Gene Regulation and Mutation PDF
Document Details
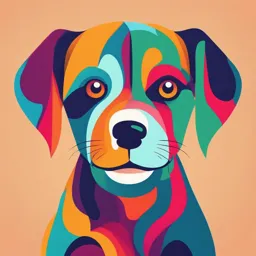
Uploaded by SilentCrocus
De La Salle Medical and Health Sciences Institute
Sheila Marie P. Torres M.S.
Tags
Related
- Control of Gene Expression – Ch 33 PDF
- Lecture 14a: General Principles of Gene Expression Regulation in E. Coli PDF
- Chapter 29: Transcription and the Regulation of Gene Expression PDF
- Gene Expression Regulation: Lecture Notes PDF
- BIOCHEMISTRY REGULATION OF GENE EXPRESSION & MUTATIONS PDF
- Gene Expression Biochemistry BUCM 2024-2025 PDF
Summary
This document explores gene regulation, focusing on the lac operon, tryptophan operon, and general principles of regulation. It also includes a brief overview of eukaryotic gene regulation mechanisms.
Full Transcript
Mutation and Regulation of Gene Expression Sheila Marie P. Torres M.S. Department of Biochemistry, College of Medicine, De La Salle Health Sciences Institute Types of Gene Regulation Positive Regulation – Expression of the genetic information is...
Mutation and Regulation of Gene Expression Sheila Marie P. Torres M.S. Department of Biochemistry, College of Medicine, De La Salle Health Sciences Institute Types of Gene Regulation Positive Regulation – Expression of the genetic information is quantitatively increased by the presence of specific regulatory element (activator/inducer) Negative Regulation – Expression of the genetic information is diminished by specific regulatory element (repressor) Types of genes 1. inducible- needs an inducer or activator 2. constitutive- housekeeping genes, genes that are expressed at a constant rate Regulation of gene expression Occurs at different steps of gene expression But gene expression is controlled mainly at transcription level Regulation of gene Expression in Prokaryotes Operon Simple mechanism Genes are clustered on the chromosome and are transcribed together Only a single promoter is required to initiate and control the expression of these related genes Lac Operon Contains: Regulatory gene (lac i): produces the repressor protein Promoter site (P) for the binding of the RNA polymerase With two specific regions: Catabolite activator protein binding site (CAP) site RNA Pol entry site Operator site (O) – lac repressor binds to this site and blocks initiation of transcription Three structural genes: Z- for β-galactosidase Hydrolyzes lactose into glucose and galactose Y- for permease Transport of lactose across the cell membrane A- for thiogalactoside transacetylase Always “turned off” but Inducible Regulation of the lac operon Bacteria usually depend on glucose as the primary source of energy In the absence of glucose , lactose can be used as energy source 1. negative regulation by lac repressor in the absence of lactose and presence of Glucose Lac repressor binds the O site and blocks RNA Pol access of the structural genes No transcription and translation 2. positive control by induction of expression in the presence of lactose and absence of Glucose Permease allows transport of lactose through the cell membrane β-galactosidase cleaves lactose into glucose and galactose allolactose is formed by combining glucose and galactose through α1-6 linkage allolactose serves as inducer and binds the repressor protein repressor protein cannot bind the O site due to conformational change in its structure RNA Pol is allowed to have basal transcription of the structural genes Enhance by CAP-binding protein 3. positive control by catabolite repression in the presence of glucose and lactose Glucose is metabolize first Glucose inhibits the high rate of transcription by lowering the concentration of cAMP When glucose is exhausted, cAMP concentration increases cAMP binds to CAP-binding protein the complex binds to the CAP-binding site in the promoter this enhances transcription of the structural genes by the RNA polymerase Tryptophan Operon genes for the enzymes of tryptophan biosynthetic pathway repressible genes o Genes whose expression is turned off by the presence of a repressor The regulatory gene produces repressor but is inactive (apo-repressor) The 5 structural genes produce proteins essential for tryptophan synthesis In the absence of tryptophan, the operon is on Inactive repressor cannot bind the operator site Excess tryptophan represses transcription Tryptophan acts as co-repressor that activates the apo-repressor Binding of the activated repressor blocks RNA Pol transcription of the structural genes Regulation by attenuation Regulation by premature termination of transcription Depends on coupled transcription and translation Never occur in eukaryote e.g. attenuation in the histidine operon His Operon contains genes for the synthesis if histidine and produces histidine enzymes for the biosynthetic pathway of histidine when histidine is not available in the medium. When histidine is readily available the operon is turned off. One way to control his operon is by attenuation. Transcription is constitutively initiated at the 5UTR of mRNA As soon as the Shine Dalgarno appears at the 5UTR of the mRNA, ribosome binds to translate the 5’UTR (leader sequence) into a non functional protein known as the leader peptide. If histidine is abundant in the surrounding, the ribosome easily find His tRNA and the leader peptide is formed. A secondary structure, hairpin loop is formed. RNA Pol stops transcription before it reaches the structural genes. In the absence of histidine, the ribosome stalls at histidine codon because it cannot easily find his tRNA. The message does not fold into a secondary structure. RNA Pol continues to transcribe the structural genes. Mechanism of Regulation of gene Expression in Eukaryotes 1. chromatin remodeling 2. Covalent modification of the histone tails through acetylation 3. DNA methylation 4. Gene amplification 5. Gene Deletions DNA of eukaryotic organism are not naked and are complex with histones. The nucleosome core provides barrier for the formation of the transcriptional apparatus especially when the promoter is included in the nucleosome core. Hence the need for chromosome remodeling 1. Chromatin Remodeling/Nucleosome Remodeling Displacement of the nucleosome from specific DNA sequences ATP driven Activates the gene 2. Covalent Modification of the histone tails Through acetylation – Histone acetyltransferases (HATs) transfer the acetyl group from acetyl coA to lysine residues in the tail of the histone octamer – Reduces electrostatic interaction between histone and the DNA. Unwinding of the DNA from histones – Some repressor or co-repressor contains Histone deacetylases that removes acetyl groups and inactivates the gene Epigenetic Effects on Gene Regulation o Chemical modifications of DNA o There is no change in the base sequence and therefore not a mutation o It usually involves methylation of cytosine in CG sequences Such methylation usually silences the gene Example: extreme condensation silences the gene like what happened in heterochromatin Heterochromatin is highly compacted during interphase and is found in regions near the centromere Constitutive heterochromatin remains condensed most of the time in all cells (Euchromatin on the other hand contains all active genes that are always transcribed) Methylation on the CPG island of the FMR1 gene silences the gene leading to none production of the FMR1 protein in Fragile x Syndrome Heterochromati 3. DNA Methylation Cytosine on both strands may be methylated to 5-methylcytosine (Inactivates the gene) Maintenance of inactive heterochromatin Most important type of gene regulation in preventing the transcription of the genes intended to be permanently turned off Occurs in the CpG islands (usually near promoters) Housekeeping genes-CpG islands are unmethylated In Tissue specific genes- these CpG islands maybe methylated to silenced the genes 4. Gene Loss Genes are deleted or partially deleted from cells Non production of functional protein 5. Gene Amplification Certain regions of chromosomes undergo repeated cycles of replication Not a usual physiological means The newly synthesized DNA is excised and form small unstable chromosomes called double minutes o Integrate into other chromosome throughout the genome therefore amplifying the gene Transcriptional Level of Control Enhancers and silencers are DNA sequences that can control activities of the gene although they are hundreds of base pairs away from the promoter Activators and Repressor proteins bind to these elements. These protein are known as special transcription factors. Transcription Factors Activator proteins that bind response elements With 2 recognizable domains: – DNA-binding domain Binds to specific nucleotide sequence in the promoter or response element Used to define certain families of transcription factors – Activation domain Binds to other transcription factors Interact to RNA Pol II to stabilize the formation of the initiation complex Recruit chromatin modifying proteins such as HATs or HDATs Selected DNA binding motifs 1. Helix-turn-helix Homeodomain 2. Zinc Fingers (steroid hormone receptor) Cys4 zinc finger Cys2 His2 zinc finger (e.g. TFIIIA) 3. Basic domains Leucine zippers factors (bZIP) Basic helix-loop-helix (bHLH) 4. Beta-scaffold factors with minor groove contacts HMG (High mobility group) proteins Properties of Some Common Transcription Factors Transcription Response Function Protein Class fcctor (DNA Element binding (binding site) protein SP-1 GC-rich basal Zinc Finger NF-1 CCAAT box basal Steroid HRE Steroid response Zinc Finger Receptor cAMP CRE response Response to cAMP Leucine Zipper response element element (CREB) protein Homeodomain Regulation during Helix turn helix Proteins development SP-1 and NF-1 are general transcription factors whereas the rest are specific transcription factors. TFIID (TATA factor) is also a kind of general transcription factor that bind the TATA box HORMONES May regulate the activity of some transcription factors. Examples include the steroid receptors and the CREB protein. e.g. control of Gluconeogenesis by response elements Glucagon binds to a receptor in the membrane cAMP is increased Protein kinase A becomes active Protein kinase phosphorylated CREB and activates it Activated CREB enters the nucleus and binds to the CRE gene associated with PEPCK gene Increases gene expression Increases PEPCK in the cell Increases rate of gluconeogenesis Cortisol diffuses into the hepatocyte Binds to the receptor Complex enters the nucleus Binds to the glucocorticoid response elements associated with PEPCK Increases gene expression Increases rate of gluconeogenesis Post Transcriptional Regulation Alternative Splicing – Differential splicing of introns – Enables the gene to code for more than one different protein Regulation of mRNA stability Differences in mRNA half-lives Ends of the mRNA (g cap and poly A tail ) help protect the mRNA from degradation RNA interference (RNAi) Mechanism of gene silencing through decreased expression of mRNA either by repression of translation or by increased degradation Plays a key role in cell proliferation, differentiation and apoptosis Mediated by noncoding RNA- miRNA miRNA associates with RISC (RNA-induced silencing complex) Complex binds with mRNA Tags the mRNA for degradation by endonuclease Control of the phosphorylation of eIF2 eIF2 involved in the formation of the ternary complex during initiation eIF2 contains 3 subunits, α, β, γ phosphorylation on serine of the alpha subunit by different kinases that are activated when cell are under stress phosphorylation makes the binding of eIF2 on GTP-GDP recycling protein (eIF2-B) tight this inactivates the complex and 43S preinitiation complex could not be completed another point of control is the activation of mRNA 4E when bound to BP-1 could not bind to 4G and therefore cannot form 4F. Insulin and mitotic growth factors activates kinases that phosphorylates BP-1. Phosphorylation causes the dissociation of 4E to BP-1. 4E can now bind to 4g to form 4F. Activation of mRNA follows. Mutation A heritable change in the DNA sequence – Could be passed on to daughter cells if the mutated cell divided Mutations can occur on a small scale, most often affecting one or two nucleotides of DNA, or they can involve large segments of a chromosome or an entire chromosome. Any mutation in a gene’s DNA can alter the function of the protein encoded by the gene. Classification Based on Phenotypic Effects Loss of function mutation – Eliminates the function of the gene product – Also called null mutations or gene knockouts Gain of function mutation – Give gene product a new function – Generally a dominant allele – Can give a protein a new function or cause a regulatory effect to express old protein at new time or under new conditions Classification Based Upon Type of Molecular Change Point Mutation changes in a single nucleotide of an organism’s DNA. Base substitution mutation Classification. Substitution Mutations A substitution is the replacement of a single nucleotide with another. It is classified by its effect on the protein produced: Silent mutations have no effect on the protein. Missense mutations result in a single amino acid change in the translated sequence. Nonsense mutations result in an amino acid codon being replaced by a “stop” codon. Nonsense mutations end translation prematurely and result in a truncated protein. Examples: Sickle Cell Anemia Sickle cell anemia (or sickle cell disease) is caused by a point mutation that affects the shape of red blood cells. People with sickle cell anemia experience frequent pain, infections, and other symptoms. Genetic Homogeneity- single gene disorder Rett Syndrome Rett syndrome primarily affects girls and causes severe learning, communication, and coordination problems. Its most common cause is mutations in the MECP2 gene on the X chromosome. These mutations include changes in single base pairs, insertions or deletions of DNA in the gene, and changes that affect how the gene is processed into a protein. (Allelic Heterogeneity) Insertions and Deletions (Indels) Insertion and deletion mutations occur when one or more base pairs are inserted into or deleted from the DNA sequence. mRNA is translated three nucleotides at a time. Insertions and deletions that do not involve three nucleotides or multiples of three nucleotides change the translation of all the mRNA downstream of the mutation. These frameshift mutations almost always result in a nonfunctional protein. In frame Mutation No change in the reading frame but lead to missing codon/s Usually deletion or insertion of nucleotides that are multiples of three Mutations affecting translation hemoglobin Wayne (3’ terminal frameshift mutation) Hemoglobin Wayne results from a frameshift mutation that lies very close to the normal termination codon of the alpha-globin gene. As shown in the figure, the U (in blue) is deleted, which is in the third position of the serine codon. This changes the serine codon from UCU to UCA (and thus does not affect that particular amino acid). However, the reading frame has been disrupted, changing the downstream amino acid sequence. In fact, the normally in-frame UAA stop codon is no longer in frame and is therefore skipped. This results in a protein that is also five amino acids longer than normal. Other mutations that affect translation include missense mutations (one amino acid for another); nonsense mutations (premature stop codons); and read through, reverse terminator, or sense mutations (an amino acid for a stop codon). Silent mutations convert one codon to another, but do not change the amino acid being specified. Hemoglobin Constant Spring (see above) results from a read-through mutation. This allows the ribosome to continue into the 3' UTR. When it does so, it displaces the so-called alpha-complex, which functions to slow deadenylation (removal of the poly(A) tail). Hence, there is accelerated mRNA decay and insufficient alpha-globin production (Mendell and Dietz, Cell 107:411; 2001). Trinucleotide repeat Expansion Mutant alleles differ from their normal counterpart only in the number of tandem copies of trinucleotide Some protein-coding genes contain trinucleotide repeats, or sequences of three nucleotides repeated several times. The number of repeats can increase, or expand, due to an error during DNA replication. The repeat expansion creates a new allele, but the protein still functions. However, when the number of repeats exceeds the “normal” threshold for the gene, the protein no longer functions properly. expansions of trinucleotide repeat regions in or adjacent to genes – Expansion believed result of slippage during DNA replication – Eg. Hungtinton’s disease – fragile x syndrome – myotonic dystrophy Huntington Disease Fatal neurodegenerative disease HD gene on chromosome 4 CAG repeat in the gene’s coding region – 10-35 gives normal phenotype – >35 gives symptoms (up to 120 known) Age of onset is repeat number dependent – Excess CAG repeats give excess glutamine residues in the encoded protein Fragile X FMR-1 gene CGG repeat in 5’-UTR of gene 230 gives fragile X phenotype, including MR CG is a methylable regulatory sequence in higher eukaryotes – Excess copies causes gene to be turned of Myotonic Dystrophy MDPK gene (serine-threonine protein kinase) 15 exons, last one encodes 3’-UTR 3’-UTR has CTG repeat – 5-37 repeats given normal phenotype – >37 give afflicted phenotype Onset and severity depends upon number of repeats – 150 gives minimal impact – 1500 gives severe early onset of symptoms Genetic anticipation, molecular basis of problem unknown at this time Types of Mutations Spontaneous mutations – Happen naturally, not as a result of any specific agent(s) Replication errors or base modification as a result of chemicals naturally present in the cell (oxygen, acids, etc.) Induced mutations – Mutations that result from the the influence of an extraneous factor X-rays, UV light, chemicals not of cellular origin Sources of Spontaneous Mutation 1. Replication errors – Replication errors not detected/repaired by DNA repair systems – Tautomerism of bases contribute to this problem 2. Replication slippage – One strand of DNA becomes displaced during replication, annealing somewhat offset from its original position on the complementary strand Creates loop out region Relatively common in tandem repeat regions (e.g. STRs) 3. Depurination and Depyrimidination Bases “fall out” of DNA – Purines more than pyrimidines – N-glycosidic bond failure… – Create AP sites (apurinic or apyrimidinic) – Loss of genetic information at site Rate enhanced by low pH Each cell loses 100s of purines per day… 4. Deamination Amino groups converted to keto groups – Cytosine, adenine and guanine Cytosine uracil Adenine hypoxanthine Guanine xanthine 5-methyl cytosine thymine – Hot spots for mutation… – Oxidized bases (deaminated) often pair differently than the nonoxidized form – Spontaneous or through chemical treatments (e.g. nitrous acid) 5. Transposable Elements Agents of many spontaneous mutations in both prokaryotes and eukaryotes – Insertion mutations – Deletions – Rearrangements – Induced Mutations: Chemical Damage Base Analogs – Molecules that can substitute for purine/pyrimidine nucleosides/nucleotides and be incorporated into DNA – Do not pair properly – 5-bromouracil (or 5-bromodeoxyuridine) – Thymine analog (bromine for methyl group) – Improper tautomeric equilibrium Enol tautomer pairs well with guanine and adenine 2-amino purine is an analog of adenine (6-amino purine) but also pairs with guanine Radiation UV radiation – Nonionizing radiation – Excites electrons to a higher energy level – C and T are most vulnerable to excitation – Can cause adjacent thymine bases in the DNA to pair with each other References Majority of the figures and illustrations here are downloaded from Google Alberts, Bruce. Et al. Molecular Biology of the Cell. 6th ed. Garland Science, Taylor and Francis Group. 2015. Bohisnki, R.C. Modern Concepts in Biochemistry, 5th ed. Boston: Allyn and Bacon Inc. Devlin, Thomas; et.al. Textbook of Biochemistry with Clinical Correlations. 7 th ed. John Wiley and Sons. 2011. pp. 808-838. Harvey, Richard A; et. al. Biochemistry, Lippincott’s Illutrated Reviews. 5 th Edition. Wolters Kluwer. 2011. Halkerson, D.K. Biochemistry. 2nd ed. John Wiley and Sons. 1990. Marks, A., Lieberman M. Mark’s Basic Medical Biochemistry. A Clinical Approach. 4 th ed. Wolters Kluwer/Lippincott Williams and Wilkins. 2013 Murray, Robert K. ; et.al. Harper’s Illustrated Biochemistry. 29th ed. Mc GrawHill Lange. 2012. Naik, Pankaja. Biochemistry. 3rd edition. Mc Graw Hill. 2009.