic reticulum (SR) is an elaborate network of smooth endoplasmic reticulum that surrounds and encases each myofibril, much like a loosely knitted sweater covers your arms. It stores calcium which can then be released into the sarcoplasm when an action potential is conducted along the sarcolemma of the T tubule. Most of the sarcoplasmic reticulum runs parallel to the myofibrils, but there are right-angle enlargements of the SR at the A band-I band junctions that flank the T tubules. These enlargements are known as terminal cisternae ("end sacs") (see the image above). One T tubule along the two terminal cisternae that parallel it form the triad. The triad is critical in skeletal muscle function. At each triad, the T tubule membrane contains large numbers of voltage-dependant proteins called dihydropyridine (DHP) channels or L-type Calcium channels. Although these are called channels, they do not allow calcium to move through them; rather, they are physically linked to calcium release channels on the terminal cisternae known as Ryanodine receptor channels (RyR). When the membrane is depolarized by an action Page 14 of 38 potential, the DHP channel detects a depolarization and causes the RyR channels to open, resulting in the release of calcium from the terminal cisternae of the SR. Sliding Filament Model of Muscle Contraction A similar, although less dramatic, connection occurs in skeletal muscle physiology. In order for these muscle fibers to contract, there needs to be an electrical event-an action potential that is followed by a mechanical event- the contraction of the muscle fiber. Because you have already learned about the resting membrane potential and the action potential, we'll move past that and talk about the sliding filament model of muscle contraction. Recall that we have already mentioned the fact that the thick and thin myofilaments slide over each other, like the parts of an extension ladder. The proteins themselves don't shorten. The muscle contraction and shortening occurs as the myofilaments grip each other, slide past each other, and shorten the sarcomeres. Thus, this is known as the sliding filament model of muscle contraction. Let's also remember that in order for action potentials to both start and propagate (travel), it is necessary for various ion channels to open and close at just the right time. Some of these ion channels open in response to binding of a ligan-an atom or molecule that binds to a receptor and stimulates a specific response. These types of ion channels are known as ligand- gated ion channels. Other ion channels open in response to a change in voltage- electricity, and are known as voltage-gated ion channels. Now we'll discuss the sequence of events that occur when and action potential reaches the end of the motor neuron. 1. An action potential arrives at the axon terminal of a somatic motor neuron. Remember that in skeletal muscle, no stimulation by a motor neuron means no contraction. (This is not necessarily true for smooth and cardiac muscle, but we'll get to that later.) The axon terminal of the motor neuron connects to the muscle fiber via the neuromuscular junction (a synapse). 2. The arrival of the action potential stimulates Voltage- 2+ 2+ gated Ca channels in the axon's membrane to open, and Ca enters the axon terminal from the extracellular space. 3. The axon terminal contains synaptic vesicles filled with the neurotransmitter acetylcholine (ACh). The increased Ca2+ levels is the signal that stimulates exocytosis of these synaptic vesicles and the release of ACh into the synaptic cleft. Page 15 of 38 4. The ACh diffuses across the synaptic cleft, binding to acetylcholine receptors on the ligand-gated ion channels (nicotinic type I) in the sarcolemma of the post synaptic tissue, the muscle fiber. This specialized region of the sarcolemma is known as the motor end plate, and this is the location of the ACh receptors. 5. ACh binding causes ligand-gated Na+/K+ channels to open. These ion channels are permeable to both Na+ and K+ , however, more Na+ diffuses into the cell than K+ diffuses out of the cell. This may seem odd since both would be moving down their concentration gradients. The difference has to do with the charge on the membrane. Since the inside of the cell is negative, which will attract Na+ , Na+ will be moving down both its concentration and its electrical gradients. Potassium, on the other hand, would move down its concentration gradient but against its electrical gradient (the negative charge inside the cell will attract the K+. The Na+ entering the cell depolarizes the sarcolemma which then will cause the voltage-gated Na+ channels to open, initiating an action potential that spreads out from the neuromuscular junction. The action potential not only travels across the sarcolemma, but also down the T tubules. Remember, T tubules are just invaginations (inward protrusions) of the sarcolemma, and so are filled with extracellular fluid-high in sodium (Na+) and low in potassium (K+). Also please notice that the ACh receptors are ligand- gated, but movement of Na+ through them causes voltage gated Na+ channels to open, resulting in generation and propagation of an action potential. a. While the action potential spreads, let's take a break and describe how the stimulation of the ACh receptors is terminated. For the muscle to relax ACh must be removed from the synaptic cleft. This is done when ACh is cleaved (split) by an enzyme that resides in the cleft called acetylcholinesterase. This enzyme splits ACH into its two components, acetate (acetyl) and choline, rendering it nonfunctional. The acetate portion of acetylcholine diffuses out of the synaptic cleft. The choline, which is an essential nutrient in the Vitamin B group ( B 4 ), is taken up by the axon terminal, where it is recycled to make more acetylcholine. Although our bodies can make choline, we cannot produce enough for our needs and must get it in our diet and recycle what we have. 6. The action potential does its thing (If you have forgotten the basics of action potentials review module 5). 7. As the action potential spreads along the sarcolemma and the T-tubules, the resultant change in potential causes other voltage gated channels in the T- tubule to respond. These channels are called dihydropyridine channels (DHP) or L-type Ca2+ channels, and are mechanically linked to ryanodine receptor channels (RyR) , calcium channels located in the sarcoplamsic reticulum membrane. These two protein channels span the distance between the T-tubule and the terminal cisternae of the sarcoplasmic reticulum. In response to the change in membrane potential, the DHP channel causes the RyR to open and allows Ca2+ ions to leave the sarcoplasmic reticulum and diffuse into the Page 16 of 38 sarcoplasm. These calcium ions bind to troponin causing it to move the tropomyosin molecules off of the active sites on each G-actin molecule. 8. Uncovering the active sites allows the mysoin heads to bind to actin binding sites, forming cross bridges. In the resting state, the myosin head is "cocked" and ready to go. It also has ADP and phosphate (Pi) attached to it. The binding to actin causes Pi to detach from the myosin head resulting in the release of energy which causes the myosin head to bend (see the image below). This bending or power stroke forcefully pulls the actin past the myosin. During the power stroke the ADP is also released from they myosin. Recall the arrangement of the thick filaments with half of the myosin molecules pointing one way and half pointing the other. Since the myosin heads on the opposite ends of the thick filaments all pull towards the middle, the overall effect is to cause the sarcomere to shorten. As all of the sarcomeres in the muscle fiber shorten, the entire muscle shortens or contracts. 9. In order for significant shortening of a skeletal muscle fiber to occur, the myosin heads must now detach from the G-actin active sites and then re-attach to a different active site further along the neighboring actin molecule. This is rather like the fact that in order to climb a ladder we must pull ourselves up a rung, and then let go and move our hands and feet to higher rungs. In order for this release to occur, each myosin head must bind an ATP molecule. The binding of ATP to the myosin head allows it to release from the actin. The ATPase then hydrolyzes the ATP into ADP and a phosphate group which causes the head to "recock" (the recovery stroke),preparing it for the next power stroke. Hence, binding ATP allows the head to release and hydrolysis of ATP re-energizes the head for the next power stroke. During a single muscle cell contraction, each myosin molecule undergoes the entire cross-bridge cycle many times-a process known as cross-bridge cycling. As long as Ca2+ is present and the active sites are exposed, the process will continue. One other important concept: Using the analogy above, when we climb a ladder, we don't take both hands off of the rungs at the same time. Likewise, when muscles contract, the myosin heads are cycling asynchronously, meaning that they don't all bind actin at the same time, and they don't all release at the same time. At any given time, the 300 or so myosin heads in one thick filament will be at different stages of the cross-bridge cycle. The movement of myosin heads occurs in two phases: 1. The power stroke or working stroke occurs when the myosin heads bend and ratchet the actin molecules past the myosin, and 2. The recovery stroke involves the myosin heads detaching from actin and being cocked back into the high energy position to prepare for the next power stroke. Page 17 of 38 Page 18 of 38 Relaxation occurs when the release of acetylcholine ceases at the neuromuscular junction. The acetylcholine already in the synapse is broken down by acetylcholinesterase, ending action potential generation and propagation. This stops Ca2+ release from the sarcoplasmic reticulum (SR). Ca2+ ions diffuse away from troponin as the Ca2+ is actively transported back into the SR. This allows the troponin-tropomyosin complex to resume its resting position, blocking the active binding sites on the individual G-actin molecules. This prevents cross bridges from reforming and results in muscle relaxation. To quickly review: the sliding filament model of muscle contraction explains the fact that when skeletal muscle fibers contract, the individual proteins (actin and myosin) don't shorten. Rather, they slide over each other. ATP is necessary for detachment of myosin heads from actin. Notice also that when a sarcomere contracts, both the H zone and the light I band shrink in width, while the dark A band doesn't appear to narrow. Page 19 of 38 Muscle contractures and cramps Muscle contracture is a term that generally refers to a muscle that has shortened and resists relaxing to its normal resting length. There are many possible causes of a contracture. Some of the reasons that tend to cause long term or even permanent contracture include prolonged immobilization, spasticity, and muscle weakness. Although rare, temporary contractures can occur with severe muscle fatigue resulting in a condition called physiologic contracture. Extreme exercise may lead to a relative depletion of ATP. The lack of ATP prevents the detachment of the myosin head from the actin, preventing the muscle from relaxing. Recall that in order for the myosin head to detach from the actin, ATP must bind to the myosin. Without ATP, the cross bridge remains intact and the muscle becomes rigid. Though not considered a "contracture" in the normal sense of the word, rigor mortis is muscle rigidity also caused by a depletion of ATP. Rigor mortis is associated with death. It may take hours to fully develop, but cellular death results in the breakdown of intracellular sarcoplasmic reticulum and the leakage of Ca2+. The Ca2+ then initiates the events that allow cross bridge formation. However, cell death also results in the cessation of ATP production and without ATP to cause the dissociation of myosin heads from actin, the muscle stays in a contracted position and cannot relax or be stretched. Students often ask us what causes muscle cramps. It appears that there are many things that contribute, and physiologists are still puzzling this out. Below is some information that is mostly FYI that reveals some of the current thinking and theory about muscle cramps: Another interesting phenomenon of uncontrolled muscle contraction is a cramp. Most people have experienced or will experience a sudden, involuntary, painful contraction at some point. It is very common during or after intense exercise. A characteristic that distinguishes cramps from contractures is that contractures are "electrically silent." This means that we do not see repeated action potentials coming down the motor neurons to the muscle cell. Contractures originate because of a physiological change of the muscle fiber itself and not the motor neuron that innervates it. Cramps, on the other hand are associated with repeated firing of action potentials in the motor neurons. Cramps are found most commonly in muscles of the leg, especially the lower leg and foot. The pathophysiology of cramps is poorly understood. It is suspected that the origin of a cramp may be either central or peripheral. The central theories suggest that there are some changes in the brain or spinal cord that cause an abnormal Page 20 of 38 frequency of action potentials to the lower motor neurons that will innervate skeletal muscle. Peripheral theories suggest that there is some change around peripheral motor neurons that cause them to discharge spontaneously. Functional use of muscles is a more complicated process than most of us realize. The brain and spinal cord receive afferent (sensory) information that helps us know how and where our body is moving in space. Then, our brain and spinal cord respond by inhibiting some muscle fibers and contracting others. The brain and spinal cord receive a lot of information really fast during intense exercise and action potential patterns are tweaked and enhanced to keep us going and make our movements most successful. The sudden stopping of an intense activity might leave us with action potential delivery mechanisms intended for muscles and movements that are no longer occurring. Perhaps a cramp is a manifestation of the inability of the nervous system to resolve this conflict. Peripherally, there is significant redistribution of blood flow as the body deals with energy needs and heat buildup. In what is somewhat of a paradox, when a skeletal muscle is contracting blood delivery to the muscle decreases. This is due to the fact that a contracting muscle constricts blood vessels limiting blood flow in that vessel. Over time, during intense exercise, a muscle may contract long and hard enough that blood supply is disrupted in key areas such as the epineurium of motor nerve bundles. Blood flow disruption may be enough to cause slight imbalances in the concentrations of key extracellular ions in localized areas. Exercise also results in water loss through sweat and dehydration and can certainly influence extra cellular ion concentrations as well. There is plenty of anecdotal evidence to suggest that sports drinks balanced with electrolytes can decrease the occurrence of exercise induced cramping. While most athletes will swear that electrolyte enhanced drinks help them, it is difficult to point to an electrolyte imbalance as the ultimate cause of muscle cramps. Maughan (1986), selected a group of marathon runners who experienced cramps during a race and compared their blood tests to those who did not have cramps. There were no significant differences in serum electrolyte levels for the two groups of runners. Maughan's conclusion was that if electrolyte imbalance was the problem it was not likely systemic. Recently, the BBC news outlet reported on a sudden death that occurred in a 23 year old male running a marathon in Hove England. The autopsy reported that sports supplements high in potassium coupled with dehydration contributed to the fatality. Page 21 of 38 Intense exercise is often the precipitator of a muscle cramp, but exercise is not a necessary requirement to experience a cramp. Many people report cramping at rest or even at night while they are asleep. The theories that suggest that exercise is the cause of cramping are hard to adapt to a cramp that has nothing to do with intense exercise. The mechanisms of cramps at night or at rest are even a bigger puzzle than the ones occurring with exercise. One common theory has been to blame muscle cramps on Ca2+. People who demonstrate hypocalcemia (low blood Ca2+ levels) generally experience frequent muscle cramps. It has already been illustrated that intracellular Ca2+ is key to muscle contraction, but muscles also depend on the concentration of extracellular Ca2+ to help stabilize the voltage-gated Na + channels. If the extracellular Ca2+ concentration declines then the Na + channels become hypersensitive and have a tendency to "pop" open independent of any outside stimulus. This can lead to uncontrollable cramping. There are several conditions that can cause calcium levels to drop. Thyroid and parathyroid problems, kidney problems, severe dietary deficiencies, pregnancy, and very low vitamin D are a few. It is not likely, however, that generally healthy people will have hypocalcemia during exercise or at rest. The suggestion to drink milk to resolve cramps for most healthy people experiencing them is not likely to be helpful. We hear all kinds of folklore advice. "Eat a banana, drink milk, drink water, ingest baking soda, etc." Research on the internet on how to stop muscle cramps is like looking up ways to stop the hiccups; everyone has a solution. The take home message on muscle cramps is this: 1. There are likely many many different causes and we really don't know much about them or why they occur. 2. We need to be very careful to not get caught up in sports drinks, powders, potions, and plans that are sold to us to increase our exercise ability or decrease our pain (like cramps). Remember the guy mentioned above who died doing this (he was only 23!). 3. It is pretty normal to get muscle cramps as they are quite common. However, if cramps are getting worse, lasting longer, and coming more often over a period of time, you should seek medical advice as there are several pathologies that have muscle cramps as one of the defining symptoms. Page 22 of 38 MOTOR UNITS The motor neurons that innervate skeletal muscle fibers are called alpha motor neurons. As the alpha motor neuron enters a muscle, it divides into several branches, each innervating a muscle fiber (note this in the image above). One alpha motor neuron along with all of the muscle fibers it innervates is a motor unit. The size of the motor unit correlates with the function of the muscle. In muscles involved with fine, coordinated control, the motor units are very small with 3-5 muscle fibers per motor neuron. Muscles that control eye movement and muscles in our hands have relatively small motor units. On the other hand in muscles involved with more powerful but less coordinated actions, like the muscles of the legs and back, the motor units are large with 1000s of muscle fibers per motor neuron. Page 23 of 38 MUSCLE TWITCH When an action potential travels down the motor neuron, it will result in a contraction of all of the muscle fibers associated with that motor neuron. The contraction generated by a single action potential is called a muscle twitch. A single muscle twitch has three components. The latent period, or lag phase, the contraction phase, and the relaxation phase. The latent period is a short delay (1-2 msec) from the time when the action potential reaches the muscle until tension can be observed in the muscle. This is the time required for calcium to diffuse out of the SR, bind to troponin, the movement of tropomyosin off of the active sites, form ation of cross bridges, and taking up any slack that may be in the muscle. The contraction phase is when the muscle is generating tension and is associated with cycling of the cross bridges, and the relaxation phase is the time for the muscle to return to its normal length. The length of the twitch varies between different muscle types and could be as short as 10 ms (milliseconds) or as long as 100 ms (more on this later). If a muscle twitch is just a single quick contraction followed immediately by relaxation, how do we explain the smooth continued movement of our muscles when they contract and move bones through a large range of motion? The answer lies in the ordering of the firing of the motor units. If all of the motor units fired simultaneously the entire muscle would quickly contract and relax, Page 24 of 38 producing a very jerky movement. Instead, when a muscle contracts, motor units fire asynchronously, that is, one contracts and then a fraction of a second later another contracts before the first has time to relax and then another fires and so on. So, instead of a quick, jerky movement the whole muscle contraction is very smooth and controlled. Even when a muscle is at rest, there is random firing of motor units. This random firing is responsible for what is known as muscle tone. So, a muscle is never "completely" relaxed, even when asleep. However, if the neuron to a muscle is cut, there will be no "muscle tone" and this is called flaccid paralysis. There are several benefits of muscle tone: First it takes up the "slack" in the muscle so that when it is asked to contract, it can immediately begin to generate tension and move the limb. If you have ever towed a car you know what happens if you don't take the slack out of the tow rope before starting to pull. The second thing muscle tone does is deter muscle atrophy. TYPES OF MUSCLE CONTRACTION Muscle contractions are described based on two variables: force (tension) and length (shortening). When the tension in a muscle increases without a corresponding change in length, the contraction is called an isometric contraction (iso = same, metric=length). Isometric contractions are important in maintaining posture or stabilizing a joint. On the other hand, if the muscle length changes while muscle tension remains relatively constant, then the contraction is called an isotonic contraction (tonic = tension). Furthermore, isotonic contractions can be classified based on how the length changes. If the muscle generates tension and the entire muscle shortens than it is a concentric contraction. An example would be curling a weight from your waist to your shoulder; the bicep muscle used for this motion would undergo a concentric contraction. In contrast, when lowering the weight from the shoulder to the waist the bicep would also be generating force but the muscle would be lengthening, this is an eccentric contraction. Eccentric contractions work to decelerate the movement at the joint. Additionally, eccentric contractions can generate more force than concentric contractions. Think about the large box you take down form the top shelf of your closet. You can lower it under total control using eccentric contractions but when you try to return it to the shelf using concentric contractions you cannot generate enough force to lift it back up. Strength training, involving both concentric and eccentric contractions, appears to increase muscle strength more than just concentric contractions alone. Page 25 of 38 However, eccentric contractions cause more damage (tearing) to the muscle resulting in greater muscle soreness. If you have ever run downhill in a long race and then experienced the soreness in your quadriceps muscles the next day, you know what we are talking about. Muscle size is determined by the number and size of the myofibrils, which in turn is determined by the amount of myofilament proteins. Thus, resistance training will induce a cascade of events that result in the production of more proteins. Often this is initiated by small, micro-tears in and around the the muscle fibers. If the tearing occurs at the myofibril level the muscle will respond by increasing the amount of proteins, thus strengthening and enlarging the muscle, a phenomenon called hypertrophy. This tearing is thought to account for the muscle soreness we experience after a workout. As mentioned above, the repair of these small tears results in enlargement of the muscle fibers but it also results in an increase in the amount of connective tissue in the muscle. When a person "bulks up" from weight training, a significant percent of the increase in size of the muscle is due to increases in the amount of connective tissue. It should be pointed out that endurance training does not result in a significant increase in muscle size but increases its ability to produce ATP aerobically. FACTORS THAT INFLUENCE THE FORCE OF MUSCLE CONTRACTION Obviously our muscles are capable of generating differing levels of force during whole muscle contraction. Some actions require much more force generation than others; think of picking up a pencil compared to picking up a bucket of water. The question becomes, how can different levels of force be generated? Multiple-motor unit summation or recruitment: It was mentioned earlier that all of the motor units in a muscle usually don't fire at the same time. One way to increase the amount of force generated is to increase the number of motor units that are firing at a given time. We say that more motor units are being recruited. The greater the load we are trying to move the more motor units that are activated. However, even when generating the maximum force possible, we are only able to use about 1/3 of our total motor units at one time. Normally they will fire asynchronously in an effort to generate maximum force and prevent the muscles from becoming fatigued. As fibers begin to fatigue they are replaced by others in order to maintain the force. There are times, Page 26 of 38 however, when under extreme circumstances we are able to recruit even more motor units. You have heard stories of mothers lifting cars off of their children, this may not be totally fiction. Watch the following clip to see how amazing the human body can be. Muscle recruitment. (Video Transcription Available) Wave summation: Recall that a muscle twitch can last up to 100 ms and that an action potential lasts only 1-2 ms. Also, with the muscle twitch, there is not refractory period so it can be re-stimulated at any time. If you were to stimulate a single motor unit with progressively higher frequencies of action potentials you would observe a gradual increase in the force generated by that muscle. This phenomenon is called wave summation. Eventually the frequency of action potentials would be so high that there would be no time for the muscle to relax between the successive stimuli and it would remain totally contracted, a condition called tetanus. Essentially, with the high frequency of action potentials there isn't time to remove calcium from the cytosol. Maximal force, then, is generated with maximum recruitment and an action potential frequency sufficient to result in tetanus. Page 27 of 38 Initial Sarcomere Length: It has been demonstrated experimentally that the starting length of the sarcomere influences the amount of force the muscle can generate. This observation has to do with the overlap of the thick and thin filaments. If the starting sarcomere length is very short, the thick filaments will already be pushing up against the Z-disc and there is no possibility for further sarcomere shortening, and the muscle will be unable to generate as much force. On the other hand, if the muscle is stretched to the point where myosin heads can no longer contact the actin, then again, less force will be generated. Maximum force is generated when the muscle is stretched to the point that allows every myosin head to contact the actin and the sarcomere has the maximum distance to shorten. In other words, the thick filaments are at the very ends of the thin filaments. These data were generated experimentally using frog muscles that were dissected out and stretched between two rods. Intact muscles in our bodies are not normally stretched very far beyond their optimal length due to the arrangement of muscle attachments and joints. Page 28 of 38 However, you can do a little experiment that will help you see how force is lost when a muscle is in a very short or a very stretched position. This experiment will use the muscles that help you pinch the pad of your thumb to the pads of your fingers. These muscles are near maximal stretch when you extend your arm and also extend your wrist. As your wrist is cocked back into maximal extension, try to pinch your thumb to your fingers. See how weak it feels? Now, gradually flex your wrist back to a straight or neutral position. You should feel your pinch get stronger. Now, flex your elbow and your wrist. With your wrist in maximal flexion, the muscles you use to pinch with are near their most shortened position. Try pinching again. It should feel weak. But, again, as you extend your wrist back to neutral you should feel your pinch get stronger. ENERGY SOURCE FOR MUSCLE CONTRACTION The ultimate source of energy for muscle contraction is ATP. Recall that each cycle of a myosin head requires an ATP molecule. Multiply that by all of the myosin heads in a muscle and the number of cycles each head completes each twitch and you can start to see how much ATP is needed for muscle function. It is estimated that we burn approximately our entire body weight in ATP each day so it becomes apparent that we need to constantly replenish this important energy source. For muscle contraction, there are four ways that our muscles get the ATP required for contraction. 1. Cytosolic ATP: This ATP represents the "floating" pool of ATP, or that which is present and available in the cytoplasm. This ATP requires no oxygen (anaerobic) to make it (because it is already there) and is immediately available but it is short lived. It provides enough energy for a few seconds of maximal activity in the muscle-not the best source for long term contraction. Nevertheless, for the muscles of the eyes that are constantly contracting quickly but for short periods of time, this is a great source. 2. Creatine Phosphate: Once the cytosolic stores of ATP are depleted, the cell calls upon another rapid energy source, Creatine Phosphate. Creatine phosphate is a high energy compound that can rapidly transfer its phosphate to a molecule of ADP to quickly replenish ATP without the use of oxygen. This transfer requires the enzyme creatine kinase, an enzyme that is located on the M-line of the sarcomere. Creatine phosphate can replenish the ATP pool several times, enough to extend muscle contraction up to about 10 seconds. Creatine Phosphate is the most widely used supplement by weight lifters. Although some benefits have been demonstrated, most are very small and limited to highly selective activities. Page 29 of 38 3. Glycolysis: Glycolysis, as the name implies, is the breakdown of glucose. The primary source of glucose for this process is from glycogen that is stored in the muscle. Glycolysis can function in the absence of oxygen and as such, is the major source of ATP production during anaerobic activity. This series of chemical reactions will be a major focus in the next unit. Although glycolysis is very quick and can supply energy for intensive muscular activity, it can only be sustained for about a minute before the muscles begin to fatigue. 4. Aerobic or Oxidative Respiration: The mechanisms listed above can supply ATP for maybe a little over a minute before fatigue sets in. Obviously, we engage in muscle activity that lasts much longer than a minute (things like walking or jogging or riding a bicycle). These activities require a constant supply of ATP. When continuous supplies of ATP are required, the cells employ metabolic mechanisms housed in the mitochondria that utilize oxygen. We normally refer to these processes as aerobic metabolism or oxidative metabolism. Using these aerobic processes, the mitochondria can supply sufficient ATP to power the muscle cells for hours. The down side of aerobic metabolism is that it is slower than anaerobic mechanisms and is not fast enough for intense activity. However, for moderate levels of activity, it works great. Although glucose can also be utilized in aerobic metabolism, the nutrient of choice is fatty acids. As described below, slow-twitch and fast-twitch oxidative fibers are capable of utilizing aerobic metabolism FATIGUE When we think of skeletal muscles getting tired, we often use the word fatigue, however, the physiological causes of fatigue vary considerably. At the simplest level, fatigue is used to describe a condition in which the muscle is no longer able to contract optimally. To make discussion easier, we will divide fatigue into two broad categories: Central fatigue and peripheral fatigue. Central fatigue describes the uncomfortable feelings that come from being tired, it is often called "psychological fatigue." It has been suggested that central fatigue arises from factors released by the muscle during exercise that signal the brain to "feel" tired. Psychological fatigue precedes peripheral fatigue and occurs well before the muscle fiber can no longer contract. One of the outcomes of training is to learn how to overcome psychological fatigue. As we train we learn that those feelings are not so bad and that we can continue to perform even when it feels uncomfortable. For this reason, elite athletes hire trainers that push them and force them to move past the psychological fatigue. Peripheral fatigue can occur anywhere between the neuromuscular junction and the contractile elements of the muscle. It can be divided into two Page 30 of 38 subcategories, low frequency (marathon running) and high frequency (circuit training) fatigue. High frequency fatigue results from impaired membrane excitability as a result of imbalances of ions. Potential causes are inadequate functioning of the Na+/K+ pump, subsequent inactivation of Na+ channels and impairment of Ca2+ channels. Muscles can recover quickly, usually within 30 minutes or less, following high frequency fatigue. Low frequency fatigue is correlated with impaired Ca2+ release, probably due to excitation coupling contraction problems. It is much more difficult to recover from low frequency fatigue, taking from 24 hours to 72 hours. In addition, there are many other potential fatigue contributors, these include: accumulation of inorganic phosphates, hydrogen ion accumulation and subsequent pH change, glycogen depletion, and imbalances in K+. Please note that factors that are not on the list are ATP and lactic acid, both of which do not contribute to fatigue. The reality is we still don't know exactly what causes fatigue and much research is currently devoted to this topic. SKELETAL MUSCLE FIBER TYPES Classically, skeletal muscle fibers can be categorized according to their speed of contraction and their resistance to fatigue. These classifications are in the process of being revised, but the basic types include: 1. Slow twitch oxidative (type I) muscle fibers, 2. Fast-twitch oxidative-glycolytic (Type IIA) muscle fibers, and 3. Fast-twitch glycolytic (Type IIX) fibers. Fast-twitch (type II) fibers develop tension two to three times faster than slow- twitch (type I) fibers. How fast a fiber can contract is related to how long it takes for completion of the cross-bridge cycle. This variability is due to different varieties of myosin molecules and how quickly they can hydrolyze ATP. Recall that it is the myosin head that splits ATP. Fast-twitch fibers have a more rapid ATPase (splitting of ATP into ADP + Pi) ability. Fast-twitch fibers also pump Ca2+ ions back into the sarcoplasmic reticulum very quickly, so these cells have much faster twitches than the slower variety. Thus, fast-twitch fibers can complete multiple contractions much more rapidly than slow-twitch fibers. For a complete list of how muscle fibers differ in their ability to resist fatigue see the table below: Page 31 of 38 Slow Twitch Oxidative Fast-twitch Oxidative (Type Fast-Twitch Glycolytic (Type I) IIA) (Type IIX) Myosin ATPase activity slow fast fast Size (diameter) small medium large Duration of contraction long short short SERCA pump activity slow fast fast Fatigue resistant resistant easily fatigued Energy utilization aerobic/oxidative both anerobic/glycolytic capillary density high medium low mitochondria high numbers medium numbers low numbers Color red (contain red (contain myoglobin) white (no myoglobin) myoglobin) In human skeletal muscles, the ratio of the various fiber types differs from muscle to muscle. For example the gastrocnemius muscle of the calf contains about half slow and half fast type fibers, while the deeper calf muscle, the soleus, is predominantly slow twitch. On the other hand the eye muscles are predominantly fast twitch. As a result, the gastrocnemius muscle is used in sprinting while the soleus muscle is important for standing. In addition, women seem to have a higher ratio of slow twitch to fast twitch compared to men. The "preferred" fiber type for sprinting athletes is the fast-twitch glycolytic, which is very fast, however, most humans have a very low percentage of these fibers, < 1%. Muscle biopsies of one world class sprinter revealed 72% fast twitch fibers and amazingly 20% were type IIX. The Holy Grail of muscle research is to determine how to change skeletal muscle fibers from one type to another. It appears that muscle fiber types are determined embryologically by the type of neuron that innervates the muscle fiber. The default muscle appears to be slow, type I fibers. If a muscle is innervated by a small neuron that muscle fiber will remain slow, whereas large mylenated fibers induce the fast isoforms. In addition, the frequency of firing rates of the neuron also alters the muscle fiber type. Research suggests that humans have subtypes of fibers, making up about