Instructional Materials in Veterinary Anatomy PDF
Document Details
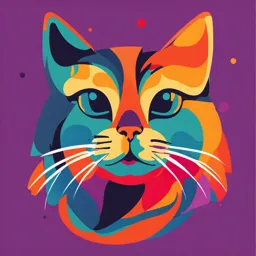
Uploaded by FeatureRichMarigold
2010
Hazel Marie R. Boloron
Tags
Related
Summary
This document is instructional material in veterinary anatomy, focusing on developmental anatomy. It covers embryology, including gametogenesis in various species, and the significance of developmental anatomy in understanding gross anatomy and congenital malformations. The text includes definitions of key terms and a detailed discussion of chemical components and characteristics of spermatozoa and semen from different animals.
Full Transcript
Instructional Materials in VETERINARY ANATOMY 53 (Developmental Anatomy) by HAZEL MARIE R. BOLORON, DVM, MS 2010 Developmental Anatomy In...
Instructional Materials in VETERINARY ANATOMY 53 (Developmental Anatomy) by HAZEL MARIE R. BOLORON, DVM, MS 2010 Developmental Anatomy Instructional Materials in Developmental Anatomy by: HAZEL MARIER BOLORON Embryology is the study of the physiological development and growth of antenatal organism. SIGNIFICANCE 1. Provide a dynamic perspective on gross anatomy by presenting a historical view of tissue and organ relationship. 2. Aids in the understanding of congenital malformations. HISTORICAL BACKGROUND Theories 1. Preformation theory - believed that all tissues and the general body form were present in the sperm or egg, and that development proceeded by enlargement of this miniature organism. 17th century - microscopist Halsmelier claimed to have observed a miniature human (homunculus) in the head of a sperm. 2. Epigenetic theory - proposed that the tissues and form of an organism arose in a sequential manner from the fertilized egg. 1828 - von Baer proposed that all vertebrate embryos pass through a stage at which they are anatomically very similar. This stage is seen in the dog of about 18 days of gestation, 24 days of gestation in the cow, and 48-60 hours of incubation in the chick embryo. DEFINITION OF TERMS Epithelium - a tissue which lines the surface of an organ, i.e., epidermis of skin (derived from ectoderm) lining of digestive tract (derived from endoderm) lining of kidney tubules which lines blood vessels mesothelium lines body cavities (eg. peritoneal cavity) Mesenchyme - embryonic precursor of all organs except the CNS. Connective tissue - tissue which binds other tissues of the body. Ectoderm - outermost layer of cells. It covers the whole body except at the site of emergence of the umbilical stalk. Mesoderm - middle layer of a more loosely arrayed cells which forms most of the muscle and skeletal tissues, the urogenital system, the heart and the blood vessels. Endoderm - innermost layer of cells which forms the lining of the digestive tract, respiratory system, and of those organs associated with digestion. Neural tube - hollow tube located in the dorsal midline beneath the ectoderm. Primitive gut - hollow tube which runs the length of the embryo near the ventral midline. It is derived from endoderm Pharynx - rostral end of the primitive gut. It presents a series of lateral outpocketings called the pharyngeal pouches that extend out to the surface ectoderm Paraxial mesoderm - segmented mesoderm located beneath the neural tube and on either side of the notochord. Each segment is called a somite. The somites give rise to the axial skeleton and voluntary muscles Notochord - a longitudinal rod immediately ventral to the neural tube that extends from the level of the midbrain to the tail. It also indicates the future location of the vertebral column. Intermediate mesoderm - portion of mesoderm located lateral to the paraxial mesoderm which consists of epithelial ducts and tubules that will form nephrogenic and gonadal structures Lateral mesoderm - mesoderm which extends around the gut and beneath the surface ectoderm to the ventral midline It is divided into outer somatic (parietal) and inner splanchnic layers with a cavity, the coelom between them. These structures are found throughout the embryonic body except in the head and tail regions where the coelom is absent. Heart - initially seen as a slightly curved tube beneath the caudal part of the pharynx. Extending rostrally from the heart is a single vessel, the ventral aorta from which bilateral pairs of vessels called aortic arches arise. 1 Developmental Anatomy All vertebrate embryos share the following common features: 1. Neural tube 2. Somites 3. Primitive gut 4. Coelom 5. Heart and Aortic arches Prenatal Stages 1. Period of the ovum - from fertilization up to 10-12 days in cow 2. Period of the embryo - development which covers artil the individual attains the form of the adult - 12-45 days in cow 3. Period of the fetus - covers the remainder of the prenatal period until birth - 45 days up to parturition STAGES OF DEVELOPMENT 1. GAMETOGENESIS - The process of development and maturation of sex cells called the gametes. The gametes arise from primordial germ cells outside of the embryonic body in the yolk sac tissue. These cells migrate to and enter the embryonic gonad where they proliferate and eventually develop and mature. Oogenesis Primordial Germ Cell: Oogonium - undergo mitosis up to birth and enter prophase of meiosis Primary oocyte - undergo synapsis and each replicate to form two chromatids Exchange of genetic material occurs Completion of the first meiotic division does not take place until after sexual maturity Secondary oocyte – cell division is unequal (the other smaller cell is the first polar body which will only degenerates Undergo second maturation division (meiosis) Ovum - retains most of the cytoplasm (the other cell becomes a second polar body) Spermatogenesis Primordial Germ Cell Spermatogonium – undergo mitosis during the entire life of the animal – replicates its DNA Primary spermatocyte – undergo first meiotic (reduction) division Secondary spermatocyte – immediately undergo second division Spermatids – undergo maturation or spermiogenesis No cell division but modifying those structures that are retained to form a highly specialized cell geared for motility and penetration. As a result, the following changes take place: Spermatid Spermatozoa Golgi apparatus becomes Acrosome (head cap) Nucleus becomes Head Centrioles becomes Tail (flagellum) Gametes - mature reproductive cells of individuals capable of fertilization. The structure and physiology of gametes but must accommodates three functions: 1. To be able to survive in environments quite different from that of a gonad. 2. To be able to recognize homologous cells of the other gender and participate in events related to fertilization. 3. To provide sufficient genetic and depending upon the species, cytoplasmic materials to support development of a new organism. Ova are classified on the basis of size which is related to the amount and distribution of the yolk (lecithin) as follows: Animal Size Yolk Distribution Bird Macrolecithal Telolecithal (at one end) Amphibian vegetal Mesolecithal Increasing gradient from animal to pole Placental mammal Microlecithal Isolecithal (equal) 2 Developmental Anatomy In placental mammals, the ovum is surrounded with an acellular glycoprotein layer called the zona pellucida. Surrounding this are small follicular cells called the corona radiata. The spermatozoon contains three essential components: 1. A haploid chamber tightly condensed chromosome. 2. An acrosome containing lytic enzymes important for penetrating the zona pellucida. 3. A set of structures essential for motility. Chemical Composition of Spermatozoa The principal chemical components of spermatozoa are nucleic acids, proteins and lipids. Nearly one-third of the dry weight of a single sperm cell is contributed by the nucleus. The nuclear chromatin is composed of approximately half DNA and half protein, but its contribution to the total weight of spermatozoon varies greatly because of species variability in its size. Many structural and enzymatic proteins, as well as much of the lipid, are found in the tail Inorganic Constituents Spermatozoa, containing about 2% ash, are high in phosphorus, nitrogen and sulfur. Most of the phosphorus is associated with DNA, whereas the sulfur is derived from both basic nuclear proteins and keratinoid components of the tail (Mann and Lutwak-Mann, 1981). Biochemical Components The spermatozoal nucleus is composed of condensed chromatin in which the DNA is stabilized by conjugation with the sperm-specific proteins collectively known as sperm histones. The type and composition of sperm histones varies with species (Mann and Lutwak-Mann, 1981). Sperm nuclei from some species contain mostly small sperm histones of low molecular weight known as protamines, whereas spermatozoa from other species contain varying amounts of the larger arginine-rich histones. These basic nuclear proteins, which are important for condensation and stabilization of the DNA, are held together by sulfhydryl bonds. The sulfhydril bonding, which occurs within spermatozoa, increases as the cells pass through the epididymis. A diagram of a typical mammalian sperm; B, structural features, C, D, and E cross sections through the middle piece, principal piece, and end piece at the levels indicated. 3 Developmental Anatomy A generalized diagram of the role of the cellular elements of the spermatid in the formation of the structures of the mature sperm. Seminal Characteristics and Chemical Components of Semen from Farm Animals* Characteristics and Man Bull Ram Boar Stallion Cock Components Ejaculate volume (mL) 3-4 5.8 0.8-1.2 130-200 60-100 0.2-0.5 Sperm concentration 55-200 800-2000 2000-3000 200-300 150-300 3000-7000 (million/mL) Sperm/ejaculate (billion) 5-15 1.6-3.6 30-60 5-15 0.5-3.5 Mottle sperm (%) 40-75 60-80 50-80 40-75 60-80 Morphologically normal 63-95 80-95 70-90 60-90 85-90 sperms (%) Protein (g/100 mL) 6.8 5.0 3.7 1.0 1.8-2.8 pH 6.5 6.4-7.8 5.9-7.3 7.3-7.8 7.2-7.8 7.2-7.6 Fructose 460-600 250 9 2 4 Sorbitol 10-140 26-170 6-18 20-60 0-10 Citric acid 620-806 110-260 173 8-53 Nil Inositol 25-46 7-14 380-630 20-47 16-20 Glycerol Phosphoryl 100-500 1100-2100 110-240 40-100 0-40 Choline (GPC) Ergothionineine 0 0 17 40-110 0-2 Sodium 225±13 178±11 587 257 352 Potassium 153±6 89±1 107 103 61 Calcium 40±2 6±2 6 26 10 Magnesium 8.0±0.3 6.0±0.8 5-14 0 14 Chloride 174-320 86 260-430 448 147 *Adapted from Foote (1980); Lake (1971); White (1980). Mean values of chemical components (mg/100 mL ± S.E.) unless otherwise indicated. Hyphenated values indicate ranges. PHYSIOLOGY OF REPRODUCTION Puberty and Estrous Cycle The female reproductive tract starts to function when a female reaches the age of puberty. Puberty indicates that the female has reached sexual maturity - capable of producing offspring. The age of puberty varies between breeds and among female animals of the same breed. The first manifestation to indicate that the female animal has reached the age of puberty is when it starts to show signs of estrus. In human, a girl does not show signs of heat, instead she shows or manifests signs of menstruation to indicate that she has reached that age of puberty. When the animal reaches puberty, the anterior pituitary gland starts to secrete gonadotrophic hormones which could affect the ovaries. The first gonadotropin secreted in significant amount is the Follicle Stimulating Hormone (FSH) with little Luteinizing hormone or LH. FSH causes the growth and development of the Graafian Follicle (GF) in the ovary. In turn, this developing follicle secretes a hormone known as estrogen. This is the hormone which causes estrus in female. Estrogen is also a potent inhibitor of FSH. Thus, the estrogen produced by the ovary has negative feedback on FSH production by the anterior pituitary. With the presence of estrogen, FSH production declines and at the same time stimulates production of LH. At the peak of estrogen production, FSH production decreases and LH production 4 Developmental Anatomy increases. Usually, LH is also produced with little FSH because the cells in the anterior pituitary which secrete the former are the same cells that secrete the latter These cells are the basophils of the anterior pituitary LH is the hormone that causes ovulation of maturing follicles. It also initiates the formation of corpus luteum (CL) by converting the cells of the stratum granulosum into lutein cells. Eventually, upon the action of LH, what used to be the GF will be filled up with lutein cells and becomes the CL So, the GF with the action of LH becomes the CL. The CL secretes a specific hormone known progesterone. It has a strong inhibitory effect on FSH production. As long as the CL is secreting progesterone, estrus is inhibited. Progesterone prepares the endometrium of the uterus for implantation of the fertilized egg. It also maintains normal pregnancy until birth. Thus, CL persists if there is pregnancy; however, if there is no pregnancy, the CL will regress. It is now known that the uterus secretes prostaglandins which could destroy the CL In a normal cycle or if there is no pregnancy, prostaglandins is secreted by the uterus and causes the luteolysis or regression of the CL. When this happens, FSH production would again continue and a new cycle begins. The period from one estrus to the next estrus is known as the estrous cycle. In many farm animals like carabao, cattle, pigs and horses, estrus comes every 21 days if the animal is cycling regularly although it could vary from 12 to 30 days. Animals may be classified based on the occurrence of their estrous cycle as: a. Monoestrus, if the animal comes in heat only once a year, such as dogs, b. Seasonally polyestrus, if it comes in heat at certain seasons only, such as sheep; c. Polyestrus, if it comes in heat all throughout the year, like cattle, swine and carabao. An estrous cycle may be divided into four portions: a. Proestrus which is characterized by follicular growth; b. Estrus which is under the influence of estrogen, and later followed by ovulation; c. Metestrus is characterized by the formation of CL, and d. Diestrus which is under the influence of progesterone secreted by the CL. А transition period between diestrus to proestrus is the anestrus which is the preparation to the next cycle. Animal may be classified also as spontaneous ovulators and induced ovulators. Spontaneous ovulators are those that ovulate spontaneously during or at around estrus, such as cattle, carabao, sheep, hogs, etc. Induced ovulators are those animals that do not ovulate unless there is copulation, such as rabbit. 2. FERTILIZATION Following insemination, the sperm undergoes capacitation which represents a change in the sperm to make them capable of fertilizing the ovum. Capacitation also involves removal or inactivation of decapacitating factor found on the male reproductive tract. Physiological Significance of Decapacitation Factor: a. Prolongs life of the sperm cells. b. Prevents sperm from penetrating the lining of the male and female reproductive tract. c. Prevents sperm agglutination. d. Prevents phagocytosis of the sperm in the female reproductive tract. Capacitation is a process which initiates an enzymatic alteration of surface coat proteins and a concomitant increase in sperm motility. Shortly before fertilization the acrosome reaction occurs. This is the partial disintegration of the outer membrane of the acrosome which results in the release of acrosomal contents, and is a necessary prerequisite to fertilization. If there is no capacitation, no acrosome reaction, thereby no penetration to the egg membrane. As a result, no fertilization to occur. The length of time sperm remains active and viable in the female reproductive tract differs among species, being less a day in the cow and up to a week in the dog. Fertilization is a process by which the sperm initiates and participates in the development of the egg. There is fusion of the male and female gametes which result to fertilization reaction. At the site of the fusion of the membranes the resting potential of the zygote's membrane drops. There is rapid increase in the intracellular calcium, and the contents of many small, subsurface cortical vesicles are released between the zygote's membrane and 5 Developmental Anatomy the zona pellucida. This separates the zona pellucida from the surface of the zygote (fertilized ovum), an event often referred to as the formation of the "fertilization membrane". Enzymes released by cortical vesicles cause the zona pellucida to become impermeable to other sperms and also to most potentially infectious agents. The changes in the zygote membrane also prevent polyspermy (the incorporation of more than one sperm into the female gamete) and are usually lethal. Subsequently, the metabolic activity of the cell (respiration, protein synthesis, DNA synthesis), which had been quiescent prior to fertilization, becomes reactivated. Enzymes involved in sperm penetration 1. Hyaluronidase - breaks the cumulus oophorus and corona radiate 2. Corona-penetrating enzyme (CPE) 3. Trypsin-like enzyme (TLE) breaks the zona pellucida present in the acrosomal Membrane 4. Acrosin 3. CLEAVAGE STAGES Cleavage is a series of cell division that occurs following fertilization. In mammals, cleavage occurs while the cells are still surrounded by the zona pellucida. Cleavage results in the production of many smaller cells, the blastomeres. The pattern of cleavage depends upon the amount of yolk present in the zygote. In birds’ cleavage is partial or meroblastic (meros- part) due to the massive volume of yolk sac which prevents complete division of the zygote. In placental mammals’ cleavage is total (holoblastic). Cleavage in Domestic Animals In the isolecithal zygote the planes of the second and third cleavages are at right angles to the preceding division plane. Also, after the four-cell stage, cleavage is often asynchronous and it is common to find five-, six- and seven-cell embryos. Cleavage results in the formation of a solid cluster of cells termed a morula. Prior to this stage the cells of the corona radiata are shed, but the embryo remains surrounded by the zona pellucida. In most domestic animals there are 16-64 cells in the morula by the 4th day of development while in man it consists of 9-16 cells. The blastomeres of the morula lose their spherical appearance and become tightly apposed to each other, this change is called compaction. The blastomeres secrete a fluid which collects within the morula to form a cavity called fertilization and the embryo at this stage is called compaction. The blastomeres secrete a fluid which collects within the morula to form a cavity called the blastocoel. This occurs within a week after fertilization and the embryo at this stage is called the blastocyst or blastula. During this stage the zona pellucida ruptures and the embryo breaks free. There is a marked increase in the total size and often a change in shape of the organism during this stage, in large part due to enlargement of the blastocoel. For example, the dog blastocyst becomes pear-shaped with a diameter of 1.5-2.5, which presents a 10-to 20-fold increase in size. Equine blastocysts remain spherical, but pig, cattle and sheep blastocysts undergo a tremendous elongation, expanding at a rate of up to 1 cm per hour and, in the ewe, reaching a length of 1 m by 16 days of gestation. All the cells of the blastocyst are not identical. Those in one small area become slightly larger than the rest of the cells surrounding the blastocoel. These larger cells constitute the embryonic disk (inner cell mass, blastodisk), from which the embryo will develop. The cells on the periphery of the blastocyst are the trophoblast cells. They facilitate the absorption of nutrients (trophe = nourishment) early in development and later participate in the formation of extraembryonic membranes, which contribute to the formation of the placenta. In domestic animals the trophoblast cells overlying the embryonic disk degenerate or shift peripherally and the blastodisk degenerate or shift peripherally and the blastodisk becomes exposed on the surface of the blastocyst In most primates the embryonic disk remains beneath the trophoblastic cover and is referred to as the inner cell mass. Cleavage in Birds The avian zygote is the "yolk" that one observes upon breaking open and egg. On the upper side of this large single cell there is a tiny white spot, the embryonic disk which indicates the location of the nucleus and other cytoplasmic organelles of the zygote. Meroblastic cleavage commences at this site. Four or five cell divisions occur with complete karyokinesis (nuclear division) but incomplete cytokinesis. Cell membranes do not fully surround the new cells and separate from them from each other. Thus, the early embryo is a syncytium, which is the term used to any multinucleated cell. 6 Developmental Anatomy Formation of blastomeres occurs through the growth of membranous furrows between nuclei. Soon the proliferating mass of blastomeres separates from the underlying yolk creating a space called the subgerminal cavity. Cells directly over the subgerminal cavity are the central blastomeres and, like the embryonic disk of mammals, these will form the body of the embryo. The more peripherally located cells are called marginal blastomeres, and these will form some of the extraembryonic membranes. Marginal blastomeres continue to divide rapidly and the population expands peripherally over the surface of the yolk. Only those located near the outer perimeter remain syncytial. Embryo Transfer Embryo transfer is the technique of transferring pre-implementation stage embryos from a donor female to a surrogate recipient The initial stage involves the induction of superovulation using gonadotropin in cows followed by artificial insemination. This results in the fertilization of an average of six to seven normal embryos. These embryos may be maintained for a day in an oxygenated, buffered tissue culture medium supplemented with serum or may be frozen in liquid nitrogen (-196°C) for long term storage. Upon thawing these embryos must be checked for possible damage. The stage of embryo for transfer is based on two factors: accessibility and optimal survival. Bovine embryos enter the uterus and become readily obtainable nonsurgically at 4-6 days after estrus (3.5-5 days after ovulation), which time most are at the 16-call stage or beyond. Starting at around day 8 post-estrus the blastocyst expands nearly doubling in diameter, and a day later the zona pellucida begins to disintegrate, allowing the embryo to break free. After this period the embryo becomes unprotected and could be damage easily. Thus, it is advisable to collect embryos between day 5 and day 9 post-estrus. At present commercial application of embryo transfer is limited due to several factors. a. Considerable variation in the number of embryos obtained from superovulation donors, from over 20 to less than 2. b. The technology for screening and storing of embryos is highly specialized and costly, much more so than the sperm Embryo transfer is presently used to expand the number of offspring from cows that have delivered outstanding calves, quickly introduce new breeds or strains into an area to alleles in daughters of known carriers. 4. BLASTULATION - formation of a ball of cells 5. GASTRULATION: Avian Gastrulation (gaster = belly) is the stage of rearrangement of the cells of the embryonic disk to form three separate parallel tissues called development of the embryo marked by germ layers. The outermost layer is the ectoderm, the innermost is the endoderm and the layer in between these two is the mesoderm. In birds gastrulation occurs in the central blastomeres of the embryonic disk overlying the subgerminal cavity, known as the area pellucida (clear area). The area pellucida appears clear because it is separated from the underlying yolk by the subgerminal cavity. In contrast, the more marginal blastomeres appear more opaque because they adhere to the yolk. This opaque is called the area opaca. During the late cleavage stages the central blastomeres divided into two subpopulations that are initially intermingled but appear to be of two different sizes. The larger cells which contain more yolk segregate from the smaller cells and form the roof of the subgerminal cavity. Many of these larger cells aggregate at one pole of the embryonic disk. This aggregation marks the future caudal end of the animal and is the first grossly visible asymmetry in the developing embryo. Through the process of delamination some of the larger, more deeply located cells break away from the mass of smaller cells and move into the subgerminal cavity. These cells and those cells migrating from caudal aggregate into the subgerminal cavity form a new layer called the hypoblast. The cavity below the hypoblast is still the subgerminal cavity but the newly formed cavity above it is called the blastocoel. The thicker layer of smaller cells roofing the blastocoel is now called the epiblast. Expansion of the epiblast in the caudal end of the embryonic disk results in the accumulation of cells in this part of the developing embryo. Gastrulation begins as soon as the hypoblast is established and starts at the caudal end of the embryonic disk. The major stages of gastrulation are: 7 Developmental Anatomy a. Oriented radial expansion of the epiblast and caudal convergence of cells. The epiblast occupies a greater area towards the cephalic end of the embryonic disk white the cells in the caudal region accumulate in the midline. b. Formation of the primitive streak. The primitive streak is a median thickened are of the epiblast which results from the convergence and caudal condensation of cells. The primitive streak is elongated. It marks the location of the future longitudinal axis of the embryo. As the epiblast expands the primitive streak also elongates. The primitive streak presents a hairpin appearance with a slightly widened cranial tip called the primitive node (Hensen's node, primitive knot). c. Involution of epiblast cells. Formation of endoderm and mesoderm. The primitive streak presents in its center a longitudinal furrow called the primitive groove. This depression marks the site at which cells are leaving the epiblast. The first cell to break away invade hypoblast layer causing the original hypoblast cells to the displaced peripherally. These new epiblast-derived cells in the deeper layer will form the intraembryonic endoderm. Majority of the cells that leave the epiblast at the primitive streak form a loose mesenchymal population located between the epiblast and hypoblast (endoderm) layers. This population is called the mesoderm. As gastrulation proceeds, more epiblast-derived cells break away from the primitive streak to join the mesodermal population resulting in the lateral expansion of the mesenchymal population. d. Regression of the Primitive Streak. Formation of the notochord. The cranial third of the epiblast which was the last area to participate in gastrulation is the first to stop expanding. When the cells in this region have already migrated away as mesoderm no additional cells arrive at the midline to replace them. As a result the streak appears to regress (shorten) with the primitive node shifting position continually closer to the caudal end of the embryo. During regression, cells in or close to the primitive node are deposited beneath the epiblast in the midline to form the notochord and paraxial mesoderm. The primitive streak in chick embryo reaches a maximum length of 2 mm and extends about two-thirds of the craniocaudal length of the embryonic disk. Gastrulation in avian begins at about 24-30 hrs after fertilization which is usually about 6 hours after the egg is laid. The maximum length is attained at about 18 hours of incubation and the primitive streak disappears by 2.5 days (about 60 hrs) of incubation. Gastrulation is completed when the primitive streak has regressed completely. The epiblast cells that did not undergo involution and remain in the upper surface of the embryo are now called the ectoderm. Mesoderm is formed from the entire length of the primitive streak. While the streak is undergoing regression the lateral margins of the mesoderm expand laterally and rostrally eventually meeting in the midline in the front of the embryo. The first mesodermal cells formed become the extraembryonic membranes while the last mesodermal cells to be formed from the epiblast remain closest to the dorsal midline of the embryo to become the paraxial mesoderm. Gastrulation: Primates and Domestic Animals Gastrulation in most domestic animals occurs towards the end of the 2nd week of gestation and several days earlier in laboratory animals. The morphogenetic processes in mammalian gastrulation are similar to those in avian except for some conspicuous differences which are associated with the necessary in mammals to form extraembryonic tissues very early in development. During the blastocyst stage, cells delaminate from the inner surface of the embryonic disk and expand beneath the trophoblast to form a thin continuous sheet lining the interior of the blastocyst, establishing a tube of hypoblast inside a tube of trophoblast. The cavity of the hypoblast tubed is called the archenterons or primitive gut. Most of this tube will not be included inside the embryonic body and will become the extraembryonic yolk sac. Formation of the primitive streak occurs in the epiblast cells of the embryonic disk, which occupies a relative small area of the blastocyst compared with the extraembryonic trophoblast. The epiblast cells which move inwards at the primitive streak become the definitive endoderm, the mesoderm of the embryonic axis, including the notochord and somites, and also the adjacent lateral mesoderm. In mammals like the pig, sheep and cow, in which the blastocysts become very elongated, the lateral mesoderm spreads quickly away from the embryonic disk. The lateral mesoderm splits into two layers, one associated with each of the epithelial tissues present. The mesoderm underneath the trophoblast and embryonic ectoderm is the somatic mesoderm and 8 Developmental Anatomy the two layers combine to form the somatopleure. The mesoderm surrounding the hypoblast and the embryonic endoderm is splanchnic (visceral) mesoderm and the combine layers are called splanchnopleure. After regression of the primitive streak the cells remaining on the surface of the embryonic disk constitute the ectoderm. In primates the inner cell mass is usually retained inside of and attached to the trophoblast. During the early stages of gastrulation the trophoblast cells separate from the inner cell mass allowing the cells of the epiblast to undergo morphogenetic movements similar to those described above. Schematic median view of late gastrula and early neurula stages, illustrating in B, the elevation of the head process, and in C and D, initial folding of the surface of the embryonic disk to form the subcephalic pocket and the rostral part of the pharynx. At the same time, the primordium of the heart is folded beneath the embryo. Two cells have been blackened to help clarify the translocations occurring at these stages. Arrow in D passes through the cranial intestinal portal. *Chart indicates origin of epithelial part of organ only - These organs all have secondary supporting investments of mesodermal origin. Chart showing the derivation of the various structures of the body by progressive differentiation and divergent specialization. Note especially how the origin of all the organs can be traced back to the three primary germ layers, ectoderm, entoderm, and mesoderm. 9 Developmental Anatomy The Germ-Layer Origin of Tissues Mesoderm Endoderm Ectoderm (including mesenchymes 1. Epidermis, including: cutaneous 1. Muscle (all types) Epithelium : glands, hair, nails, lens 2. Connective tissue, cartilage, 1. Pharynx including root of 2. Epithelium of: bone, notochord tongue, auditory tube, tonsils; Sense organs, nasal cavity, 3. Blood, bone marrow thyroid parathyroid, thymus sinuses. mouth, including: oral 4. Lymphoid tissue 2. Larynx, trachea, lungs glands, enamel, anal canal 3. Digestive tube including 3. Nervous tissue, including: Epithelium of: associated glands hypophysis, chromaffin tissue 5. Blood vessels, lymphatics 4. Bladder 6. Body cavities 5. Vaginal, labia, vestibule 7. Kidney, ureter 6. Urethra, including associated 8. Gonads, genital ducts glands 9. Suprarenal cortex 10. Joint cavities (From Arey, Developmental Anatomy, Courtesy of W. B. Saunders Co. 6. NEURULATION Neurulation is the stage of embryonic development marked by the formation of the central nervous system, the initial development of the gut and the heart, and the appearance of segmented paraxial mesoderm. During this stage the body of the embryo begins to delineate within the three germ layers established during gastrulation. The first indication of neurulation is the thickening of the surface ectoderm along the dorsal midline to form the neural plate, which is bounded on both sides by slight elevations called neural folds. The plate becomes depressed in the midline forming the neural groove and the neural folds become more elevated. Subsequently these folds converge towards the dorsal midline, meet and fuse together resulting in the formation of the closed neural tube that is separated from the overlying surface (superficial) ectoderm. As the neural tube is being formed the cephalic region of the avian embryo begins to grow above the surface of the blastodisk and to elongate rostrally. This rostral elongation is the first step in the formation of the embryonic body and occurs in two stages: a. As the cephalic neural tube elongates it overgrows the underlying ectoderm and endoderm. b. The first stage results in the formation of a transverse furrow called the subcephalic pocket located beneath the rostral margin of the forebrain. The elongated cephalic tissues are collectively called the head process. After the initial rostral elevation of the head, the surface (superficial) ectoderm lateral to the brain begins to fold downward resulting in the formation of furrows called the lateral body folds. These folds are continuous with the subcephalic pocket. As growth progresses the lateral body folds become deeper, eventually undercutting the entire cephalic end of the embryo and separating the head process from the underlying extraembryonic membranes. At a later stage an identical process will begin at the tail region and proceed progressively cranially. The cranial and caudal portions of the lateral body folds eventually meet at the level of the umbilical stalk which connects the embryonic body with the extraembryonic structures. During neurulation the gut (digestive) tube also begins to form. The most rostral tip of the gut tube, the pharynx is formed when the subcephalic pocket expands beneath the head process. The right and left endodermal folds meet in the ventral midline and fuse forming a closed endodermal tube. This process proceeds cranio-caudally and later extends cranially from the hindgut region. The transient openings from the gut into the subgerminal cavity (in birds) or archenterons (mammals) are called the cranial and caudal intestinal portals. As the gut closes beneath the level of the hindbrain cardiogenic mesoderm beside the paired endodermal folds is brought to the ventral midline. A tube of endothelium on each side will fuse to form a single cardiac tube. The cardiac tube will expand to the right, loop 180 degrees and become subdivided into a four-chambered heart. 7. ORGANOGENESIS - formation of organ systems. Assembling and molding of embryonic tissues into fully functional organs. Embryonic Duplications and Twinning Multiple birds are caused by: a. Fertilization of separately ovulated female gametes; b. Complete or partial separation of cleavage-stage blastomeres and blastocysts or, c. Duplication during gastrulation. 10 Developmental Anatomy Embryos from multiple births are classified as free (unattached to each other) or cojoined, and symmetrical or asymmetrical. a. Free (separate), symmetrical, dizygotic twins. This group includes most normal twins. Those from separate zygotes are called dizygotic twins. Each embryo develops independently with its own separate set of fetal membranes although in some species fusion of extraembryonic membranes occurs. In the cow this includes fusion of the allantoic blood vessels, which allow blood to be exchanged between the fetuses. If the twins are of different sexes the exchange results in the abnormal development of the genital system of the female and the production of a freemartin. b. Free, symmetrical, monozygotic twins. These are identical twins derived initially from a single zygote which separates or duplicates at several different stages. The two blastomeres produced from the first cleavage may separate and form each an embryo. This separation more frequently occurs later during cleavage or blastocysts stages. Two separate sets of extraembryonic membranes will be formed whenever twinning is initiated during these stages. An abnormally small rupture in the zona pellucida permits some but not all of the blastocyst to break free. This may allow the formation of monozygotic twin bovine embryos. Provided that both hemisected blastocysts contain part of the embryonic disk, each half may develop normally. In some instances, the embryonic disk will split into two separate parts immediately prior to gastrulation wherein there is usually some sharing of extraembryonic membranes between the two fetuses. c. Free asymmetrical twins. This type of twins originates from monozygotic or dizygotic twins. In asymmetrical free twins one member is normal while the other is rudimentary in development and survives by being attached to the blood supply of the fetal membranes of the normal twin. The abnormal twin has no recognizable body form and consists of skin with pigment and hair, connective tissue, bone, teeth, muscle and rudimentary digestive organs. Specific craniofacial structures can sometime be identified. The abnormal twin is usually surrounded by its own amnion. The abnormal twin has also been called various names such as: 1) amorphous globosus; 2) anidian (formless) fetus: and 3) acardiac fetus or holocardius Among domestic animals acardiac twins are most common in the cow. This abnormal twinning should be differentiated from the condition known as mummified fetus or lithopedian (stone child) in which development of a normal twin as arrested. Rather than being resorbed, the twin becomes dehydrated and shrunken. Mummified fetus can cause dystocia (abnormal delivery) or, if retained, lead to or interfere with a subsequent pregnancy. Mummified fetuses are most commonly found in cows. d. Cojoined or fused symmetrical twins. These twins are monozygotic in origin and represent incomplete division of one embryo into two components, usually at some time during the primitive streak stage. If the twins are nearly complete, they are generally termed diplopagus (2-fold joined), the preferred equivalent of Sieamese twins. Such twins are identified according to the site of attachment. 1) Thoracopagus - twins are joined at the sternal region of the thorax, facing each other, often with partially fused of compromised hearts. 2) Abdominopagus twins - are joined at the abdomen, often with partially fused intestines 3) Pyopagus - twins are joined back to back at the pelvis or scrum (pygus = buttocks). 4) Cephalopagus (craniopagus) - twins are jointed in the head region. Duplication of one part of the future axial (and adjacent) structures is also possible. This usually arises during the elongation or regression of the primitive streak. This set of anomalies is described by using the prefix di(or tri-, tetra-, etc.) and the appropriate region-specific suffix. Examples: 1) dicephalus two heads 2) diprosopus two faces 3) dicaudatus two tails 4) tetrabrachius two pairs of thoracic limbs 5) tetrascelus two pairs of pelvic limbs e. Cojoined asymmetrical twins. These twins are unequal in size (heteropagous) and consist of one reasonably normal individual, the autosite, with an extra body part, the parasite, attached to it. A frequent manifestation of this is the formation of an extra limb attached along the back of the animal. This is called notomelus (not = back; melus = limb). Another example of cojoined asymmetrical twinning is a normal animal with an extra set of pelvic limbs projecting caudally from its ischial arch. 11 Developmental Anatomy Asymmetrical cojoined twins usually arise after gastrulation when specific organ-organized forming regions called fields (limb field, heart field, eye field, etc.) are becoming organized. Development of avian extraembryonic membranes. A and B show transverse sections on the 3rd and 5th days of incubation, during which time the large mass of yolk becomes partially encompassed by the yolk sac. C and D are lateral views of the 5 th and 8th days of incubation to illustrate the development of the allantois. Incubation times for domesticated birds Bird Days Quaila 16 Budgerigar 18-20 Chicken 21 Pheasantb 22-28 Peafowl 28 Duckc 28 Turkey 28 Goose 28-32 a Domesticated Coturnix (Japanese) quail. b Varies according to species. c The Moscovy Duck is 35-37 days. EXTRA EMBRYONIC MEMBRANES AND PLACENTATION Extraembryonic membranes are structure which protect the embryo and allow exchange of gases as well as passage of nutrients. There are four extraembryonic membranes, namely: a. Amnion b. Chorion 12 Developmental Anatomy c. Allantois d. Yolk sac The amnion and chorion are derived from somatopleure while the allantois and yolk sac are from splanchnopleure. Although called membranes, most embryonic membranes are initially composed of two layers, somatic or splanchnic mesoderm plus ectoderm or endoderm. Avian Extraembryonic Membranes a. Yolk sac. This extraembryonic membrane is derived from the endoderm and the adjacent mesoderm which directly cover the yolk. The outer perimeter of the yolk sac is marked by a blood vessel, the sinus terminalis or marginal vein, which demarcates the extent of growth of the splanchnic mesoderm over the yolk. By the 6" day of incubation in the chick, the yolk sac encloses most of the yolk, and is vascularized by vitelline blood vessels in the splanchnic mesoderm. These vessels pass into the embryo along the yolk stalk, which is formed by the completion of body folding and closure of the embryonic gut tube. Yolk does not pass directly into the embryonic digestive tract but is absorbed through the yolk sac endoderm and endothelial lining of the vitelline blood vessels. Thus, nutrients pass into the embryo via the vitelline veins. On the 19" day of incubation in chick, which is 2 days prior to hatching, the yolk sac is drawn into the abdominal cavity and the remaining undigested yolk is entirely absorbed by about 6 days after hatching. b. Amnion and chorion. Simultaneous with the process of body folding, a pair of folds called the chorioamniotic folds consisting of embryonic somatopleure begin to elevate. The chorioamniotic folds initially form cranial to the head and elevate progressively more caudal levels on both sides of the embryo. Later in development these changes are repeated at the caudal and of the embryo forming a pair of folds that grow progressively cranially. The chorioamniotic folds expand dorsally then meet and fuse over the dorsal midline of the embryo. As a result of this growth of folds two layers at somatopleure, separated by the extraembryonic coelom, now extend over the embryo. The outer layer of somatopleure is the chorion and the inner layer is the amnion. The ectoderm of the amnion is continuous with the ectoderm of the embryonic skin at the umbilical stalk. A longitudinal raphe called the chorioamniotic raphe or mesamnion marks the point of fusion of the left and right chorioamniotic folds. In some species of birds (and animals) the chorioamniotic raphe persists while in others it appears leaving no connection between the amnion and chorion as chorioamniotic raphe persists in the chick. The cavity between the embryo and the amnion, the amniotic cavity is filled with amniotic fluid, initially a product of secretions from the amniotic ectoderm but later receives fluids from the fetal kidneys, oral glands and respiratory tract. The amniotic fluid serves to buoy and protect the embryo and provides environment in which the embryo can move its body and limbs. Initially blood vessels are absent in the chorion or amnion. The chorion and the allantois are sites of exchange of the water. c. Allantois. This extraembryonic structure is derived from the splanchnopleure and arises as a diverticulum in the hindgut of embryo. It first appears as a small vesicle on the sight aide a 3-day embryo in the extraembryonic coelom. By 10 days of development the allantois fills the cavity between the amnion and the chorion. The allantoic splanchnic mesoderm meets and fuses with the splanchnic mesoderm of the yolk sac and also with somatic mesoderm of the amnion and chorion. The allantois is supplied by allantoic arteries which branch off the caudal portions of the two dorsal aortae. Allantoic (umbilical) veins enter the embryo at the umbilical stalk and course cranially in the ventral body wail to enter the sinus venosus of the heart, along with the vitelline veins. The allantoic cavity collects the urinary excretory wastes, most of which precipitate as uric acid in birds. As the embryo and its extraembryonic membranes develop, the volume of albumen is reduced, mostly by the transfer of its fluid content to the embryo. The space it originally occupied is taken up by the embryo. When the yolk sac and chorion reach 13 Developmental Anatomy the ventral margin of the yolk, a small opening remains where yolk and albumen are continuous. This margin of extraembryonic membrane is the yolk sac umbilicus. Later, when the allantois expands in the extraembryonic coelom and reaches this location, a layer of chorion grows over the surface of the shrinking albumen sac is enclosed in the abdomen of the embryo along with the yolk sac. Placenta The placenta of the eutherian mammals (all mammals except marsupials and egg-laying monotremes) is a structure formed by the position of fetal membranes and maternal tissues. The primary function of the placenta is to selectively mediate psychological exchanges between the fetus and mother. The placenta, in particular the fetal components, acts as a barrier to prevent fetal and maternal blood from mixing. Superficially, the fetal membranes of mammals are very similar to those of birds (and reptiles). One major difference is that there is little or no yolk in the mammalian zygote, and as such, the yolk sac is often transient and not as well developed. The rudimentary yolk sac has an essential role in the initial stages of hematopoiesis (blood cell formation) and, in some species, is the source of germ cells. The chorioallantois is the principal fetal component of the mammalian placenta. Prior with the position with the uterine endometrium, the mammalian embryo obtains its essential nutrients by absorption from the fluid contents of the uterine cavity. This fluid called histotrophe or “uterine milk” is a secretory product of the uterine glands. With the formation of the placenta, the histotrophic nutrition is supplemented by the hemotrophic nutrition, in which essential metabolites are provided by the maternal circulatory system. The fetal components of the placenta consist of three layers, namely: a. Endothelium which lines the allantoic blood vessels. b. Chorioallantoic mesodermal connective tissue layer. c. Chorionic epithelial cell layer which is derived from the epithelial layer of the trophoblast. The maternal components of the placenta consist of three layers, namely: a. Vasculine endothelium b. Uterine connective tissues c. Surface epithelium The placentas of all eutherian mammals vary in type according to the number of tissue layers that remain intact between the fetal and maternal circulating blood and to the distribution of contact sites. In domestic animals two types of placentas are present on the basis of histological features. a. Epitheliochorial placenta occurs in the horse, pig and cattle and in parts of the placenta of sheep and goats. In this type placenta the fetal chorion is in contact with the maternal uterine epithelium. There is no loss of endometrial tissue during attachment and throughout gestation. At birth maternal endometrium remains intact and is not sloughed off during parturition. This placenta is therefore also called non-desiduate (a = not; deciduous = a falling off), The domestic ruminant is an exception because although the attachment is epitheliochorial, during parturition a portion of the endometrium which formed the functional placenta is sloughed. Thus, in these species a partially deciduate placenta exists. b. Endotheliochorial placenta occurs in carnivores such as the dog and cat. In this type of placenta, the endometrial epithelium and connective tissue are lost during attachment, leaving the uterine vascular endothelium in contact with the fetal chorion. Loss of endometrium occurs during placental development and at birth. This type is also called deciduate placenta. In most rodents and many primates including human, the maternal uterine vascular endothelium is also lost resulting in free maternal blood bathing the chorionic cells. This type of placenta is called hemochorial placenta which is also called deciduate. Placentas are also classified according to shape and vascular arrangement. The various shapes refer to the arrangement specialized zones of apposition between fetal and maternal tissues where physiological exchanges occur. The types of placenta according to the above classification are the following: 14 Developmental Anatomy a) Diffuse Placenta In which the zone of apposition is distributed over most of the chorioallantois, e.g., horse and pig. b) Cotyledonary Placenta In which the zone of apposition is confined in discrete areas called the uterine caruncles in the uterine mucosa and fetal cotyledons in the fetal chorion. The apposed uterine caruncles and fetal cotyledon are collectively called placentome, e.g., ruminants. In domestic animals, the chorionic epithelium branches villi at the site of apposition with maternal tissues. These villi are epithelial-lined cylinders filled with mesenchyme and allantoic blood vessels, and they established a placenta. In some species such as carnivores, dissidents and primates, these will coalesce and the allantoic vessels form irregular, tortuous channels. This vascular arrangement is termed a labyrinthine placenta. Extraembryonic membranes of the dog. The membranes of a cat would appear similar except that the yolk sac is less prominent. Fetal-maternal apposition in domesticated mammalsa,b Animal Attachment beginsC Size of trophoblast at this stage Attachment completeC Cat 11-12 4 mm 16-17 Cattle 28-32 200+ cmd 40-45 Dog 14-17 4 mm 20-21 Horse 35-40 6-7 cm 95-105 Sheep 45-18 10-20 cm 28-35 Swine 12-13 Up to 1 md 24-26 a Data adapted from McLaren (1980); and Evans and Christesen (1979) b Data refer to definitive, chorioallantoic attachment, in some of these species there are earlier choriovitellinic attachments. c Days after ovulation. d Usually equals or exceeds the length of the uterine tube. Apposition and Implantation Embryonic tissues come in contact with the uterine wall shortly after entering the uterus. In most domestic animals, the site of permanent contact is antimesometrial (opposite to the line mesenteric attachment) and in pig and some ruminants, this side of the uterus shows an increase in the vascularity prior to contact by the blastocyst. The term implantation is commonly used to describe the attachment of the trophoblast to the uterine lining. In most large domestic animals, the attachment is none invasive and fetal tissues can be physically separated from the uterine epithelium. Rodent blastocysts settle into uterine crypts, which are depressions in the uterine lining that subsequently close around the embryo and separate it from the lumen of the uterus. In most carnivores, rodents and primates, including man, attachment is interstitial; the trophoblast invades and partially destroys the endometrium. 15 Developmental Anatomy Later, adjacent uterine mucosal tissues may close around the embryo, a process that is called nidation (nidus = nest). CONCEPTS AND MECHANICS OF DEVELOPMENT Definitions There are four developmental processes which operate during the early stages of development. These are the following: a. Growth is an increase in the size and number of cells in the whole or any part of the organism. b. Morphogenesis includes any change in the shape or location of a cell or tissue. Examples are the expansion of the epiblast and involution of the primitive streak, closure of the neural tube, fusion of the heart tube and bending of the cardiac loop. c. Patterning is the establishment of programmed subsets of cells in proper relation to each other and to surrounding tissues. Examples are the positioning of feathers or hairs in the skin and of teeth in the oral cavity or the locating and shaping of bones and muscles in limb. A more complex patterning occurs in the development of musculoskeletal systems, such as the axial system, the face jaws and limbs. Shortly after the end of neurulation, the precursors of forelimb and hindlimb structures appear as pairs of epithelia-covered mesenchymal swellings called limb buds which are located on the dorsolateral margin of the embryo. If mesenchyme from the wing bud of a chick embryo is removed and replaced with leg bud mesenchyme from a duck embryo, a limb having the gross appearance of a duck’s leg develops in the chick thoracic region. Thus, the information for patterning of a complex set of structures was present in the primordium in advance. d. Cytodifferentiation is the complex, often tortuous process by which each cell or cell line attains and expresses a stable neophyte. This process usually occurs over the course of many cell generations, with some of these generations of cells expressing intermediate characteristics. Although the actual genetic events marking the onset of cytodifferentiation are not known, it is well established that during early cleavage stages each of the blastomeres has the capability of forming the complete embryo. In animals, the first visible difference between cell types occurs with the formation of the morula, at which some blastomeres are located internally surrounded by the surface blastomeres. However, it has been shown that if the position of any of these blastomeres is experimentally altered, the cell will develop in accordance with its new position. This means that all the blastomeres are equipotential during early stages of cleavage in mammals. The same is not true by the blastomeres stage. Cells located in the inner cell mass are capable of forming trophoblast if their position is surgically altered, but trophoblast cells have by this stage lost the ability to develop as part of the inner cell mass. By the expanded blastocyst stage, only a few hours later, the cells of the inner cell mass have also become restricted and lost their ability to form certain extraembryonic tissues. Experimental analyses have shown that once the three germ layers are formed, each has developmental potentials than the others. Shortly thereafter the first specific cell lines are delineated, including cardiac muscle and blood cells, notochordal cells, somites and neural cells. The four developmental processes are all interrelated and usually interdependent. A genetic or teratogenic insult that disrupts any one of these processes will inevitably cause secondary alterations in all of them. 16 Developmental Anatomy Morphogenetic changes exhibited by epithelial and mesenchymal tissues during early embryonic development. Tissue Interactions During the gastrula, neurula and early organogenesis stages, interactions continuously occur between the adjacent tissues. These interactions are necessary for the subsequent development of one or all tissues involved. a. Lens Induction. The possibility of a causal relationship between two events was first demonstrated by lens induction. This was established by Hans Spemann who showed that the presence of the optic vesicle immediately beneath the surface ectoderm at the future site of lens formation was in some necessary for the lens to form. Spemann tested the theory of causal relationship by removing the optic vesicle from an amphibian embryo before it contacted the presumptive lens-forming surface ectoderm. As a result, no lens formed at this site. This demonstrated that the action of one tissue or at least its physical presence is necessary for the development of another tissue. More recently it has been found that the optic vesicle is not the only tissue to influence the presumptive lens epithelium. Lens formation was shown to influenced also by endodermal cells that will form the pharynx and later by presumptive cardiac mesoderm. This recent finding illustrates two important aspects of tissue interaction: 17 Developmental Anatomy 1) That tissue inductive interactions are not a singular event, but rather a series of events with cumulative effects. 2) That inductive interactions may occur long before the final expression of a cell's phenotype. b. Skin. The integument is a useful tissue to study tissue interactions because the structures that develop from the ectodermal epithelium vary from one region to another. In birds these include different types of feathers, scales on the legs and feet, the beak, the comb and the wattle. Structures formed by surface ectoderm are those appropriate to the original location of the underlying mesenchyme. Thus, the underlying mesenchyme controls not only the pattern of epithelial differentiation also the types of structures formed. The range of specialized cell types that the surface ectoderm can form is restricted by both the ontogenetic and phylogenetic histories. For example, it will not form the kidney (from mesoderm) or the intestinal (from endoderm) cells when grafted adjacent to the appropriated inducing tissues. These capabilities have been lost during earlier stages. Furthermore, the surface ectoderm is not capable of forming tissues not within its genetic repertoire. Avian ectoderm will form rudimentary feathers but not hair when cultured with mammalian dermis. An exception however is the reported ability of the avian oral epithelium to form enamel-producing tooth tissues when grafted adjacent to mouse oral mesenchyme. These findings suggest that modern birds have retained the genes coded for enamel proteins for over 100 million years, and that the inductive stimulating from murine oral mesenchyme can elicit this long quiescent response. c. Gut. The lining of the gut and other organs associated with it are formed by endoderm. At the start of gastrulation the endoderm is surrounded by splanchnic mesoderm. If the pancreatic primordium which develops from the gut endoderm caudal to the stomach isolated from the mesenchyme, the pancreas will not fully develop. When either pancreatic mesenchyme or mesenchyme from other gland such as lung or salivary gland is combined with the endoderm. Normal pancreatic development occurs. In a few cases however, including the lung, stomach and mammary gland, heterologous mesenchyme can alter the normal course of epithelial differentiation. For example, avian gizzard epithelium combined with proventricular (gastric) mesoderm will develop gastric glands, which indicates that mesoderm can alter the programming of endodermal tissues in certain situations. These data are important for two reasons: a. They illustrate the broad range of phenomena encompassed by the term inductive tissue interaction. In some instances, the mesenchyme appears to direct or instruct the developing epithelial component: in others, it plays a permissive role. In many situations, there is reciprocal exchange between adjacent tissues. b. At the stages during which many of the interactions are occurring, one and possibly both, of the components are already programmed. In the case of the integument, the dermal mesenchyme contains necessary information to pattern itself on the overlying ectoderm. In case of the pancreas, one specific part of the endodermal tube was specified to form a pancreas, and would develop as such so long as there was a permissive environment. This means that some parts of the gut endodermal tube are inherently patterned, most of the surface ectoderm is not. These instructions do not represent the initial step in the development of either the skin or the gut, rather, they are but one of a series of programming events, a series that must have begun at earlier stages of development. Primary Induction Primary Induction refers to events occurring during blastula and gastrula stages that lead to the formation of axial tissues (neural plate, paraxial mesoderm, and notochord). During blastulation and gastrulation, interactions also occur between developing surface epiblast and underlying mesenchyme, particularly the primordial of the notochord and paraxial mesoderm. As result of these interactions, one part of the surface ectoderm becomes uniquely programmed to form neural tissue, with the various cranio-caudal regions specified. The paraxial mesoderm becomes committed to form metameric somites, and the mesoderm in the midline condenses to form the notochord. The subsequent development of nervous, muscular and skeletal systems depends largely upon these early interactions. NERVOUS SYSTEM Nerve tissues develop from embryonic ectoderm that is induced to differentiate in this direction by the underlying notochord. First, a neural tube plate forms; then the edges of the 18 Developmental Anatomy plate thicken, forming the neural groove. The edges of the groove grow toward each other and ultimately fuse, forming the neural tube. This structure gives rise to the entire CNS, including neurons, glial cells, ependymal cells and the epithelial cells of the choroid plexus. Some cells lateral to the neural groove become the neural crest and give rise to most of the Peripheral Nervous System (PNS), as well as a number of other structures. Neural crest derivatives include the following: 1. Chromaffin cells of the adrenal medulla 2. Melanocytes of skin and subcutaneous tissues 3. Odontoblast 4. Cells of the pia mater and the arachnoid 5. Sensory neurons of cranial and spinal sensory ganglia 6. Postganglionic neuron of sympathetic and parasympathetic ganglia 7. Schwann cells of peripheral axons 8. Satellite 9. Craniofacial connective tissue Neural tube is the primordium of the brain and spinal cord. Cells beside it breaks away to form the neural crest which contributes to the: 1. Peripheral ganglia 2. Medullary parts of the adrenal gland 3. Craniofacial connective tissue The rostral neuropore (neural tube) closes first. Transverse section of the tube has three concentric layers: 1. Innermost layer (germinal layer) sheet of neuroepithelial cells that persist as ependymal cells, lining the central canal and ventricular system. Neuroepithelial cells proliferate and migrate outward into the middle to become the, mantle layer 2. Mantle layer - immigrant cells which are precursors of neurons becomes the gray matter. Mantle layers become arranged in dorsal and ventral columns that bulge into the lumen of the tube. a. Dorsal bulge (alar plate) dorsal horn or column, the afferent system b. Ventral bulge (ventral plate) ventral horn or column efferent neurons. Later, four groups of neurons are organized; arranged in dorso-ventral sequence, compose the gray matter of the spinal cord: a. somatic afferent b. visceral afferent c. somatic efferent d. visceral efferent Processes of the cells extend outward and formed the, marginal layer 3. Marginal layer consisting of the dendrites and axons becomes the white matter. PRIMARY DIVISIONS SUBDIVISION MAJOR DERIVATIVES LUMEN Telencephaton Cerebral cortex Lateral Ventricle Basal nuclei Prosencephalon Limbic system Epithalamus Third Ventricle Diencephalon Thalamus Hypothalamus Mesencephalon Tectum (Corpora Cerebral aqueduct quadrigemina) Tegmentum Cerebral peduncles Metencephelon Pons Rostral part of 4th Cerebellum ventricle Rhombencephalon Myelencephalon Medulla oblongata Remainder of neural Spinal cord Central canal tube 19 Developmental Anatomy Complete displacement of NCC is accomplished by two mechanisms: 1. Active cell migration - embryonic environment into and through with cc migrate dictates the course of their movement. 2. Passive translocation - establishing patterns of pigmentation Control of autonomic serum differentiation 3 phases: 1. Determination - commitment to develop into an auto neuron 2. Cytodifferentiation - appearance of biochemical characteristics unique to a sympathetic or parasympathetic neuron 3. Maturation Elevation of the characteristics into an adult level. One component involved in the maturation is the nerve growth factor (NGF). Preganglionic nerve ending in the ganglia contains enzyme, choline acetyl transferase while Postganglionic neuron contains tyrosine hydrolase (TOH) the 1st enzyme in the synthesis of norepinephrine Peripheral nerve - target tissue interaction Interactions between nerves and their target tissues play essential roles in the development of somatic efferent and afferent neurons and voluntary muscles. DEVELOPMENT OF THE EYE Eyes - started as an ectodermal thickening Optic groove - 1st neural part of the eye to appear - deepens to a lateral evagination of the brain wall to form optic vesicle - outer wall thickens and invaginates to form optic cup - opens ventrally and extends proximally to form an optic stalk - a constriction between the optic cup and the brain Lens placode Ectodermal thickening overlying the optic vesicle Invaginate to form the lens vesicle separates from the surface ectoderm: the latter layer forms the cornea (superficial layer) deeper layer derived from neural crest mesenchymal cells Lateral surface of optic vesicle forms bilayer optic cap inner surface gives rise to neural retina outer surface layer gives rise to pigmented epithelium of the retina Connective tissue in the choroids, sclera and iris are derived from neural crest cells and minor contribution from mesoderm. Blood vessels that develop in the nearby mesenchyme from the hyaloid artery Hyaloid veins - drains the area Development of the inner ear. Ear is divided into: 1. External 2. Middle 3. Inner Each have different embryonic origin. External - derived from the visceral arch 2 Auricular hillock - swelling which arises from the mesenchyme found in both sides of the 1st visceral grove. 20 Developmental Anatomy Pinna - comes from the 1st visceral arch External auditory meatus - grove between the 1st and 2nd hillock Initially: develop on the ventrolateral surface of the head don't shift their location but growth of the lower jaw and associated muscles expands the volume of the tissue ventral to the ear. Associated with 7th CN Inner ear - focal thickening of the surface ectoderm called otic placode Invaginate to form otic cup and comes in contact with myelencephalon Lip of cup closes and separate from otic vesicle Classification of PN PNS consist of: 1. motor or efferent component- conducts impulses away from CNS 2. sensory or afferent - conduct impulses towards CNS Entry tissues in the body except CNS are innervated by efferent and afferent neurons although relative numbers of each type varies greatly, e.g., muscles – have plenty of efferent and few afferent integument/skin - have plenty of afferent and also have efferent neuron to peripheral blood vessels, skin glands, as feather or hair muscles. Separation of the PNS into afferent/efferent components 1. Motor neurons dendritic zones and cell bodies are located in the CNS or in peripheral ganglia and axons extend to target tissues. 2. Sensory - dendritic zones are located in the peripheral tissues and axons project centrally. Most afferent neurons are located in spiral and cranial sensory ganglia except those associated with vision and olfaction All PNS neurons are categorized as: 1. Visceral includes innervation of all voluntary muscles including those of peripheral bld. vessels and the integument derived from somatic mesoderm and the head from NCC 2. Somatic afferent/efferent innervates voluntary muscles and related CT and those ectodermally derived epithelia like skin, mouth and other sense organ. Digestive Apparatus Comprises the organs concerned into the reception, mechanical reduction, chemical digestion absorption of food and drink with the elimination of unabsorbed residue. Consist of the alimentary tract, extending from the mouth to the anus, and certain glands - salivary, pancreas, and liver, Primarily formed of endoderm, the germ layer that lines the yolk sac, Muscle and CT that supports the epithelium are of mesodermal origin. Development: Digestive tube separates from the yolk sac achieved through folding process that results to the convention of the flat embryonic disc into a more or less cylindrical body. Gut - included part of the yolk sac three regions: 1. foregut 2. midgut 3. hindgut Midgut - joins with the cranial and caudal intestinal portal Foregut and midgut end blindly at the oral and cloacal membrane, circumscribed median areas where endoderm and ectoderm are in direct contact. The membrane is known as the stomodeum and proctodeum. When membrane breakdown they become confluent with the gut, cranial extension forms the mouth or the oral opening. Caudal is the anal canal or opening 21 Developmental Anatomy Stomodeum - located between the swelling of the forebrain and above the developing heart ventrally. Proctodeum - a small dorsal diverticulum of the ectoderm grows out immediately in front of the plate and extends towards the undersurface of the brain - later becomes associated with a downgrowth from the forebrain and loses its connection with the oral ectoderm and transformed into the anterior lobe of the hypophysis. Foregut - differentiates to form the pharynx, esophagus, stomach and first part of the duodenum. Midgut forms the small intestine. Mouth - forward growth of process which appears around the margins of the oral plate. Dorsally frontal process - the result of apart in growth of the paraxial mesoderm laterally and ventrally - formed by the mandibular arch (the 1st thickening in the mesoderm lateral to the presumptive pharynx, the rostral aprt of the foregut). Stomach - development involved 1. Displacement - carries stomach from a position in the neck to the ventral to the caudal thoracic segments 2. Reorientation - involves rotation about two axes, the result of unequal growth of the stomach wall Facial processes - includes orbital, nasal and oral regions, affected by three events: 1. expansion of subpopulation of rostral neural crest 2. Cranial flexure 3. growth of prosencephalon and eyes and elaboration of the olfactory epithelium Stomodeum - cavity created by the formation of cranial and lateral body folds. Separated from the pharyngeal cavity by oropharyngeal membrane (oral plate or OPM) which breaks down following fusion of Land R mandibular process. Original site of OPM corresponds to palatoglossal arch. Prontonal Mesenchyme-forms the forehead and nasal region of the face External nares and epithelial lining of nasopharynx - becomes the olfactory (nasal) Placodes invaginates to form nasal pit - depends and come in contact with the roof of the stomodeum which is the oronasal membrane and soon degenerate. Palate not formed yet and forms a large oronasal cavity derived from stomodeum and nasal pit. Later ectodermal lining of the nasal pit will expand and form the olfactory epithelium Mouth - is formed by the ectoderm of stomodeum together with the tissue covering the palatine. Endoderm form the caudal part of the mouth and oropharynx 22 Developmental Anatomy Development of the face and nasal cavity. Series on the left shows ventral views of the facial processes; on the right are sagittal sections showing the invagination of the nasal (olfactory) placode to form the nasal pit. This contacts the stomodeum at the oronasal membrane, which degenerates to join embryonic nasal and oral cavities. Subsequent formation of the secondary palate (right, bottom) will extend the separation between these cavities caudally. Palatogenesis Following initial growth and fusion of facial mesenchymal population, Roof of the stomodeum bounded laterally maxillary process. Rostrally by medial nasal process and frontonasal prominence. At the roof are pairs of openings from nasal cavities. Mesenchymal cells Forms the medial nasal process and ventral frontal prominence These population of cells aggregate in the midline to form the medial palatine process and part of it become the primary palate and later forms the inçisive bone (premaxillary) Palatine fissure marks the caudal margin of the primary palate Mesenchyme located significantly between the nasal cavities contributes in the formation of rostral cartilage of the snout, philtrum and the upper lip Later, the secondary palate is formed which separates the nasal and oral cavities from the oropharynx. Hard palate -contains horizontal wings of maxillary and palatine bone. Soft palate no skeletal elements. Oronasal cavity is partially partitioned by two vertical tissue masses, the nasal septum projects from the roof of the cavity ventrally between the two nasal cavities. Lateral palatine processes grow into the oronasal cavity from the maxillary process or both sides and extend ventrally on either side of the tongue. Critical Events 1. Elevation and fusion of lateral palatine process. The change from vertical to horizontal plane occurred similar to the movement of a door upon a hinge. 2. Movement is rapid, and elevation of these palatine shelves is completed within a day. Mesenchyme in the rostral two-thirds undergo intramembranous ossification to form the hard palate. 23 Developmental Anatomy Lateral (left) and ventral (right) views of the formation of the formation of the face in a carnivore embryo. These represent stages when the embryo is between 6 and 10 mm in length (18-21 days in the cat, 22-26 days in the dog). DEVELOPMENT OF THE TEETH Requires epithelium and the underlying CT Starts at the dental arch of each jar Develop an epithelial ridge called dental lamina (growth into the underlying mesenchymal region) Epithelium proliferate and extend along the lateral border of the lamina into the mesenchyme Lateral extension arise the enamels of the deciduous teeth (milk teeth) Later period, medial or lingual outgrowths of the dental lamina give rise to enamel organs of the permanent teeth. Early development of a deciduous branchydont tooth. A. The surface epithelium proliferates and invaginates into the underlying mesenchyme as the dental lamina. B. The attached epithelial mass begins to cavitate adjacent to the mesenchymal condensation. C. The enamel organ forms during the bell stage. Enamel and dentine have begun to form. D. Eruption has occurred. Dentine and cementum will be added continually until complete eruption is achieved. 24 Developmental Anatomy PHARYNX Endodermally lined dorsoventrally, flattened tube from the oral plate to the esophagus Development differs from the rest of the endodermal tube. o Lie closely by the cephalic and lateral body fold, the heart and aortic arches underlie it ventrally o No coelom or cavity surrounding it o No separation of intraembryonic mesoderm o Becomes surrounded laterally and ventrally by mesenchyme derived from NCC, which will form the visceral arches or pharyngeal or branchial arches. Appendicular Tissues Sensitive to both genetic (intrinsic) and environmental (extrinsic) disturbance Relative autonomy of the developing limb Critical period is coincidental with the time at which processes are occurring Period of rabid growth during which muscles and skeletal tissues mature Limb should be functionally innervated and moving, otherwise ankylosis will occur. Embryology Initial even: Aggregation of lateral plate mesoderm at four discrete sites -> marks the formation of limb bud As bud enlarges, surface ectoderm thickens at the distal margin to become the apical ecotdermal ridge (AER) Bud rapidly elongates distally at the same time the mesenchyme within the bud forms two distinct population. 1. Proximally 1st to show signs of cytodifferentiation, forming chondrogenetic condensation 2. Distally - just beneath the AER, remain cytologically homogeneous. Source of cells from which antebranchial, tarsal/carpal and phalanges be develop. Bud flattened in the dorsoventral plante of embryo and the distal part acquires a paddle shape. Limb bends ventrally so that the original ventral surface becomes the medial surface and the formation of necrotic zones. Morphogenetic Fields Embryonic tissue which appear morphologically undifferentiated but committed to a particular fates. 1 Self-differentiation once removed and implanted to another site still forms a complete limb. 2. Embryonic regulation - ability of the tissue to recognize changes in their size or position and forms a complete, patterned set of appropriate structure. Ability to form a complete and normal structure is reestablished in what was previously a subpopulation of the orig tissue. 3. Polarity - rotates at 180° around both axis. Mechanisms: 1. Limb-forming mesenchyme is unique in its ability both to initiate the formation of an ectodermal AER and to provide for maintenance of the AER. 2. AER is essential for continued proximodistal outgrowth of the limb. Ectodermal thickening can initiate reorganization of the limb mesenchyme to produce duplications of distal tissue. 3. The proximodistal pattering of the limb is programmed within the distal cap and is independent of proximally located tissues. 4. Caudal limb mesenchyme can initiate a reorganization of adjacent lumb mesenchyme and subsequently, cause the formation of a new AER and development of a complete supernumerary limb. This region is called the zone of prolonging activity (ZPA). ANGIOGENESIS Process of blood vessel formation begins extraembryonically during or shortly after gastrulation Angiogenic cords appear within the lateral mesenchyme of the embryo which are composed of solid strands of splanchnic mesodermal cells. Cord elongates and grow into the head mesenchyme and then become patent. They form the aortic arches and dorsal aorta. 25 Developmental Anatomy BLOOD ISLAND Where the 1st blood cells are formed aggregates of splanchnic mesoderm on the surface of the yolk sac and the allantoin. Peripheral mesenchymal cells of the blood islands join together to form vesicles, the limiting of which will become vascular endothelium. Vesicles will coalesce to form vascular channels and subsequently form the vitelline and allantoic vessels. Central cells of blood island is the hemocytoblast and will differentiate into blood cells. When liver is develop, it assumed the function of blood cell production other tissues such as mesonephros and spleen as a minor hematopoietic sites. During the last trimester, hematopoiesis occurs primarily in the bone marrow. HEART Slightly curved tube located midventral beneath the caudal part of the pharynx Extending rostrally is a single vessel, the ventral aorta -- from which grow bilateral pairs of blood vessels called aortic arches - which carry blood dorsally around the pharynx to empty into dorsal aorta -- carry blood to the rest of the body. AORTIC ARCH TRANSFORMATION Heart (single tubular) - open cranially with paired ventral aorta and aortic arches and empty into the dorsal aorta fuse caudal to the heat Pair vitelline arteries- to the yolk sac Pair umbilical arteries - to the allantois branch from the dorsal aorta 5 pair of aortic arches - vertebrate have six but 5" A.A. is absent in birds and mammals Connects the ventral to the dorsal aorta Each arch passes through the mesenchyme of a visceral arch. 1 & 2 degenerate during the formation of caudal arches as 3, 4 and 6 develop - ventral aorta fuse to form a single ventral aortic root or aortic sac - which serves as a common chamber delivering blood to the arches. Process involved: 1. degeneration of certain vessels 2. differential growth of portion of Sequence of events: 1. A.A. 1 & 2 degenerate but rostral part of d. aorta retained and establish the internal carotid arteries 2. segment of d. aorta located between 3 and 4th A.A. degenerate. This leaves the 3rd A.A. as the major channels from heart to the head and 4th A.A. as the prin channels to the trunk. 26 Developmental Anatomy 3. pulmonary artery arise as a branch of 6th A.A. left of the segment becomes ductus arterius right degenerate. 4. series of cervical dorsal intersegmental aort. from roots. 5. R&L subclavian art, branch off to become cervical inter. 6. Brachiocephalic trunk arise the common carotid art. & R sub. Art. Paraxial mesoderm - located beside the neural tube each segment is called Somite → give rise to: 1) dermatome; 2) myotome; 3) sclerotome Contribute to the devt of all axial skeletal structural and voluntary muscles of the body Notochord - ventral to the neural tube is a mesodermal condensation. - dev't of neural tube and para, meso. Depends up the notochord, Lateral to the p. meso. Are cluster of epithelial ducto and tubules that will form nephrogenic and gonadal structures. Remaining mesoderm which extends around the gut and beneath the surface ectoderm to the ventral midline is lateral plate mesoderm: somatic (outer) - cavity between is coelom; splanchnic (inner) 27 Developmental Anatomy A, Schematic ventral view showing the later stages of aortic arch transformation. The asymmetric changes in the dorsal aortic roots and subclavian arteries are evident in B and C. Schematic left lateral view of the initial stages of transformation of the aortic arches and formation of the vertebral artery. 28 Developmental Anatomy Development of aortic arches and recurrent laryngeal nerve. A, lateral view of embryo Venous system - a pair of veins carry blood towards the heart from both the head and the trunk regions - these are cranial and caudal cardinal veins and empty into the heart via common cardinal veins. Vertebrate phase common feature: 1. dorsal hollow neural tube 2. somites which establish the segmental pattern of the embryo 3. ventral gut tube - forms the lining of tissues asstd. with respiration, digestion and splanchniomeso. This tube is enlarged cranially 4. coelom 5. heart Schematic right lateral view of a 24-somite dog embryo. The embryo is bent ventrally at the level of the mesencephalon (cranial flexure) and the first few somites (cervical flexure), and much of the gut is open ventrally. Note that the heart lies ventral to the hindbrain and pharynx at this stage, and that most abdominal and appendicular tissues are rudimentary or not yet present. The heart is the first embryonic organ to undergo functional differentiation. Shortly after the formation of a closed cephalic neural tube and during the period of formation of somites, the ventromedian tubular heart is formed and begins to contract rhythmically. This organ serves as a peristaltic pump, moving blood from extraembryonic vessels into and through the primitive embryonic circulatory system. Stages in the formation of the heart: 1. Formation of the cardiogenic plate. The cargiogenic plate is the splanchnic mesodermal primordium of the heart. 2. Formation of a single median heart tube. This stage is result of the cranial (subcephalic pocket formation) and internal (lateral body folds) folding of the embryo, bringing the left and right endocardial tubes together ventrally and medially 3. Formation of the cardiac loop. This stage is characterized by the bending of the heart tube such that the atrium which was originally located caudal to the ventricle is now located cranial to the ventricle. 29 Developmental Anatomy 4. Formation of the cardiac septa and valves. Septa separate the right pulmonary outflow from the left systemic outflow while values prevent the backflow of blood. The above stages occur without any interruption in the movement of blood. Formation of the Cardiogenic Plate and Endocardial Tube The cardiogenic plate is formed by splanchnic mesoderm ventral to the presumptive pleuropericardial cavity (coelom). Several vessels develop from loose aggregates of cardiogenic plate mesoderm and then coalesce to form the precursor of the endocardial tube. The mesoderm located cranial to the cardiogenic plate proliferates and thickens. This marks the initial formation of the septum transversum, which subsequently will form the central part of the diaphragm. The above events occur simultaneously with the process of head folding. During this folding all of the tissues located immediately in front of the neural tube are inverted 180º and brought beneath the cranial parts of the head. Thus, the primordium of the septum transversum becomes situated caudal to the cardiac tube and the pericardial cavity is located ventrally. The lateral body folding and the closure of the pharynx begin, bringing the right and left cardiac primordial together. This process craniocaudally forming the primordium of the truncus arteriosus first, then the bulboventricle, atrium and, lastly, the sinus venosus. The endocardial tube is surrounded by a thick layer of mesoderm called the myocardium, which later forms cardiac muscle Outside of this is a thin epicardial layer. The heart tube is suspended from the dorsal body wall by the fused spianchnic mesoderm called the dorsal mesocardium. The dorsal mosocardium will eventually degenerate along most of the heart tube except at the cranial (truncus arteriosus) and future caudal (sinus venosus) ends freeing the heart and allowing it to bend and loop Cardiac contractions and circulation begin at 35-38 hour of incubation in the chick 18-19 days of gestation in the dog 4th week of development in human Partitioning of the heart occurs at: 8-18 days in the chick 4'' week in dogs 6-7 weeks of gestation in human By the time embryo is 4-5 mm in length, the heart primordium is located ventral to the hindbrain and pharynx. Cardiac contractions and circulation begin at 35-38 hr of incubation in chick, 18-19 days of gestation in dog, late 4' week of human development The beat is initially slow, but increases as atrium and then sinus venosus form. Partitioning occurs 5-8 days in the chick, 4'" week in dog, between 5 and 7 weeks of gestation in human External Morphogenesis Shortly after its formation, the middle region of the tubular heart, the bulboventride, elongates and forms a C-shaped loop. This initially expands to the right side of the embryo. Internal Morphogenesis Internal partitioning of the heart and the cardiac outflow must occur in the atrium, the atrioventricular canal, the bulboventricular loop and the truncus arteriosus. Division of aortic sac into pulmonary and systemic compartment occur at the same time 30 Developmental Anatomy Development of the spiral septum. A, ventral view of the canine heart at 22-23 days of gestation (5- to 6-mm crown-rump length). Columns B and C illustrate the formation and fusion (3-4 days later) of