Gene Expression and Mutation PDF
Document Details
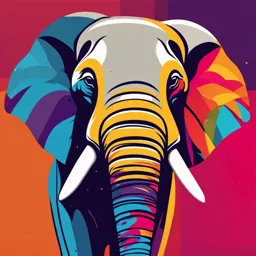
Uploaded by RecordSettingGalaxy
Tags
Summary
These are summary notes on important biological processes like gene expression and mutations. The document details the function, structure, and genetic code relating to these critical topics.
Full Transcript
Summary Notes: Gene Expression and Mutation Key Concepts 1. Gene Function: Genes influence traits by encoding proteins that perform biochemical, cellular, and organismal functions. 2. Central Dogma: DNA → RNA → Protein o Transcription: DNA is transcribed into RNA by RNA polym...
Summary Notes: Gene Expression and Mutation Key Concepts 1. Gene Function: Genes influence traits by encoding proteins that perform biochemical, cellular, and organismal functions. 2. Central Dogma: DNA → RNA → Protein o Transcription: DNA is transcribed into RNA by RNA polymerase. o Translation: RNA is translated into protein using ribosomes. 3. Non-Coding RNAs: Not all genes code for proteins (e.g., rRNA, tRNA); these RNAs have specialized functions. Gene Structure Genes include promoters, exons, and introns (in eukaryotes). Orientation is always 5' → 3'. Prokaryotes: o Lack introns. o Use simpler termination sequences for transcription. Eukaryotes: o Require RNA processing (splicing, capping, polyadenylation). o Use transcription factors for RNA polymerase binding. Genetic Code Codons: Triplets of nucleotides encode amino acids. Start Codon: AUG (Methionine). Stop Codons: UAA, UAG, UGA. Redundancy (i.e. “degeneracy): Multiple codons encode the same amino acid due to "wobble pairing." Mutations Types: 1. Silent: No change in protein. 2. Missense: Amino acid substitution (e.g., sickle cell disease). 3. Nonsense: Premature stop codon. 4. Frameshift: Alteration of the reading frame. Significance: Can have evolutionary impacts or cause diseases. Transcription in Eukaryotes 1. Initiation: Transcription factors recruit RNA polymerase to the promoter. 2. Elongation: RNA polymerase synthesizes RNA in 5' → 3'. 3. Termination: Cleavage occurs after the 3' UTR. RNA Processing Steps: 1. 5′ Capping: Stabilizes RNA; recognized by ribosomes. 2. Poly(A) Tail: Protects RNA from degradation. 3. Splicing: Removes introns; allows alternative splicing to create diverse proteins. Translation Stages: 1. Initiation: Ribosome assembles on mRNA at start codon. 2. Elongation: Amino acids added sequentially, reading the mRNA from 5’ to 3’ 3. Termination: Stop codon prompts release of the ribosome. Sites on Ribosome: o A site: Accepts new tRNA. o P site: Forms peptide bonds. o E site: Releases empty tRNA. Polysomes: Multiple ribosomes translating a single mRNA. Post-Translational Modifications Includes folding (chaperones), phosphorylation, glycosylation, lipidation. Modifications regulate protein function, localization, and stability. Protein Sorting Protein Sorting: The process by which proteins are directed to specific cellular locations where they perform their functions. This targeting is essential for maintaining cellular organization and function. Key Concepts in Protein Sorting 1. Sorting Signals: Specific sequences or structural features in proteins act as "postal codes." o Signal Peptides: Short amino acid sequences at the N-terminus that direct proteins to the endoplasmic reticulum (ER). o Nuclear Localization Signals (NLS): Short sequences that direct proteins to the nucleus. o Transit Peptides: Target proteins to organelles like mitochondria or chloroplasts. 2. Mechanism: o Proteins destined for secretion or the membrane are synthesized into the rough ER. o Other proteins are synthesized in the cytosol and sorted post-translationally. Sorting to the ER 1. Signal Recognition: o A signal recognition particle (SRP) binds the signal peptide on a nascent polypeptide. o SRP guides the ribosome to the SRP receptor on the ER membrane. 2. Translation into the ER: o The growing polypeptide chain is fed into the ER lumen. o Inside the ER: Signal peptidase removes the signal peptide. Folding and initial modifications (e.g., glycosylation) occur. 3. Targeting to Other Compartments: o Golgi Apparatus: Proteins are further modified and sorted for final destinations like the plasma membrane or lysosomes. Sorting to Other Organelles 1. Mitochondria and Chloroplasts: o Proteins have transit peptides that direct them to specific organelles. o Chaperones assist in translocation across organelle membranes. 2. Nucleus: o Proteins with nuclear localization signals pass through nuclear pores. o This process does not require full unfolding of the protein. 3. Lysosomes: o Proteins tagged with mannose-6-phosphate in the Golgi are sent to lysosomes. Post-Translational Modifications in Sorting 1. Phosphorylation: Activates or deactivates sorting signals. 2. Glycosylation: Helps in sorting within the ER and Golgi. 3. Lipidation: Anchors proteins to membranes. Importance of Protein Sorting Maintains cellular compartmentalization, ensuring that enzymes and other proteins function in the correct locations. Facilitates communication between organelles and the extracellular environment. Errors in protein sorting can lead to diseases, such as cystic fibrosis or lysosomal storage disorders. Key Discoveries in Heredity and DNA 1. Chromosomes and Heredity o Chromosomal theory of inheritance linked chromosomes to Mendel's principles (early 1900s). o Thomas Morgan Hunt demonstrated sex-linked traits, confirming chromosomal theory. 2. DNA as Genetic Material o Understand the below experiments: o Griffith's experiments (1920s): Identified the "transforming principle" in bacteria. o Avery’s work (1940s): Confirmed DNA as the "transforming principle." o Hershey-Chase experiment (1950s): Used radio-labeled DNA and proteins in bacteriophages to confirm DNA as the hereditary molecule. Structure of DNA 1. Key Contributions o Rosalind Franklin and Maurice Wilkins: X-ray diffraction revealed DNA's helical structure. o Watson and Crick (1953): Proposed the double helix model based on above x-ray data. 2. Chemical Composition o DNA is a polymer of nucleotides, each consisting of: ▪ A deoxyribose sugar. ▪ A phosphate group. ▪ A nitrogenous base (Adenine, Thymine, Guanine, Cytosine). o Nucleotides form a sugar-phosphate backbone with bases inside. 3. Key Structural Features o Strands are antiparallel (run in opposite directions). o Bases pair complementarily: A-T (2 hydrogen bonds), G-C (3 hydrogen bonds). o Chargaff’s rule provides first evidence for complementary base-pairing: Equal amounts of purines (A, G) and pyrimidines (T, C). DNA Replication 1. Semiconservative Replication o Watson and Crick's model proposed that each new DNA molecule contains one parental strand and one newly synthesized strand. o This was confirmed by Meselson and Stahl’s experiment using isotope-labeled DNA. Understand this experiment and what the three proposed replication mechanisms would have led to in the experimental results. 2. Replication Mechanism o Begins at origins of replication, forming replication bubbles. o Key terms: ▪ Leading strand: Synthesized continuously. ▪ Lagging strand: Synthesized in fragments (Okazaki fragments). o Bidirectional replication due to antiparallel strand orientation. 3. Replication Enzymes o Helicase: Unwinds DNA. o Single-stranded binding proteins (SSBs): Stabilize unwound DNA. o Topoisomerase: Prevents supercoiling ahead of the fork. o Primase: Synthesizes RNA primers. o DNA polymerase III: Extends the DNA strand from primers. o DNA polymerase I: Replaces RNA primers with DNA. o Ligase: Joins Okazaki fragments. 4. Replication Challenges o DNA polymerase adds nucleotides only to the 3' end, moving in the 5’ to 3’ direction as it adds bases. o The ends of linear DNA (telomeres) shorten with replication; telomerase extends telomeres in certain cells. Videos for Learning Multiple animations are recommended for visualizing DNA replication, including: o The replication machinery. o Meselson and Stahl experiment Summary for Chapter 10: Linkage and Sex-Linkage in Genetics 1. Gene Linkage Genes located on the same chromosome are linked and do not assort independently during meiosis. Key Terms: o Locus: The location of a gene on a chromosome. Linked genes result in deviations from Mendelian ratios (e.g., no 9:3:3:1 ratio in dihybrid crosses). Crossovers during meiosis can separate linked genes: o Genes farther apart on a chromosome are more likely to undergo crossovers, leading to recombinant gametes. o Genes closer together exhibit less recombination, meaning they are more tightly linked. Recombination Frequency: o Helps map the relative distances between genes (measured in centimorgans or map units). o 1% recombination = 1 map unit. 2. Test Crosses and Genetic Mapping Unlinked Genes: Testcross progeny segregate 1:1:1:1 (equal ratios). Linked Genes: Testcross progeny deviate from the expected 1:1:1:1 ratios. Recombination data from multiple crosses can help determine the gene order and distances on chromosomes. 3. Sex-Linkage Definition: Genes located on sex chromosomes (typically the X chromosome). Human Sex Determination: o Females: XX; Males: XY. X-Linked Inheritance: o Traits associated with X-linked recessive genes (e.g., colorblindness, hemophilia) are more common in males because they have only one X chromosome. X-Inactivation: o In females, one X chromosome is randomly inactivated to ensure dosage compensation. o This phenomenon leads to traits like calico coat patterns in cats, where different X chromosomes are active in different cells. 4. Human Genetics Pedigree Analysis: o A tool for studying inheritance patterns of genes in humans. Modes of Inheritance: o Autosomal Recessive/Dominant: Traits carried on non-sex chromosomes. o X-Linked Recessive/Dominant: Traits carried on the X chromosome. Basics Meiosis and Life Cycle: Explains how homologous chromosomes and alleles play a role in inheritance, with meiosis creating genetic diversity. Alleles: Different versions of a gene, inherited from each parent, can lead to varied protein functions. Dominance and Recessive Alleles: The document uses pea seed coloration as an example. Functional enzymes result in dominant traits (e.g., yellow seeds), while non- functional enzymes lead to recessive traits (e.g., green seeds). Mendelian Laws 1. Law of Dominance: One allele can mask the expression of another. 2. Law of Segregation: Alleles separate during gamete formation, ensuring offspring receive one allele from each parent. 3. Law of Independent Assortment: Genes for different traits are inherited independently if located on separate chromosomes. Crosses and Ratios Monohybrid Crosses: Illustrates a 3:1 phenotypic and 1:2:1 genotypic ratio in F2 generations. Dihybrid Crosses: Demonstrates a 9:3:3:1 phenotypic ratio when analyzing two traits (e.g., seed color and texture). Punnett squares are used for predicting offspring ratios and test crosses for determining unknown genotypes. Test crosses: A testcross is a cross between an individual with the dominant phenotype and a homozygous recessive individual. Used to determine whether an individual with a dominant trait is a heterozygote or homozygote. Extensions to Mendelian Genetics 1. Incomplete Dominance: Results in an intermediate phenotype (e.g., pink flowers from red and white parents). 2. Codominance: Both alleles are expressed equally (e.g., spotted patterns in animals, ABO blood groups). 3. Polygenic Traits: Traits influenced by multiple genes, leading to a continuous range of phenotypes (e.g., human height). 4. Epistasis: Interaction between genes where one gene's activity depends on another's (e.g., coat color in dogs). 5. Pleiotropy: A single gene influencing multiple traits (e.g., clasp-1 mutants in plants causing varied developmental issues). Example of genetic testcross: In tomato plants, red fruit color (R) is dominant to yellow fruit color (r), and tall plant height (T) is dominant to dwarf plant height (t). A plant that is heterozygous for both traits is crossed with a plant that is homozygous recessive for both traits. What proportion of the offspring will be tall with yellow fruits? A) 1/4 B) 1/2 C) 3/4 D) 1/8 This is a dihybrid cross problem where we have two traits: fruit color and plant height. Key Information Traits: o Red (R) is dominant, yellow (r) is recessive. o Tall (T) is dominant, dwarf (t) is recessive. Cross: o Heterozygous for both traits: RrTt o Homozygous recessive for both traits: rrtt Steps to Solve 1. Determine Gametes: o Heterozygous plant (RrTt) produces four types of gametes: RT,Rt,rT,rt. o Homozygous recessive plant (rrtt) produces one type of gamete: rt. 2. Punnett Square: Set up a 4x1 Punnett square to combine the gametes: 3. Phenotypes: o RrTt: red, tall. o Rrtt: red, dwarf. o rrTt: yellow, tall. o rrtt: yellow, dwarf. 4. Calculate Proportion: o Total possible outcomes: 4. o Offspring that have yellow fruits and are tall (rrTt): 1 out of 4. Answer: The proportion of offspring that have yellow fruits and are tall is 1/4. Summary Notes on Chapter 8 – Genetic Recombination (meiosis) Key Concepts Sexual Reproduction: o Produces genetic variation, which is essential for evolution and adaptation to environmental changes. o Genetic diversity arises through meiosis. Purpose of Meiosis: o Generates genetic variability by shuffling DNA. o Produces haploid gametes (egg and sperm), each containing one copy of every chromosome. Comparison: Meiosis vs. Mitosis Feature Mitosis Meiosis Growth and restoration of Purpose Formation of gametes somatic tissues Two genetically identical Outcome Four genetically unique haploid cells diploid cells Chromosomal No pairing of homologous Homologous chromosomes pair, recombine, Pairing chromosomes and separate during Meiosis I Stages Single division Two divisions (Meiosis I and Meiosis II) High (crossing over, independent assortment, Genetic Diversity None and random fertilization) Meiosis Stages 1. Meiosis I: o Prophase I: Homologous chromosomes pair (synapsis), forming tetrads. Crossing over (exchange of genetic material) occurs at chiasmata. o Metaphase I: Homologous pairs align at the metaphase plate. o Anaphase I: Homologs separate, reducing chromosome number by half. o Telophase I and Cytokinesis: Two haploid cells are formed, each with one set of chromosomes (1n). 2. Meiosis II (Similar to Mitosis): o Prophase II: Chromosomes condense again in haploid cells. o Metaphase II: Sister chromatids align at the metaphase plate. o Anaphase II: Sister chromatids separate. o Telophase II and Cytokinesis: Four haploid (1n) gametes are produced. Sources of Genetic Variation 1. Crossing Over (Prophase I): o Homologous chromosomes exchange genetic material, creating new allele combinations. 2. Independent Assortment (Metaphase I): o Random orientation of homologous pairs at the metaphase plate results in 2n possible combinations (e.g., 223 = ~8.4 million in humans). 3. Independent Assortment (Metaphase II): o Random orientation of sister chromatids at the metaphase plate again results in 2n possible combinations (e.g. 8.4 million x 8.4 million = over 70 trillion in humans) 4. Random Fertilization: o Any sperm can fuse with any egg, further increasing genetic variability. Life Cycles in Organisms Animals: Gametic meiosis produces gametes directly, which fuse during fertilization to form a diploid zygote. Plants: Alternation of generations: o Sporic meiosis: Sporophyte (diploid) undergoes meiosis to produce four haploid spores instead of gametes (sperm/egg). o Spores grow into a gametophyte (haploid), which produces gametes via mitosis. Fungi and Algae: Zygotic meiosis o Upon fertilization, the zygote undergoes meiosis directly, producing spores instead of gametes. Spores germinate to form haploid organisms that upon maturity undergo mitosis to make their gametes (since they are already haploid, so no need to do meiosis again). 1. Cell Cycle Overview: o The cell cycle is the life cycle of a cell, involving growth, DNA replication, and division. o Prokaryotes divide through binary fission, while eukaryotes undergo mitosis. 2. Stages of the Eukaryotic Cell Cycle: o Interphase: ▪ G1 phase: Cell grows and prepares for DNA synthesis. ▪ S phase: DNA is replicated. ▪ G2 phase: Cell prepares for mitosis. o G0 phase: Cells can exit the cycle and enter a state of terminal differentiation or re-enter the cycle. 3. Prokaryotic Cell Cycle: o Prokaryotes, which have a single circular chromosome, undergo binary fission. o DNA replication and cell division occur rapidly, with minimal separation of stages. 4. Mitosis: o Mitosis is the division of eukaryotic cells and consists of several phases: ▪ Prophase: Chromosomes condense, nuclear envelope begins breaking down. ▪ Prometaphase: Nuclear envelope breaks down and microtubules attach to chromosomal kinetochores and move them toward the middle of the spindle. ▪ Metaphase: Chromosomes align at the metaphase plate. ▪ Anaphase: Sister chromatids separate and move to opposite poles. ▪ Telophase: Chromosomes decondense, nuclear envelope reforms, spindle microtubules are lost. o Cytokinesis: Cytoplasm divides, producing two daughter cells. Typically overlaps to varying degrees with anaphase and telophase. 5. Chromosome Structure: o During replication, a chromosome forms two sister chromatids connected by a centromere. o Chromatin condenses into visible chromosomes during mitosis. o The mitotic spindle, composed of microtubules, organizes and moves chromosomes. 6. Microtubules and Spindle Assembly: o Microtubules play a critical role in chromosome movement during mitosis. o Kinetochore microtubules attach to chromosomes, while non-kinetochore and astral microtubules help in spindle positioning and maintaining spindle integrity. 7. Anaphase Mechanisms: o In Anaphase A, kinetochores shorten microtubules, pulling chromatids to poles. o In Anaphase B, non-kinetochore microtubules elongate the spindle. Overview of Photosynthesis Definition: Conversion of CO2 and water into sugars using light energy and releasing O2 as a byproduct. Location: Takes place primarily in the chloroplasts of mesophyll cells, utilizing the thylakoid membranes and stroma. Photosynthesis: Key Stages 1. Light-Dependent Reactions: o Occur in the thylakoid membranes. o Capture light to produce ATP and NADPH via an electron transport chain. o Water is oxidized, releasing O2. o Involves chemiosmosis to generate ATP (photophosphorylation). 2. Calvin Cycle (Light-Independent Reactions): o Occurs in the stroma. o Fixes CO2 into sugars using ATP and NADPH from the light reactions. o Key steps: ▪ Fixation: CO2 is attached to a 5-carbon molecule (RuBP) by Rubisco, producing two 3-carbon molecules. ▪ Reduction: ATP and NADPH convert these into G3P (which is used to form sugars). ▪ Regeneration: Some G3P molecules regenerate RuBP for the cycle to continue. Key Components Chlorophyll: o Absorbs light in the blue and red wavelengths; while green wavelengths pass through it (are transmitted), or reflected (bounce off it). This is why it looks green. o Combines with accessory pigments to broaden light absorption. Photosystems: o Photosystem II (PSII): Oxidizes water to supply electrons, generating O2. o Photosystem I (PSI): Receives electrons from ETC and boosts them to high energy with light, allowing them to reduce NADP+ to NADPH. o Antenna complexes funnel light energy toward reaction centers. Electron Transport Chain: o Establishes a proton gradient (i.e. proton motive force/electrochemical gradient) across the thylakoid membrane. o Protons flow through ATP synthase, creating ATP (i.e. chemiosmosis). Cyclic Electron Transport: o Generates ATP but not NADPH. o Electrons do not end up in NADPH, but are instead recycled to earlier in the ETC o Helps balance the energy requirements of the Calvin Cycle. Energy Flow in Photosynthesis Inputs: Light, H2O, CO2. Outputs: O2, ATP, NADPH, sugars (e.g., glucose). Challenges in Photosynthesis Photorespiration: o Occurs when Rubisco fixes O2 instead of CO2, leading to energy loss. o Plants in dry climates combat this by concentrating CO2 around RUBISCO so it outcompetes O2: ▪ Spatial concentration (concentrate CO2 in specialized cells in the leaf). ▪ Temporal concentration (concentrate CO2 at night and fix during day). Overview of Cellular Respiration Cellular respiration involves breaking down food molecules (e.g., glucose) to produce ATP, a process that includes catabolic reactions. The key equation: C6H12O6 + 6O2 → 6CO2 + 6H2O + Chemical Energy (ATP) Energy Flow and Bioenergetics Energy originates from the Sun, captured via photosynthesis, and stored in sugars. Energy in glucose is harnessed by transferring electrons through a series of redox reactions, powering ATP synthesis. Stages of Cellular Respiration 1. Glycolysis (Occurs in the cytosol): o Converts glucose (6C) into two pyruvate molecules (3C each). o Yields 2 ATP (net) and 2 NADH. o Anaerobic process (can occur in the absence of oxygen). 2. Pyruvate Oxidation and Citric Acid Cycle (Kreb’s Cycle) (occur in matrix): o Pyruvate is transported into the mitochondrial matrix and converted into Acetyl- CoA. o Acetyl-CoA enters the citric acid cycle, producing NADH, FADH2, ATP, and CO2. 3. Oxidative Phosphorylation: o Involves the electron transport chain (ETC) and chemiosmosis. o High-energy electrons from NADH and FADH2 are transferred through the ETC, creating an electrochemical gradient (proton motive force) that powers ATP synthase. o Chemiosmosis refers to the movement of H+ down their concentration gradient through ATP synthase to power ATP production. o O2 is the terminal electron acceptor, becoming reduced to H2O. ATP Production Two mechanisms: 1. Substrate-level phosphorylation: Direct transfer of phosphate to ADP. 2. Oxidative phosphorylation: ATP synthase uses energy from the proton gradient. Most ATP is produced during oxidative phosphorylation. Electron Transport Chain (ETC) The ETC is involved in the final stage of cellular respiration, oxidative phosphorylation, occurring in the inner mitochondrial membrane (or the plasma membrane in prokaryotes). It comprises a series of protein complexes and mobile electron carriers. Flow of Electrons 1. Electron Sources: o Electrons are harvested from glucose during glycolysis, pyruvate oxidation, and the citric acid cycle. o These electrons are carried to the ETC by NADH and FADH2, which are oxidized back to NAD+ and FAD as they donate their high-energy electrons. o The oxidized NAD+ and FAD molecules are recycled back to glycolysis and the citric acid cycle to get reduced and repeat the cycle. 2. Redox Reactions: o Electrons move through a chain of protein complexes (Complexes I, II, III, IV) via a series of redox reactions. o Each successive complex in the ETC has a higher affinity for electrons (stronger oxidizing power) than the previous one, pulling electrons down the chain like an electrical current. 3. Electron Energy Levels: o As electrons move down the ETC, they lose free energy in each transfer. This energy is not wasted—it is used to pump protons (H+) across the inner mitochondrial membrane, creating an electrochemical gradient. o Electrons start at high energy (when associated with NADH or FADH2) and end at a very low energy level when they are passed to the terminal electron acceptor, oxygen. 4. Oxygen's Role: o At the end of the ETC, oxygen, the strongest oxidizing agent in the chain, accepts electrons and combines with H+ ions to form water (H2O). Without oxygen, the chain cannot operate, halting ATP production. Proton Gradient (Proton Motive Force) The energy released as electrons flow is used by Complexes I, III, and IV to pump H+ ions from the mitochondrial matrix into the intermembrane space. This creates a proton motive force, an electrochemical gradient with: o Chemical Potential: High concentration of H+ in the intermembrane space. o Electrical Potential: Positive charge in the intermembrane space relative to the matrix. ATP Synthesis via Chemiosmosis Protons flow back into the matrix through ATP synthase, a turbine-like enzyme. The flow of H+ drives the rotation of ATP synthase, which catalyzes the phosphorylation of ADP to ATP. Energy Efficiency Electrons from NADH enter the chain at Complex I, contributing more energy to proton pumping than electrons from FADH2, which enter at Complex II. The gradual energy drop of electrons in the ETC ensures efficient energy capture without excessive heat loss. Key Molecules in Respiration Electron carriers: NADH and FADH2 shuttle high-energy electrons. ATP Synthase: Uses free energy released from the passive flow of H+ ions (protons) down their concentration gradient to generate ATP. Regulation of Respiration Feedback inhibition: Products of the pathway inhibit upstream enzymes, ensuring regulation based on cellular energy needs. Fermentation Alternative pathway when oxygen is unavailable: o Lactate fermentation: Produces lactic acid (in animals and some bacteria). o Alcoholic fermentation: Produces ethanol and CO2 (in yeast). The reason fermentation exists is to recycle NAD+ molecules, because in the absence of oxygen (the terminal electron acceptor), NADH can’t donate its electrons to the ETC, and thus accumulates. Because of this, the NAD+ molecules needed for glycolysis and the citric acid cycle become depleted. In the absence of NAD+, the reactions during glycolysis and citric acid cycle can no longer proceed, and the end result is no ATP production. Respiratory Variations Across Organisms Prokaryotes: Respiration occurs in the cytoplasm and plasma membrane. Strict aerobes, strict anaerobes, and facultative anaerobes adapt respiration based on oxygen availability. Additional Concepts Reactive Oxygen Species (ROS): Byproducts of aerobic respiration that can damage cells; managed by antioxidant systems like enzymes (e.g., catalase). Some anaerobic prokaryotes are able to use oxidative phosphorylation because they use molecules other than O2 as the terminal electron acceptor. Summary Notes for Introductory Biology Lecture: Origins of Life 1. Chemical Origins of Life o Panspermia Hypothesis: Suggests life on Earth might have originated from extraterrestrial sources, such as meteorites containing organic molecules. o Abiogenesis: Life arose from non-living matter under early Earth's reducing conditions. ▪ Atmosphere Composition: Hydrogen, CO₂, NH₃, CH₄, H₂O; minimal O₂, high UV radiation. ▪ Oparin-Haldane Hypothesis: Reducing atmosphere facilitated formation of complex organic molecules. ▪ Miller-Urey Experiment: Demonstrated formation of amino acids and organic molecules under simulated early Earth conditions. o Mineral Surface Polymerization: Clay acted as a catalytic surface for polymer and liposome formation. Polymers get trapped in liposomes, become protocells. 2. RNA World Hypothesis o RNA was likely the first molecule for storing genetic information and catalyzing reactions, including its own replication. o Evidence includes ribozymes, RNA enzymes that catalyze reactions. o Transition to DNA and protein: DNA became the stable genetic material, while proteins took over catalytic functions due to their versatility. 3. First Metabolic Processes o Likely occurred in environments such as deep-sea alkaline vents. ▪ Gradients of H⁺ ions (proton gradients) powered early chemical reactions, a mechanism still used in modern photosynthesis and respiration. ▪ Iron-sulfur clusters, abundant in vents, were likely precursors to enzymes involved in energy metabolism, and are in fact still present as cofactors for many modern enzymes. 4. Development of Cellular Life o Protocells formed with lipid bilayers and basic metabolic functions. o The Last Universal Common Ancestor (LUCA): ▪ Anaerobic metabolism using H₂, CO₂, and N₂. ▪ Thermophilic, thriving in high-temperature environments. ▪ Shared characteristics of modern cells: lipid bilayer, ribosomes, DNA→RNA→Protein information storage/retrieval system. 5. Evolution of Photosynthesis o Early phototrophs used sunlight to produce energy, eventually leading to oxygenic photosynthesis by cyanobacteria. o The Great Oxygenation Event (GOE): ▪ Oxygen accumulation due to photosynthesis caused mass extinctions of anaerobic organisms. ▪ Created an environment for the evolution of aerobic respiration and eukaryotes. 6. Eukaryotic Evolution o Originated via endosymbiosis: ▪ Aerobic bacteria became mitochondria. ▪ Photosynthetic bacteria became chloroplasts. o Horizontal gene transfer (from the endosymbionts) and endomembrane development played critical roles. Their genomes were gradually outsourced to the host’s nuclear genome so that they could focus all of their efforts on energy production. 7. Evidence of Early Life o Earliest fossils: Stromatolites (~3.5 billion years ago). o Radiocarbon evidence: Early life dates back to ~3.9 billion years ago. o Geological indicators: Rusted (oxidized) iron in geological strata (~2.5 billion years ago) signify oxygen accumulation. Lecture 2 (macromolecules, cell theory, microscopy) 1. Molecules of life Water: The solvent of life, dissolving polar and charged molecules due to its polarity. Types of Macromolecules: o Carbohydrates: Polymers of sugars (e.g., glucose). o Lipids: Not true polymers but hydrophobic molecules (e.g., fats, oils). o Proteins: Polymers of amino acids; their structure is determined by the sequence and properties of amino acids. o Nucleic Acids: Polymers of nucleotides (e.g., DNA, RNA). Polymerization: The process of linking monomers together (dehydration synthesis), while depolymerization (hydrolysis) involves breaking down polymers by adding water. Protein Structure: o Primary: Linear sequence of amino acids. o Secondary: Formation of α-helices and β-sheets through hydrogen bonding. o Tertiary: Folding of the polypeptide into a 3D structure determined by chemistry of amino acid sidechains. o Quaternary: Interaction of multiple proteins to form a complex. 2. Cell Theory Core Principles: o All living organisms are composed of one or more cells. o The cell is the basic unit of structure and function in living organisms. o All cells arise from the division of pre-existing cells. 3. Microscopy Types: o Light Microscopy: Uses light to view cells and tissues. Variants include: ▪ Brightfield: Direct light transmission, often requires staining for better contrast. ▪ Darkfield: Illuminates the sample from the side, so that only light that is scattered by the specimen reaches the objective lens. ▪ Phase-Contrast: Enhances contrast by shifting light phases. ▪ Differential Interference Contrast (DIC): Enhances contrast and gives a pseudo-3D appearance to specimens. o Electron Microscopy: ▪ Transmission Electron Microscopy (TEM): Provides detailed internal views of thinly sliced samples. ▪ Scanning Electron Microscopy (SEM): Produces detailed 3D images of surfaces. Concepts: o Resolution: The ability to distinguish two objects as separate entities. o Magnification: increasing the apparent size of the specimen, as a lens does. Higher magnification increases resolution. o Contrast: Enhances the visibility of structures within the sample. Does not increase resolution. Lecture 3 (microscopy, Prokaryotes vs. Eukaryotes) 1. Microscopy Types of Microscopy: o Light Microscopy: Includes techniques such as brightfield, darkfield, phase- contrast, and Differential Interference Contrast (DIC), which enhance contrast by exploiting light-scattering properties. o Fluorescence Microscopy: Utilizes fluorophores to visualize specific structures by emitting light when excited by photons. This method allows observation of live specimens and specific cellular components. o Electron Microscopy (EM): Provides higher resolution due to the shorter wavelength of electrons. Includes: ▪ Transmission Electron Microscopy (TEM): Thin sections of specimens are imaged, creating 2D images with high detail. ▪ Scanning Electron Microscopy (SEM): Visualizes 3D surface contours of specimens, typically at lower magnifications. Key Concepts: o Fluorescence: Occurs when an electron absorbs light, moves to a higher energy state, and emits light at a longer wavelength. o Advantages of Fluorescence Microscopy: Visualization of specific cellular structures, ability to observe live cells, and applications in imaging molecules like proteins and actin filaments. o Resolution in Microscopy: Electron microscopes provide much higher resolution than light microscopes but require fixed (dead) specimens. 2. Prokaryotes vs. Eukaryotes Prokaryotes: o Typically small (1-3 µm), unicellular organisms such as bacteria and archaea. o Have a nucleoid instead of a nucleus and contain a single circular chromosome. o Lack membrane-bound organelles. Eukaryotes: o Larger cells (10-100 µm), which include animals, plants, fungi, and protists. o Contain membrane-bound organelles, including a nucleus that houses linear chromosomes. o Can be unicellular or multicellular. Similarities: Both prokaryotes and eukaryotes share fundamental features like a plasma membrane, cytoplasm, ribosomes, and a cytoskeleton. Lecture 4 (Prokaryotes vs. Eukaryotes, Methods, and Organelles) 1. Prokaryotes vs. Eukaryotes Shared Features: Both cell types have essential components like DNA (genetic material), ribosomes for protein synthesis, a plasma membrane, and cytoplasm. Differences: Prokaryotic cells lack a true nucleus and membrane-bound organelles, while eukaryotic cells have a nucleus, mitochondria, and other organelles. 2. Cellular Organelles and Structures Plasma Membrane: Composed of a phospholipid bilayer with embedded proteins, regulating the entry and exit of substances. Cytoplasm: Contains the cytosol (liquid component) and organelles where many metabolic reactions occur. Ribosomes: Complexes of proteins and RNA that synthesize proteins from mRNA; prokaryotic ribosomes (70S) are smaller than eukaryotic ribosomes (80S). Cytoskeleton: A network of filaments in the cytoplasm responsible for maintaining cell shape, aiding in cell division, and transporting substances within the cell. 3. Research Methods Differential Centrifugation: A technique used to separate cell components based on size and density. Involves spinning samples at different speeds to isolate organelles of interest (e.g. nuclei, mitochondria). Green Fluorescent Protein (GFP): A protein derived from jellyfish that emits green light when exposed to blue light. GFP is widely used in biology to visualize cellular structures, processes, and dynamics in living specimens. LECTURE 5 (nucleus, endomembrane) 1. The Nucleus Structure: o Surrounded by a double membrane called the nuclear envelope, which contains nuclear pores to regulate the entry and exit of molecules, which is gated by the nuclear pore complex. o Chromatin: DNA and associated proteins within the nucleus. o Nucleolus: A region responsible for assembling ribosomal subunits. o Nucleoplasm: The "cytoplasm" within the nucleus. Function: o Stores genetic material (DNA) and coordinates activities such as growth, metabolism, and protein synthesis. 2. The Endomembrane System A network of membranes that compartmentalizes the cell and regulates the movement of molecules within cells. Components: o Nuclear Envelope: Continuous with the rough endoplasmic reticulum. o Endoplasmic Reticulum (ER): ▪ Rough ER: Studded with ribosomes; involved in protein synthesis, folding, and modification. ▪ Smooth ER: Lacks ribosomes; involved in lipid synthesis and detoxification. o Golgi Apparatus: Modifies proteins received from the ER, adds finishing touches like sugars, and sorts them for delivery. o Vesicles: Transport materials between different parts of the endomembrane system, such as from the ER to the Golgi. o Lysosomes: Contain digestive enzymes to break down waste materials and cellular debris. o Plasma Membrane: Regulates what enters and exits the cell. Concepts: o Compartmentalization: Different metabolic processes can occur in specific, controlled environments within the cell. o Membrane Trafficking: Movement of proteins and other molecules through vesicles between organelles. o Protein Processing: Proteins are synthesized in the ER, modified in the Golgi, and transported to their final destinations. 3. Vesicle Trafficking Process: o Exocytosis: Vesicles move materials from the ER to the Golgi and then to the plasma membrane to be secreted from the cell. o Endocytosis: The plasma membrane engulfs external materials and brings them into the cell in vesicles. Example: Neurotransmitter release (exocytosis) and viral entry into cells (endocytosis). Lecture 6 (endomembrane, semi-autonomous, cytoskeleton) 1. Endomembrane System Components: o Nuclear Envelope: Controls what enters and exits the nucleus. o Endoplasmic Reticulum (ER): ▪ Rough ER: Protein synthesis and folding. ▪ Smooth ER: Lipid synthesis and detoxification. o Golgi Apparatus: Modifies proteins and sorts them into vesicles for transport. o Vesicles: Transport materials between cellular compartments. o Lysosomes: Digestive organelles that break down waste and engulfed materials. o Vacuoles: Large structures in plants and fungi that regulate turgor pressure and store nutrients. o Plasma Membrane: Regulates what enters and leaves the cell. 2. Semi-Autonomous Organelles Mitochondria and Chloroplasts: o Generate energy (ATP in mitochondria, sugars in chloroplasts). o Mitochondria are involved in respiration, and chloroplasts perform photosynthesis. o Both organelles have their own DNA and ribosomes, replicate independently, and have double membranes. o They originated from ancient prokaryotic symbionts. 3. Cytoskeleton Components: o Microtubules: Polymers of tubulin that maintain cell shape, aid in cell division, and serve as tracks for organelle movement. o Microfilaments: Polymers of actin that support organelle movement, cell movement, division, and structural support. o Intermediate Filaments: Polymers of intermediate filament proteins; found in some eukaryotes like animals but not in plants or fungi. Functions: Maintain cell shape, provide mechanical support, cell adhesion. Lecture 7 (cytoskeleton, ECM) 1. Cytoskeleton Components: o Intermediate Filaments: Provide structural support and adhesion. Found in animal cells, they are made of proteins like keratin and collagen. Plants and fungi lack intermediate filaments, relying on cell walls for structural support. o Microtubules: Composed of tubulin, these structures are involved in maintaining cell shape, enabling intracellular transport, and facilitating cell division. Motor proteins kinesins and dyneins move along microtubules, carrying vesicles and organelles. o Microfilaments: Made of actin, microfilaments support cell shape, movement, and division. Myosin motor proteins walk along microfilaments, playing a role in muscle contraction and cellular migration. Functions: o Cell shape and polarity: Microtubules and microfilaments help maintain cell shape. o Cell division: Microtubules organize chromosomes during mitosis, while microfilaments aid in cytokinesis. o Intracellular transport: Motor proteins use the cytoskeleton to transport organelles and vesicles. o Movement: Cilia and flagella, driven by microtubules and dynein, facilitate cell movement or fluid movement over cell surfaces. 2. Extracellular Matrix (ECM) Components: o Animals: ECM is composed primarily of proteins (e.g., collagen) and glycoproteins. It provides structural support, cell adhesion, cell shape. o Plants, Fungi, Bacteria: The ECM takes the form of cell walls. Plant cell walls are primarily composed of cellulose, fungi use chitin, and bacteria have peptidoglycan. ECM Functions: o Support: Provides structural integrity to tissues and organs. o Cell adhesion: Holds cells together or attaches them to surfaces. o Protection: Acts as a barrier against physical damage and pathogens. o Intercellular communication: Allows communication between cells and facilitates selective transport through structures like plasmodesmata (plants) and gap junctions (animals). Key Concepts in Bioenergetics Bioenergetics: The study of energy flow through biological systems. o Metabolism: Enzymatically-driven processes. Can be divided into: ▪ Anabolism: Building complex molecules (e.g., photosynthesis, lipid synthesis). ▪ Catabolism: Breaking down molecules for energy (e.g., glycolysis, cellular respiration). o Energy Types: ▪ Potential Energy: Stored energy (e.g., chemical bonds). ▪ Kinetic Energy: Energy of motion (e.g., molecular movement). Thermodynamics in Biology 1. First Law of Thermodynamics: o Energy cannot be created or destroyed but can transform or transfer. o Biological systems are open systems, exchanging energy and matter with their surroundings. 2. Second Law of Thermodynamics: o Systems naturally move towards disorder (increased entropy). o Biological systems maintain order but increase entropy in their surroundings due to heat loss. Free Energy and Metabolic Processes Gibbs Free Energy (ΔG): o Measures the energy available to do work. o Exergonic Processes: ▪ Release energy (negative ΔG). ▪ Examples: Diffusion, ATP hydrolysis. o Endergonic Processes: ▪ Require energy input (positive ΔG). ▪ Examples: Active transport, biosynthesis. Energy Coupling: o Combines exergonic reactions (e.g., ATP hydrolysis) with endergonic reactions to drive biological processes. Enzymes: Catalysts of Life Role: Lower the activation energy of a reaction to speed it up. Mechanisms: o Stabilize substrates in the transition state o Examples: ▪ Bring substrates together in correct orientations. ▪ Induce strain in specific bonds to facilitate breaking. ▪ Create a distribution of electrical charges in the active site Models of Enzyme Function: 1. Lock-and-Key: o The enzyme's active site has a fixed shape complementary to the substrate. Old, not accurate. 2. Induced Fit: o The enzyme and substrate undergo conformational changes for a better fit at the active site. Factors Influencing Enzyme Activity: 1. Temperature: o Increases activity up to an optimal point before denaturation occurs. 2. pH: o Enzymes function best at their environmental pH optima. 3. Concentrations: o Higher substrate/enzyme levels increase reaction rates until saturation. 4. Regulators: o Competitive Inhibitors: Compete for the active site. o Non-competitive Inhibitors: Bind elsewhere, altering enzyme function. ATP and Energy in Cells ATP (Adenosine Triphosphate): o Universal energy currency regenerated from ADP + Pi. o Hydrolysis releases energy (-7.3 kcal/mol) used in cellular work. Lecture 8: Membrane Structure and Function 1. Membrane Structure and Composition: o Phospholipid bilayer forms the basic structure, with hydrophilic heads facing water and hydrophobic tails avoiding water. o Membrane asymmetry: The outer and inner leaflets differ in lipid, protein, and carbohydrate composition. o Membranes are fluid, described by the "fluid mosaic model," allowing flexibility and the proper function of embedded proteins. 2. Membrane Fluidity: o Factors influencing fluidity: temperature, phospholipid composition (degree of unsaturation, tail length), and cholesterol. o Cholesterol acts as a "fluidity buffer," maintaining stability under temperature fluctuations. 3. Membrane Proteins: o Integral and peripheral proteins serve various roles such as transporters, enzymes, signal receoptors, and cell recognition. o Integral membrane proteins contain stretches of non-polar (and thus hydrophobic) amino acids that span the membrane. These are referred to as transmembrane domains. 4. Membrane Transport: o Passive transport requires no energy and includes simple diffusion and facilitated diffusion. o Active transport requires energy (ATP) to move molecules against concentration gradients. Lecture 9: Membrane Transport 1. Types of Membrane Transport: o Passive Transport: Movement down concentration gradients (high to low); includes diffusion and facilitated diffusion. o Facilitated Diffusion: Requires transport proteins (channels, gated channels, carrier proteins) to help molecules cross the membrane. o Active Transport: Moves molecules against gradients using energy; two types: ▪ Primary Active Transport: Uses ATP (e.g., sodium-potassium pump). ▪ Secondary Active Transport: Uses energy from electrochemical gradients (e.g., sodium-glucose co-transporter). 2. Endocytosis and Exocytosis: o Endocytosis: Uptake of substances via vesicles (includes receptor-mediated endocytosis, pinocytosis, phagocytosis). o Exocytosis: Release of substances from cells through vesicle fusion with the membrane. Lecture 10: Osmosis and Active Transport 1. Osmosis: o The movement of water across a semi-permeable membrane toward regions of higher solute concentration. o Cells can shrink (hypertonic), swell (hypotonic), or remain stable (isotonic) based on the surrounding solution. 2. Active Transport Examples: o Primary Active Transport: Proton pumps and sodium-potassium pumps maintain concentration gradients by using ATP. o Secondary Active Transport: Utilizes ion gradients (e.g., sodium-glucose cotransport). 3. Signal Transduction: o Key roles of transporters, enzymes, and receptor proteins in transmitting signals across membranes. o Signaling pathways often involve phosphorylation cascades, amplifying very faint extracellular signals (e.g., hormones). Lecture 11: Signal Transduction and Energy Storage 1. Energy in Gradients: o Concentration gradients across membranes store potential energy, used to drive cellular processes (e.g., active transport). o Electrochemical gradients, such as those created by the sodium-potassium pump, generate voltage across membranes (important for nerve function). 2. Signal Transduction Pathways: o Signal transduction involves receptor proteins that activate intracellular pathways (e.g., phosphorylation cascades). o Small signals can be amplified to produce larger responses (e.g., hormone action).