IB Biology HL Notes.pdf
Document Details
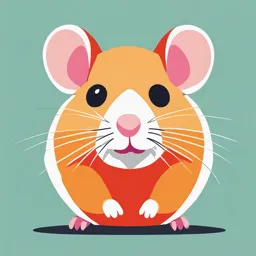
Uploaded by IrresistibleDiscernment5991
IB
Tags
Full Transcript
A unity and diversity - cells A1.1: Water A1.1.1 Water as medium of life State that the first cells originated in water. List reasons why water is a substance on which life depends Water is a substance depends on ➔ The first cells originated in water ➔ Water is a solvent ◆...
A unity and diversity - cells A1.1: Water A1.1.1 Water as medium of life State that the first cells originated in water. List reasons why water is a substance on which life depends Water is a substance depends on ➔ The first cells originated in water ➔ Water is a solvent ◆ Allowing it to dissolve and transport molecules around a body ➔ Water is a metabolite in condensation and hydrolysis reactions ➔ Water is a temperature buffer ◆ Enzyme activity is sensitive to temperature and reaction only occur in a narrow range of temperature ◆ water helps to buffer temperature changes in cell because of its relatively high specific heat capacity ➔ Water maintains biological structures (e.g. cell membrane, protein folding, DNA double helix) A1.1.2 Hydrogen bonds as a consequence of the polar covalent bonds within water molecules Atomic structures ➔ An atom is the smallest unit of matter with protons, neutrons, electrons ➔ Electrons in an atom are attracted to proteins in the nucleus by an electromagnetic force (positive charge attracts negative charge) Atomic bonding ➔ Atoms are able to form bonds, a attraction between atoms, ions or molecules, including ◆ Non polar covalent bond, share electrons equally ◆ Polar covalent bond , share electrons unequally ◆ Ionic bond, attraction between a positive and negative charged ion ◆ Hydrogen bond, attraction between two polar molecules, between slightly positive and slightly negative region of two different polar molecules ➔ Within water molecule electrons are shared through polar covalent bonding between the atom ➔ Water molecules are partially attracted to each other (cohesion), + hydrogen to - oxygen A1.1.3 Cohesion of water molecules due to hydrogen bonding and consequences for organisms ➔ Cohesion: the water’s ability to make hydrogen bonds with itself, causing water molecules to stick together ◆ Allows plants to move water up roots (transpiration), retains water on Earth’s surface, contributes to the physical property of water important to living organisms ➔ Transport of water under tension in xylem ◆ Xylem tissue are long, tubular vessels in vascular plants that form a continuous tubes from the roots to the leaves ◆ Transpiration (evaporation) occurs through the stomata of a leaf. As transpiration occurs, it creates negative pressure (tension) ◆ Water moves upwards due to tension/pulling forces ◆ Cohesion pulls up water molecules in a chain as the top most water is pulled up and out of the stomata ➔ Surface tension: a property of the surface of a liquid that allows it to resist an external force, due to the cohesive nature of its molecules ◆ The molecules on the surface area are more attracted to other molecules of the liquid than to molecules in the surrounding air ◆ Net effect: an inward force that cause water to behave as if its surface were covered with a stretched elastic membrane ➔ Water surfaces as habitats ◆ Surface tension allows water striders to walk on water surface and provides stable environment for other organisms that live on or near the surface of water ◆ Enough force is needed to break the hydrogen bonds and the water surface A1.1.4 Adhesion of water to materials that are polar or charged and impacts for organisms. Hydrogen bonds form between water and polar or charged molecules ➔ Adhesion: the attraction of water to other polar or charged molecules ◆ Allows plants to move water using capillary action ◆ Permits water to move through soil, even against the force of gravity ➔ Hydrophilic substances are attractive to water because they can make intermolecular bonds with the water ◆ Polar and charged molecules are hydrophilic ➔ Polar molecules will dissolve in water as they are “hydrophilic” and can form hydrogen bonds with water ◆ e.g glucose, has five hydroxyl (-OH) groups along the carbon skeleton, and is polar. “O” in each has slight negative charge, “H” has a slight positive charge ➔ Water is able to dissolve charged molecules because water is electrostatically attracted to ions Impact of adhesion for organisms ➔ Cellulose: a polymer made of glucose, presenting in plant cell walls ◆ Allows structures with a lot of cellulose to absorb water; water sticks to the cellulose via hydrogen bonds ➔ Capillary action: the movement of water in through a narrow space, often in opposition to external forces like gravity ◆ Plants and trees cannot thrive without capillary action ◆ Helps bring water up into the roots, with help of adhesion and cohesion, water and dissolve ions can move all the way up to the branches and leaves. ◆ Adhesion of water to the walls of a vessel will cause an upward force on the liquid ➔ Capillary action enables soil to retain water. Water moves upwards through soil pores, against gravity, similar to how it moves up a tube. A1.1.5: Solvent properties of water linked to its role as a medium for metabolism and for transport in plants and animals ➔ solvent + solute → solution ◆ Solvent: the liquid in which a solute dissolves ◆ Solute: the substance that dissolved in a solvent ➔ Solvation: the interaction of a solvent with a dissolved solute ➔ Water forms hydrogen bonds with the solute, forming a hydration shell around the solute ➔ Not everything will dissolve in water ◆ Molecules that are nonpolar do not have a charge atoms do not dissolve in water as they are “hydrophobic” (cannot attract water, insoluble, non polar) They will clump together when exposed to water Role of water as a medium for metabolism ➔ Cytosol: the liquid part of the cytoplasm, common to all cells ◆ Composed of about 80% water and contains dissolved salts, fatty acid, sugars, amino acids, and proteins such as enzymes ◆ These substances are needed to carry out metabolic processes to keep cell alive ➔ Water is needed for cellular metabolism because it dissolves the reactants and enzymes ◆ Catabolic reactions break down large molecules into smaller ones ◆ Anabolic reactions build larger molecules from smaller ones Transports in plants ➔ Mineral ions are transported in xylem sap ➔ Sucrose and other products of photosynthesis are transported in phloem sap Blood transports ➔ Salt ions ( Na+ and Cl-) ➔ Amino acids (both negative and positive charges) ➔ Proteins (antibodies and those used in blood clotting) ➔ Glucose (polar molecule, freely soluble and carried in plasma) ➔ Waste products of metabolism (urea which is later removed from the blood at the kidney) ➔ A small amount of dissolved gases (CO2 and O2) A1.1.6 Physical properties of water and the consequences for animals in aquatic habitats ➔ Water’s physical properties make it the medium of life ➔ Physical property: a measurable characteristic of matter that do not involve chemical reactions ◆ Buoyancy ◆ Viscosity ◆ Thermal Conductivity ◆ Specific heat capacity Physical property 1: Buoyancy ➔ Buoyancy: a force exerted upwards on an object ◆ If the buoyant force of the fluid is greater than the object’s weight, the object will float ◆ Denser objects will sink, less dense objects will float Physical properties 2: Viscosity ➔ Viscosity: a measure of a fluid’s tendency to flow ➔ It is due to the amount of friction the molecules of a liquid experience as they flow over each other. ◆ A thick fluid is more viscous, thin fluid is less viscous ➔ Blood > water > air ◆ Air: molecules have little friction as they flow over each other, so air is not viscous ◆ Water: can form hydrogen bonds, increasing friction and viscosity ◆ Blood: dissolved solutes and cells in blood increasing friction as it flows and viscosity Physical properties 3: Thermal Conductivity ➔ Thermal conductivity: a measure of a material's ability to move heat across a temperature gradient ◆ Less conduction, heat slowly moves through the material, better insulation and preventing heat loss ◆ More conductive, heat rapidly moves through the material, better for absorbing and transferring heat ➔ Styrofoam < air < wool < fat < water < copper ➔ Water rapidly removes heat from marine mammals' bodies, causing greater heat loss compared to being in air. Physical properties 4: Specific heat Capacity ➔ Specific heat capacity: the quantity of heat needed to raise the temperature of a chemical per unit mass ◆ Water has the highest specific heat capacity of any liquid, good for temperature regulation (takes a lot of heat energy to raise the temperature of water) ➔ Water’s high heat capacity is caused by its numerous hydrogen bonds ◆ Each “bond” is weak, but requires a lot energy to break them all ➔ Benefits: Water heats up or cools down very slowing, allowing a stable internal environment and habitat of living things ◆ E.g. The high specific heat capacity of water in living bodies helps stabilise body temperature when environmental temperatures fluctuate, preventing enzymes from denaturing. Buoyant Force Viscosity Thermal Specific heat conductivity capacity Water Higher Higher Higher Higher (water applies more (water is more (water absorbs and (it takes more upward force that air, resistant to flow) transfers heat) energy to change allowing objects to float) the temperature of water) Air Lower Lower Lower Lower (Air applies less upward (Air is resistant to (heat is lost slower (it takes less energy force than than water, so flow) to the air) to change the most doesnt not float in temperature of air) air) The black-throated loon (Gavia arctica) interacts with the physical properties of water in their habitat. ➔ Buoyancy in water allows birds to stay afloat without expending a lot of energy ◆ However, the viscosity of water required adaptations for streamlining when swimming ➔ Air is not viscous, allowing the loon to easily move through it when flying ➔ Air has low thermal conductivity, so the loon doesn't lose as much body heat to air ◆ But air has low specific heat, cousin its temperature to change rapidly A1.1.7* Extraplanetary origin of water on Earth and reasons for its retention ➔ Water covers 71% of the earth’s surface ➔ Earth formed around 4.5 billion years ago, water may have begun to exist on earth as early as 4.4 billion years ago ➔ The leading hypothesis is that water was brought when asteroids containing ice collided with the early earth ➔ 2 factors of how water was retained on early earth: ◆ The distance of the earth from the sun keeps temperature on earth below that needed to vaporise water ◆ Earth’s gravity keeps water from escaping the planet A1.1.8* Relationship between the search for extraterrestrial life and the presence of water ➔ Goldilocks Zone: the area around a star where it is not too hot and not too cold for liquid water to exist on the surface of surrounding planets ◆ If a planet is too close to a star, water will vaporise; too far away and water freezes A2.2: Cell structure A2.2.1 Cells as the basic structural unit of all living organisms Cell Theory - Atypical examples (exceptions): Striated muscle, giant algae and aseptate fungal hyphae 1. All living things are composed of cells 2. Cells are smallest unit of life 3. Cells come from pre-exisiting cells A2.2.2 Microscopy skills ➔ Microscopes: devices that produce a magnified image of objects too small to be seen directly by eyes. ➔ Magnification: how much larger an object appears compared to its real size. ◆ M = I/A ➔ The field of view (FOV): the diameter of the area visible through the microscope. ◆ Formula: 𝐷𝑖𝑎𝑚𝑒𝑡𝑒𝑟 (𝑚𝑒𝑎𝑠𝑢𝑟𝑒𝑑 𝐹𝑜𝑣 𝑎𝑡 𝑙𝑜𝑤 𝑝𝑜𝑤𝑒𝑟) × 𝑚𝑎𝑔𝑛𝑖𝑓𝑖𝑐𝑎𝑡𝑖𝑜𝑛 𝑜𝑓 (𝑙𝑜𝑤 𝑝𝑜𝑤𝑒𝑟 𝑚𝑎𝑔𝑛𝑖𝑓𝑖𝑐𝑎𝑡𝑖𝑜𝑛) 𝑜𝑏𝑗𝑒𝑐𝑡𝑖𝑣𝑒 𝑚𝑎𝑔𝑛𝑖𝑓𝑖𝑐𝑎𝑡𝑖𝑜𝑛 𝑜𝑓 (ℎ𝑖𝑔ℎ 𝑜𝑟 𝑚𝑒𝑑𝑖𝑢𝑚 𝑝𝑜𝑤𝑒𝑟 𝑚𝑎𝑔𝑛𝑖𝑓𝑖𝑐𝑎𝑡𝑖𝑜𝑛) 𝑜𝑏𝑗𝑒𝑐𝑡𝑖𝑣𝑒 = 𝑑𝑖𝑎𝑚𝑒𝑡𝑒𝑟(𝐻𝑃) 1. Estimate (or count) numbers of specimen would fit across FOV 2. Divide the FOV diameter by that estimated count. E.g. If the field of view diameter is 600 μm and about 2.3 of the object would fit across the FOV, divide the diameter by 2.3 to find the object’s length. ◆ The higher the power of magnification, the smaller the field of view 1 mm = 1,000 µm 𝑠𝑐𝑎𝑙𝑒 𝑏𝑎𝑟 𝑚𝑒𝑎𝑠𝑢𝑟𝑒𝑚𝑒𝑛𝑡 𝑙𝑎𝑏𝑒𝑙 𝑚𝑒𝑎𝑠𝑢𝑟𝑒𝑚𝑒𝑛𝑡 1 cm = 10,000 µm Must be be in the same unit A2.2.3 Developments in Microscopy ➔ Resolution: Making separate parts of an object distinguishable by eye (A measure of how detailed the image is) ➔ Staining: coloured substance used to enhance visibility or label specific structures ◆ Fluorescent stains: glowing dyes for visualising structures under special light, generating a brighter image ➔ Immunofluorescence: a technique that uses fluorescently stained antibodies to visualise specific proteins within a cell. (Frye&Eddin discovered that cell membrane can have movement in 1970) ◆ The protein tagged with the immunofluorescence can be located and tracked as it moves in the cell. Compound Light Microscope Electron Microscope Low magnification of (1500x) Up to 10,000x to 30,000x Low resolving power (0.25-0.3µm) High resolving power (0.001µm) See dead or living cells in 2D coloured image See dead specimens (2D&3D black white image) Not clear Clear image cheaper Expensive Low voltage High voltage Movements can be seen No movements can be seen ➔ Freeze-fracture: an electron microscope preparation technique, was developed in 1960’s ◆ Rapid freezing of cells → fracturing of cells along lines of weakness, including through the centre of membranes → surfaces etched with a coasting → creating a replica of surfaces that can be viewed with an electron microscope. ◆ Provides planar views of the internal structure of membrane ◆ Discovery: integral (transmembrane) proteins are scattered through the centre of membranes. A2.2.4 Structures common to cells in all living organisms Similarities Between prokaryotic and eukaryotic cell: ➔ All cells have a plasma membrane ➔ All cells contain genetic materials Structures: Plasma membrane, cytoplasm, DNA, ribosomes A2.2.5 Prokaryotic cell structure (bacteria and archaea) ➔ No nucleus, mitochondria, or any membrane bound organelles ➔ Simple cell structure without compartments ➔ Prokaryotic cells were the first cells to evolve ➔ Mostly small in size and can be found everywhere ➔ Divide through binary fission. Functions of structures in prokaryotic cells: ➔ Plasma membrane: regulates what materials enter and exit the cell ➔ Cytoplasm: gel-like fluid substances (mostly water with many dissolved molecules), site of metabolic reactions. ➔ 70s Ribosomes: build proteins during translation ➔ Cell wall with peptidoglycan: protects the cell, maintain its shape and prevents it from burstin ➔ Pili: hair-like extensions, attach the cells to surfaces ➔ Capsule: helps the cell keep from dehydrating and adhere to surfaces ➔ Flagellum: long extensions used in cell locomotion (the movement of an organism from one place to another), it spins like a corkscrew ➔ Nucleoid: DNA can found in nucleoid and found freely in cytoplasm ◆ DNA is a single loop, not wrapped around proteins, called “naked” ➔ Plasmid: carry genes for replicating their DNA ◆ A small, circular, and naked DNA, containing genes for antibiotic resistance Prokaryotic cell are “Naked” means the DNA is not associated with proteins A.2.6 Eukaryotic cells (animal & plants) ➔ Divide through mitosis or meiosis ➔ Have membrane-bound organelles. Descriptions and functions of structures in eukaryotic cells: ➔ Nucleus: makes ribosomes for protein synthesis, chromosomes (containing DNA + associated histone proteins) to carry genetic information ➔ Ribosomes: site of protein synthesis in the cell ➔ Endoplasmic reticulum: transport of materials ◆ Rough ER: a series of connected flattened membrane sacs, continuous with the outer membrane of the nuclear envelope has 80s bound ribosomes attached Function: synthesis & transport of polypeptide to inside of RER ◆ Smooth ER: Similar to RER, but no ribosomes and not involved in protein Function: produce and transport lipids ➔ Golgi apparatus: collects, packages, and transports proteins into vesicles (secretion through exocytosis) vesicle buds off from golgi ➔ Mitochondria: production of ATP by aerobic respiration (main site of cellular respiration) ➔ Lysosome: small spherical organelle, contains digestive enzymes to degrade and recycle components of damaged or old organelles ➔ Vesicles: contain and transport material within cells ◆ Transport vesicles: move materials to another location ◆ Secretory vesicles: kicks out cells (exocytosis) ➔ Chloroplast: photosynthesis (take in sunlight, water, and carbon dioxide to produce glucose), are green because they contain light-absorbing pigment chlorophyll ➔ Vacuole: Animal cells have multiple smaller vacuoles primarily for water storage, while plant cells have a large singular vacuole that stores various substances including chemicals, waste products, and proteins. ➔ Microtubules: are polymers of a protein, called tubulin, used for intracellular transport of organelles and the separation of chromosomes during mitosis ➔ Centriole: consist of 9 groups of triplet microtubules, organising microtubules that serve as the cell's skeletal system ➔ Cilia & Flagella: enables movements in cells ◆ Cillia: shorter and numerous ◆ Flagella: longer Protein secretion Rough ER → Golgi Apparatus → Secretory vesicles → Cell exterior. A2.2.7 Unicellular organisms Metabolism: chemical reactions inside the cell or in an organism Reproduction: produce offspring: Asexual or Sexual Homeostasis: maintenance and regulation of internal cell condition Growth: grow or change in size Response: ability to react to changes in environment Excretion: get rid of waste products, including carbon dioxide from respiration Nutrition: living things exchange materials and gases with the environment - Autotroph: organisms that produce its own food e.g. plants - Heterotroph: organisms that doesn’t make its own food e.g. animal How does unicellular cells carry all functions of life? Function Paramecium (Asexual) Chlamydomonas Nutrition Is a heterotroph, eats smaller Is a autotroph, produces its own food by unicellular organisms photosynthesis using a chloroplast Response can control beating of cilia to Light sensitive "eyespot" allows sensing move in different directions light and swim to it using its two flagella Excretion Waste products from digestion are Expels waste products of metabolism e.g. excreted through an anal pore O2 from photosynthesis diffuses out of the cell Growth Increases in size and dry mass Will *grow* until it reaches a maximum by accumulating organic matter surface area to volume ratio and minerals from its food Homeostasis Keeps internal conditions within limits e.g. expels excess water using contractile vacuole Reproduction Reproduces asexually using mitosis or sexually using meiosis and gametes Metabolism Produces enzymes which catalyse many different chemical reactions in the cytoplasm A2.2.8 Difference in eukaryotic cell structures between animals, fungi, and plants Structures Animal Cell Fungi Cell Plant Cell Plastids Present, including chloroplasts (double membrane, produce None None for photosynthesis and and store food) amyloplasts to store starch Cell wall Cells of fungi and plant have cell wall, composed of (a rigid layer outside the None chitin in fungi and cellulose in plants membrane to strength and protect the cell) Vacuole Small temporary vacuoles Present as a large, permanent vacuole in cells of fungi (membrane bound expel excess water or other and plants, used for storage of substances and organelles that store and/or waste products pressurising the cell waste products) Centrioles Used to arrange the mitotic Absent, except in fungi and plants with swimming (Cylindrical organelles that spindle during cell division and male gametes, which have a centriole at the base of organise the assembly of to serve as anchor points for the flagellum structures composed of cilia and flagella microtubules) Cilia and Flagella Present in many animal cells, Absent except in fungi and plants with male gametes (extensions from the cell including in the male gamete that swim using flagella (tails) surface which helps in cell movement) A2.2.9 Atypical cell structure in eukaryotes Discrepancies ➔ Red blood cell: no nucleus ➔ Aseptate fungal hyphae: many nuclei ➔ Skeletal muscle fibre: many nuclei ➔ Phloem sieve tube element: no nucleus Red Blood cells (erythrocytes) ➔ Carry oxygen from the lungs and deliver it throughout the body ➔ Removal of nucleus and mitochondria makes the cells very small and more flexible ◆ Allowing the cell more room for haemoglobin (carrier of oxygen) and enabling the cell to transport more oxygen ◆ Biconcave shape increases their surface area to volume ratio for efficient gas exchange. ◆ Ability to move through narrow capillary vessels. Aseptate Fungal Hyphae ➔ Aseptate fungal hyphae are tube-like structures that contain no cell membranes between the many nuclei. ➔ Sometimes are not divided up into individual cells (aseptate hyphae), resulting in a continuous cytoplasm along the length of the hyphae. ➔ Discrepancy: continuous structures with multiple nuclei Skeletal muscle cell ➔ Skeletal muscle fibre cells result from the fusion of multiple cells, resulting in a single large cell that has multiple nuclei ➔ Discrepancy: A very large eukaryotic cell with more than one nucleus Phloem Sieve Tube Element ➔ Specialised cells in the phloem tissue of plants ➔ Transports organic compounds made during photosynthesis ➔ Lose nucleus and organelles to create more space for phloem sap transport. ➔ Discrepancy: a eukaryotic cell without organelles A2.2.10 Cell types and cell structures viewed in light and electron micrographs Micrograph: magnified photograph taken through a microscope to depict an item, often using electron microscopes for higher magnification Micrograph Prokaryotic Cell Plant Cell Animal Cell Eukaryotic Light Can be arranged in Always multicellular Larger size above Many discrete groups of chains. 5µ𝑚 structures are visible Larger size over 5µ𝑚 from micrographs. Small size under 5µ𝑚 Varies in shape Flat sides with visible Structures adapted to Often stained for cell wall No cell wall perform specific identification functions. Chloroplast visible Nucleus visible if Can be helical, spherical stained or rod Large water vacuole visible Electron No membrane bound Membrane bound Membrane bound Many discrete organelles organisms visible organisms visible structures are visible from micrographs. Cell wall external to Cell wall external to Only small waste plasma membrane plasma membrane vacuole present Structures adapted to perform specific Nucleoid region with Nucleoid region with functions. DNA DNA A2.2.11 Drawing and annotation based on electron micrographs Prokaryote Micrographs ➔ Ultrastructures are cellular structures that are too small to be seen with a light microscope. Therefore, it requires an electron microscope to be seen Requirements for drawing cell structures: ❖ Cell wall: uniformly thick and drawn outside the plasma membrane ❖ Capsule: drawn outside the cell wall ❖ Plasma membrane: drawn as a continuous single line ❖ Ribosome: drawn as a dark dot ❖ Nucleoid: shown as a tangle of thread or irregular shape without a nuclear membrane ❖ Flagella: shown to be longer than any pili ❖ Pili: hair like structures ❖ Plasmid: circular ring of DNA ❖ Size stated 1 to µm A2.2.12* Origin of eukaryotic cells by endosymbiosis Two processes are thought to have led to the origin of eukaryotes. ➔ Infolding of the prokaryotic cell membrane ➔ Endosymbiosis Infolding ➔ Endoplasmic reticulum, golgi apparatus, and nuclear envelope evolved from inwards folds of the membrane of ancestral prokaryotic cells ➔ Internal membranes allow cell to carry out chemical reactions in separate compartments Endosymbiosis ➔ Chloroplast and mitochondria likely evolved from small symbiotic prokaryotes that lived within the cell ➔ Both benefits from each other, and live together, one organism one organism (the endosymbiont) lives inside another (Host) ➔ Mitochondria can carry out aerobic respiration to produce ATP for the eukaryotic cell ➔ Chloroplasts can do photosynthesis ➔ Eukaryotic organelles like mitochondria, chloroplasts, and plastids evolved from free-living prokaryotes through symbiotic relationships. Evidence for the endosymbiosis theory: ➔ Structural Evidence Mitochondria and chloroplasts have similar size, shape, double membrane, and 70s ribosomes. ➔ Genetic Evidence Both organelles have circular naked DNA like prokaryotic cells and share common DNA sequences. ➔ Functional Evidence Mitochondria and chloroplasts move and reproduce independently through binary fission, similar to prokaryotes. A2.2.13* Cell differentiation as the process for developing specialised tissues in multicellular organisms Genome: all the genetic information of an organism. ➔ All cells within an organism share the same genome Differentiation and gene expression ➔ Differentiation: the development of specialised structures and functions in cells ➔ Gene expression: the process of turning gene information into function ➔ 4,000 genes expressed in nearly all cell types. ➔ “Housekeeping” genes: code for proteins involved in regulation, DNA replication, and metabolism Differentiation and Gene Expression Mechanisms: ➔ Differentiation occurs when different cell types express different genes ➔ Gene expression converts gene information into function ➔ Non- “housekeeping” genes differentially expressed in various cell types ➔ Gene expression regulation involves protein binding specific DNA sequences ➔ Stem cells differentiate by activating or deactivating genes. ◆ E.g. Egg and sperm production involves mitosis, cell growth, meiosis, and specialisation for specific functions. Differentiation during development and environmental influences: ➔ A lot of differentiation occurs during embryonic development ◆ Pluripotent cells differentiate through DNA methylation, silencing genes. ➔ Environmental conditions can change gene expression. ◆ External conditions: drugs, chemicals, temperature, and light. ◆ Internal conditions: proteins, steroids. Tissue: a group of cells that have differentiated in the same way to perform the same function ➔ Cells, tissues, organs and body systems form a hierarchy of subsystems that are integrated in a multicellular living organisms Benefits of cell specialisation ➔ Every cell in a multicellular organism is specialised for a specific function ◆ Specialised cells focus on specific tasks, improving efficiency and energy conservation. ◆ Possess a specialised structures and metabolism ◆ Allow faster evolution in specific tasks A2.2.14* Evolution of Multicellularity Multicellularity (an organism composed of many cells) ➔ In a multicellular organism, the cells specialise and lose the ability to live independently ➔ Exists in all animals, plants, most/many but not all fungi and algae Evolution of multicellularity ➔ Multicellularity has evolved independently many times in eukaryotes ➔ It has occurred repeatedly throughout the history of life Steps in the evolution of multicellularity: 1. Formation of cellular clusters form single cells a. Independent cells come together as a group b. Cell division and daughter cells fail to separate resulting in an aggregate of identical cells. 2. Differentiation of the cells within the cluster for specialised functions Note: Early steps in the evolution of multicellularity remain uncertain due to limited fossil evidence from hundreds of millions of years ago and ongoing research. Aggregation of unicellular organisms today ➔ Some bacteria generate multicellular “biofilms” on surfaces ➔ Biofilm destruction: Cells have an incentive to not produce the flue responsible for folding them together, leading to biofilm destruction Selective Advantages of clustered cells over independent cells ➔ Predation: a selective pressure that is hypothesised to lead to multicellularity ➔ Once clusters of cells have been established, cells begin to serve specialized functions (differentiation of cells). B Form and function B1.2: Proteins B1.2.1 Generalised structure of an amino acid ➔ Proteins: polymers (polypeptide) made of monomers (amino acids) ◆ Function: Enzymes (pepsin, DNA polymerase) Structure (keratin, collagen) Carriers and transport (haemoglobin, aquaporin) Cell communication (signals, receptors) Defence (antibodies) Movement (actin and myosin) Storage (bean seed proteins) ◆ 20 different amino acids ◆ Polymer = polypeptide Protein can be one or more polypeptide chains folded and bonded together Large and complex molecules Complex 3D shape ➔ Amino acids structure Alpha carbon Amini group: NH2 Carboxyl group: -COOH R group (side chain): different for every amino acid B1.2.2: Condensation reactions forming dipeptides and longer chains of amino acids ➔ Amino acids are linked together by condensation reaction and peptide bonds ◆ Remove water to form peptide bonds ➔ Polypeptides: polymers (chains) of amino acids, can contain many amino acids ◆ Amino acids are covalently joined together and in condensation and can be broken down by hydrolysis reactions ➔ Oligopeptide: a peptide comprising a small number of amino acids (10 or fewer) ➔ Dipeptide: a molecule of two amino acids joined together by peptide ➔ Condensation: builds polymer/ large molecule (released H2O) ➔ Hydrolysis: breaks polymers into monomers or large molecules (addition of water) B1.2.3: Dietary requirements for amino acids ➔ Comparison of amino acid sources: ◆ Plants can make amino acids by photosynthesis ◆ animal obtain acids from their food they consume ➔ 9 of 20 amino acids are essential in humans ➔ Essential amino acids: one cannot be synthesised in sufficient quantities by the animal so must be obtained from the diet. ➔ Non-essential amino acids: can be synthesised by an animal using metabolic pathways that transform one amino acid into another ➔ There are two types of food: ◆ Vegan diets, which exclude all animal-derived foods, can lack some essential amino acids if not planned carefully. ◆ Complete proteins: Food that contain all 9 essential amino acids, (beef, poultry, fish, egg, fairy, soy, quinoa and buckwheat) ◆ Incomplete proteins: food that contain some but not all the essential amino acids, (nuts, seeds, beans and grains) B1.2.4: Infinite variety of possible peptide chains ➔ Most natural polypeptide chains contain between 50-2000 amino acids (any number is possible) ◆ Endless possibilities for length and sequence ➔ If there are 2 and 10 amino acid, the chain is oligopeptide ➔ Sequence of amino acid in polypeptide is determined by DNA ➔ Amino acids can be combined in various combinations, forming different proteins ➔ Examples of polypeptide: ◆ Insulin (2 short polypeptides, one with 21 amino acids and other with 30) ◆ Amylase (enzyme in saliva that digests starch, single polypeptide of 496 amino acids, with a Cl- and Ca2+ ion) ◆ Titin ( part of the structure of muscle, 27-35,000 amino acids) B1.2.5: Effect of pH and temperature on protein structure ➔ Proteins have different structures and interactions depending on their R-groups (folds differently) and amino sequence (order of acids) ◆ Attraction between different R-groups means the polypeptide chain folds in different ways ◆ Changing the structure changes the function ➔ The protein-folding problem ◆ Denatured proteins no longer work in their unfolded condition ◆ Proteins may be denatured by extreme change in pH or temperature (as bonds are broken so polypeptide chains unfold) ➔ Denaturation: most proteins are delicate and their 3D shape is easily damaged by changed to chemical or physical conditions ◆ Unfolding a protein, ◆ Temperature, pH, salinity may contribute to denaturation ◆ Destroys functionality ➔ Too high or low of a pH or high temperature, the enzyme will start to denature (change the shape and no longer work) ➔ Heat ◆ Breaks intermolecular bonds or interactions between amino acids ◆ Denaturation point varies for each protein ➔ Extreme pH ◆ Optimal pH varies ◆ Higher or lower breaks ionic bonds or cause new interaction Enzymes ➔ Enzymes are complementary to a specific substrate, but as they heat above the optimum temperature, the enzyme loses its shape and denatures ➔ Disruptions in homeostasis can prevent enzymes from functioning ➔ Changes in temperature and pH can break hydrogen bonds ➔ Too high or low of a Ph or high temperature, the enzyme will start to denature (change the shape and no longer work) ➔ Describe graph ◆ As the temperature is increasing, the percentage active enzyme also increases ◆ Until the temperature of 47°C it reaches its optimum temperature ◆ After it reaches its optimum temperature, the percentage active enzyme decreases as the temperature increases ◆ As ↑ temp = more kinetic energy = more successful collision between particles = faster reaction ◆ ↑ temp is breaking the hydrogen bonds, which will lead to denature and lose its shape and taking down the reaction Renaturation: proteins an regain their native state by folding back to the original conformation ➔ Chaperonins: protein molecules that assists in the proper folding of other proteins B1.2.6* Chemical diversity in the R-groups of amino acids as a basis for the immense diversity in protein form and function ➔ 20 amino acids are commonly found in proteins ➔ R-groups determine the folding pattern and functionality of proteins ◆ Hydrophobic (non polar)or hydrophilic (polar or charged/ acidic or basic) B1.2.7* Impact of primary structure on the conformation of protein ➔ The structure of protein has 4 levels of complexity: primary, secondary, tertiary, and quaternary ➔ Primary structure: the linear sequence of amino acids in a polypeptide ➔ Backbone: the repeating sequence of atoms linked by covalent bonds ◆ (-C-C-N-C-C-N-) and so on ➔ Confirmation: the 3D arrangement of its atoms in a polypeptide or protein ◆ Determines their functions and through this the behaviour of cells ➔ Artificial intelligence (AI) has been used to speed up the process of discovering protein conformation, since the 1970s, 180,000 proteins have been determined B1.2.8* Pleating and coiling of secondary structure of proteins - Describe the secondary structure of a protein, including the type and location of the bonds involved. - Identify the alpha-helix and beta-pleated sheet in images of protein structure. ➔ Secondary structure: the way a polypeptide folds in a repeating arrangement ◆ Occurs due to weak hydrogen bonds Hydrogen bonds form between carboxyl (C=O) groups and amino (N-H) groups, polar Usually form between non-adjacent amino acids resulting in a change in shape of the linear polypeptide chain ➔ Two shapes that can form ◆ Alpha-helix (α-helix) ◆ Beta-pleated sheet (or β-pleated sheet) B1.2.9* Dependence of tertiary structure on hydrogen bonds, ionic bonds, disulfide covalent bonds and hydrophobic interactions ➔ Primary & secondary structures might remain, but further folding occurs ➔ Tertiary structure: folding of a whole polypeptide chain into a 3D structure ◆ Due to interactions between R-groups of different amino acids ➔ Four main types of interactions: ◆ Ionic bonds between positively charged and negatively charged R-groups NH+ and COO- groups ◆ Hydrogen bonds between polar R-groups ◆ Disulfide bonds between cysteine, covalent bond (strongest of all interactions) ◆ Hydrophobic interactions between any of the non-polar R-groups B1.2.10* Effect of polar and non-polar amino acids on tertiary structure of proteins ➔ Amino acids in proteins can be divided into two broad categories: ◆ Non-polar and therefore hydrophobic ◆ Polar or charged and therefore hydrophilic ➔ Soluble globular proteins: ◆ Need to be soluble in water to function in cytoplasm or extracellular aqueous environments. ◆ Have hydrophilic amino acids on surface to interact with water ◆ Have hydrophobic amino acids in interior to stabilise tertiary structure ➔ Integral membrane protein ◆ Have hydrophobic amino acids on outer surface, allows them to embed in the non-polar core of the membrane ◆ Have hydrophilic regions side and outside cell to interact with aqueous solutions ◆ In transmembrane proteins, the hydrophobic region forms a “belt” with hydrophilic regions on either side This arrangement stabilises the protein’s structure and positioning in the membrane ➔ Channel proteins ◆ Allow hydrophilic solutes/water to diffuse across the hydrophobic membrane ◆ Transmembrane: proteins that span the membrane (need to be polar in some parts and non-polar in others) ◆ This central hydrophilic channel or pore allows selective passage of specific ions/molecules B1.2.11* Quaternary structure of non-conjugated and conjugated proteins ➔ More than one polypeptide chain bonded together ◆ Only then does polypeptide become functional protein ◆ Hydrophobic interactions ➔ Non-conjugated protein: consists of polypeptide chains only ◆ Insulin: 2 polypeptide linked by disulfide bonds ◆ Collagen: consists of 3 polypeptides wound together to form a rope-like structure with high tensile strength ➔ Conjugated protein: consists of polypeptide chains + non-polypeptide parts ◆ Haemoglobin: 4 polypeptide chains, each associated with a haem group (binds oxygen, allowing protein to transport oxygen) B1.2.12* Relationship of form and function in globular and fibrous proteins ➔ Fibrous vs. globular proteins Properties Fibrous protein Globular protein Shape Long and narrow rounded/ spherical Role Structural (strength and support) Functional (catalytic, transport, etc.) Solubility (generally) insoluble in water (generally) soluble in water Sequence Repetitive amino acid sequence Irregular amino acid sequence Stability Less sensitive to changes in heat, More sensitive to changes in pH, etc. heat, pH, etc. examples Collagen, myosin, fibrin, actin, Catalase, haemoglobin, insulin, keratin, elastin immunoglobulin ➔ Fibrous protein: quaternary proteins that are and not highly folded ◆ Have a role of support, protection and shape ◆ Made up of single type of repeating secondary structure & long strands or sheets ◆ Example: collagen ➔ Globular protein: ◆ Have a role of regulation and catalysis ◆ Made up of several types of secondary structure ◆ From compact shapes ◆ Example: Enzymes such as alcohol dehydrogenase ➔ Collagen ◆ Provides structural support to the extracellular space of connective tissues ◆ Due to its rigidity and resistance to stretching, it is the perfect matrix for skin, tendons, bones and ligaments ◆ There are 38 various types of collagen that have been discovered , but by far, the most common are types I through IV, with type I comprising over 90% of the collagen in the human body ◆ Your body produces less collagen as you age, and existing collagen breaks down at a faster rate ➔ Insulin ◆ A small globular protein with A and B polypeptide chains ◆ Produced by pancreas, regulates glucose movement into cells ◆ Released into bloodstream, binds to specific receptors B2.1: Carbonates and lipids B2.1.1 Lipid as bilayers as the basis of cell membranes Phospholipids bilayer: phospholipid form bilayer in water due to amphipathic properties of phospholipids molecules Phospholipids ➔ Amphipathic consists of both hydrophilic and hydrophobic regions ➔ Polar phosphate head is hydrophilic is attracted to water ➔ Non polar fatty acid tails, which are composed of hydrocarbon chains, are hydrophobic Membrane bound organelles ➔ Single membrane bound (sacs enclosed by a single phospholipid bilayer) ◆ Vesicles and vacuoles, RER & SER, golgi apparatus, lysosomes ➔ Double membrane bound (structures enclosed by two phospholipid bilayers ◆ Nucleus, mitochondria, chloroplasts B2.1.2 Lipid bilayers as barriers Selective permeability of phospholipid bilayer: ➔ Selective permeability: allows only some substances to pass through ➔ Permeable: ability to allow particles to pass through ➔ Plasma membrane regulates the transport of materials entering and exiting the cell Factors influencing Permeability: 1. Molecule size: a. Larger molecules are less permeable (e.g. macromolecules such as proteins or polysaccharides) b. Smaller molecules are more permeable (e.g. water) 2. Hydrophobicity or Hydrophilicity a. Nonpolar, hydrophobic molecules can pass through the lipid bilayer more easily than polar, hydrophilic molecules. (e.g. oxygen, carbon dioxide) 3. Charge and polarity a. Large polar or charged atoms, ionic molecules, and hydrophilic molecules cannot pass + + − through the phospholipid bilayer. (Ions such as 𝐾 , 𝑁𝑎 , 𝐶𝑙 ) B2.1.3 Simple diffusion across membranes Simple diffusion: the net movement of molecules from areas of higher concentration to a areas of lower concentration (along a concentration gradient) until equilibrium is reached ➔ Small and non-polar molecules will be able to diffuse freely across the cell membrane (oxygen, carbon dioxide, glycerol) ➔ Examples of simple diffusion: Alveoli, intestine, oxygen The rate of diffusion can be influenced by a number of factors, including: ◆ Temperature (affects kinetic energy of particles in solution) ◆ Molecular size (larger particles are subjected to greater resistance within a fluid medium) ◆ Steepness of gradient (rate of diffusion will be greater with a higher concentration gradient) B2.1.4 Integral and peripheral proteins in membranes ➔ Membrane Proteins: ◆ Integral proteins: permanently embedded in phospholipid bilayer ◆ Peripheral proteins: temporarily associated with membrane periphery ➔ Enzymes are located in membranes, are proteins that catalyse (speed up) chemical reactions for various metabolic pathways ➔ Functions: ◆ Junction - connect two cells together ◆ Enzymes - localised reactions ◆ Transport - for facilitated diffusion and active transport ◆ Recognition - markers for self identification ◆ Anchorage - act as attachment points for cytoskeleton ◆ Transduction - receptors for peptide hormones B2.1.5: Movement of water molecules across membrane by osmosis and the role of aquaporins Osmosis: The net movement of free water molecules across a semipermeable membrane from a region of high water potential to a region of lower water potential. (Low solute zone → high solute zone) ➔ Solute concentration: solutions can be classified according to concentration ◆ Hypertonic: solutions with high osmolarity, higher solute concentration (gain water) ◆ Hypotonic: Solutions with low osmolarity, lower solute concentration, with a higher water potential than the cytoplasm (lose water) ◆ Isotonic: Solutions have the same osmolarity, with same solute concentration, with the same water potential as the cytoplasm (no net water flow, main constant water level) ➔ Osmosis effects: ◆ When cell is placed in a hypertonic solution, the loss of water causes the cell to shrivel The loss of cytoplasmic volume causes the membrane to from a ruffled shape (crenation) ◆ When a cell is places in in a hypotonic solution, the gain of water causes the cell to swell As the plasma membrane is fluid, it will stretch until the cell eventually bursts (cell lysis) ➔ Osmosis effects in plant cells ◆ In a hypertonic solution, the membrane will recede away from the cell wall (plasmolysis) ◆ In a hypotonic solution, the cell membrane will be pressed against the cell wall (turgor) Isotonicity For organisms to survive, the fluid surrounding cells must remain isotonic to prevent damage - There are several natural and artificial ways of maintaining his homeostatic requirement Living adaptation - Unicellular organisms may contain contractile vacuoles to regulate their water levels - Multicellular organisms may use aquaporins Medical applications: - Organs used in medical procedures must be bathed in isotonic solutions until transplant - Isotonic solutions can also be delivered intravenously to restore fluid levels in patients. Contractile Vacuoles Unicellular organisms (such as protists) nay use contractile to expel excess water - Excess water is absorb\bed into the contractile vesicle, causing it to swell (diastole) - The vacuole then fuses to the plasma membrane and contracts, expelling water (systole) Aquaporins Multicellular organisms may contain integral proteins (aquaporins) within their plasma membrane - Acts as selective water channels Facilitate a faster rate of water transport in response to any changes in solute concentration - The quantity of aquaporins can be regulated via gene expression to help control and maintain the osmotic conditions within a multicellular organism B2.1.6: Channel proteins for facilitated diffusion Facilitated diffusion ➔ Utilised by molecules that are unable to freely cross the phospholipid bilayer (e.g. large, polar/ charged molecules and ions) ➔ This process is mediated by two distinct types of transport proteins - channel proteins and carrier proteins 1. Channel protein ❖ An integral, transmembrane protein with a pore that connects the cytoplasm to the aqueous solution outside the cell. ❖ Only move molecules along the concentration gradient (no energy needed) ❖ Have a much faster rate than carrier proteins ❖ Cells can select which hydrophilic substance to diffuse and can open and close (temporary permeability). 2. Carrier proteins ❖ integral glycoproteins bind a solute and undergo a conformational change to translocate the solute (only bind specific molecules). ❖ Much slower rate of transport than protein channels ❖ Solutes move down the concentration gradient and don’t use extra cellular energy to move B2.1.7: Pump proteins for active transport Active transport: it uses ATP to pump molecules against the concentration gradient (from low to high) ➔ Protein pumps are required for the translocation ➔ involves the binding of a molecule to a specific protein pump - which is hydrolysed by ATP ◆ ATP hydrolysis cases a conformational change in the pump, causing the molecule to be translocated ◆ The pump then returns to its original conformation B2.1.8: Selectivity in membrane permeability B2.1.9: Structure and function of glycoproteins and glycolipids ➔ Glycoprotein: conjugated proteins with carbohydrate as non-polypeptide component ➔ Glycolipid: molecules consisting of carbohydrates linked to lipid ➔ Glycoproteins and glycolipids are only found on the extracellular surface of plasma membranes where they function in cell adhesion and cell recognition ◆ cell to cell recognition: help immune system to distinguish between self and non self cells, so pathogens and foreign tissue can be recognised and destroyed ◆ Cell adhesion: form a carbohydrate-rich layer on the outer surface of the plasma membrane of animal cells (glycocalyx), glycocalyx of adjacent cells can fuse, binding the cells together and preventing from falling apart B2.1.10: Fluid mosaic model of membrane structure B2.1.11* Relationships between fatty acid composition of lipid bilayers and their fluidity Membrane Fluidity ➔ Importance of membrane fluidity ◆ Enable molecules to diffuse through the membrane towards areas of the cell where they are needed ◆ Facilitate the interaction between proteins, which is crucial for cell signalling ◆ Enable membrane to fuse with one another during vesicle formation, endocytosis and exocytosis ◆ Ensure the even distribution of membrane molecules between daughter cells during cytokinesis. ➔ The higher temperature the more fluidity the membrane is ➔ The longer the phospholipid tails, the less fluid the membrane will be ◆ Palmitic acid (more fluid) ◆ Linoleic acid (less fluid) Higher temperature Lower temperature Lower viscosity (more fluid/ flexible) High viscosity (not as fluid/flexible) Less densely packed Densely packed Won't hold shape More rigid, may break Not permeable enough Too permeable Saturated Fatty Acid Tails Unsaturated Fatty Acid Tails No C-C double bonds At least one C-C double bonds Straight chain Branched chain Pack tightly together (strong intermolecular bonds) Packed loosely together (weak intermolecular bonds) Decrease membrane fluidity Increase membrane fluidity Decrease membrane permeability Increase membrane permeability Higher melting point Lower melting point Effect of temperature on membrane fluidity - At low temperature, organisms increase unsaturation of fatty acids - At high temperature, organisms increase saturation of fatty acids - The saturation of membrane lipids can vary within the body of a single organism B2.1.12* Cholesterol and membrane fluidity in animal cells ➔ Cell membrane of animal cells also contains cholesterol Structure of cholesterol ➔ Cholesterol is a lipid ➔ Consisting of a hydroxyl group linked at one end, four linked hydrocarbon rings, a hydrocarbon tail linked to the other end ➔ Cholesterol is an amphipathic molecule, containing both hydrophilic and hydrophobic parts ◆ Hydrophilic: the hydroxyl group ◆ Hydrophobic: the hydrocarbon rings and the hydrocarbon tail Temperature affects membrane fluidity ➔ Membrane fluidity: the viscosity of the lipid bilayer of a cell membrane ➔ High viscosity have low temperature, low viscosity have high temperature Function of cholesterol in the membrane ➔ Cholesterol acts as a modulator (adjustor) of membrane fluidity ◆ At high temperatures, restrains the movement of phospholipids fatty acids, stabilising the membrane by making it less fluid and reducing its permeability to small molecules ◆ At low temperatures, prevents stiffening of the membrane by preventing tight packing of the fatty acid chains, maintaining membrane fluidity B2.1.13* Membrane fluidity and the fusion and formation of vesicles ➔ Endocytosis: The cell activity transports water and solutes into the cell by engulfing them into vesicles formed from the cell membrane. ◆ Engulf molecules → the vesicle is pinches off → cell membrane fuse together ➔ Examples of endocytosis ◆ Macrophages (a type of white blood cell) can engulf pathogens when fighting infection ◆ Single celled organism (amoeba) can engulf other organisms as a food source ◆ Proteins (antibiotics) are taken from the mother’s blood at the placenta to be given to the fetus ◆ Important step in the theory of endosymbiosis ➔ Exocytosis: The cell activity transports molecules out of the cell into the extracellular space ➔ Example of exocytosis: ◆ Release of neurotransmitters from a presynaptic membrane ◆ Secretion of hormones from endocrine glands, such as insulin and glucagon from the pancreas ◆ Removal of excess water from the contractile vacuole of some unicellular organisms ◆ Release of cortical granules from egg cells during fertilisation, preventing polyspermy. B2.1.17* adhesion of cells to form tissues Tissues ➔ The cells in a tissue must stick together and communicate with each other to coordinate function ➔ 4 basic type of tissues in humans: ◆ Connective tissue: supports other tissues and binds them together (bone, blood, and lymph tissues) ◆ Epithelial tissue: provides a covering (skin, the lining of the various passage inside the body) ◆ Muscle tissue: includes striated muscles that move the skeleton, and smooth muscle, such as the muscles that surround the stomach ◆ Nerve tissue: is made up of neurons and is used to carry electric impulses to and from various parts of the body Cell adhesion in tissues ➔ Cell in a tissue form cell junction which provide for: ◆ adhesion between neighbouring cells ◆ anchoring (connect) of a cell to the extracellular matrix (environment outside of the cell) Cell adhesion molecules (CAMs): proteins that embedded within the plasma membrane that bind cells with other cells or with the extracellular matrix ➔ Helps cells stick to each other and to their surroundings ➔ Difference forms of CAMS are used for different type of cell junctions (gap) (different cams are needed for different cell to stick to their respective cells or sites) Loss of cell adhesion in cancer ➔ Cancer metastasis: the spread of cancer cells to other tissues and organs ➔ The loss of the cell adhesion allows malignant tumour cells to dissociate from the primary tumour mass during metastasis ◆ When cancer cells lose their CAMs protein, they will dissociate (leave) their primary tumour mass (original site), this is metastasis. B2.2: Organelles and compartmentalization B2.2.1: Organelles as Discrete Subunits of Cells that are Adapted to Perform Specific Functions ➔ Organelles are discrete structures in cells that are adapted to perform a specific function ➔ Can be solid structures of membrane-bound sacs enclosing specific combinations of dissolved solutes. No membrane Single membrane-bound Double membrane-bound Not enclosed by phospholipid Enclosed by a single Enclosed by two phospholipid bilayers phospholipid bilayer bilayer Ribosomes, Centrioles, Vesicles and Vacuoles, RER, Nucleus, Mitochondria, Nucleolus SER, Golgi apparatus, lysosomes Chloroplast ➔ The following don’t perform specific functions, don’t reach the requirements of an organelle ◆ Cell wall: outside the cell membrane = outside the boundary of cell (extracellular) ◆ Cytoplasm: Not specialised ◆ Cytoskeleton: not a discrete structure as it is composed of many different types of filament and tubules ➔ The study of the functions of organelles only became possible after ultracentrifuges had been invented when cell fractionation had been developed. B2.2.3: Advantages of Compartmentalization in Cytoplasm of Cells ➔ Eukaryotic cells evolved from infolding and endosymbiosis to become super-efficient spaces where multiple activities happen ➔ They contain many membrane-bound organelles ➔ Membranes act as barriers between aqueous solutions and are only permeable to a small number of substances. ➔ The membrane around an organelle creates a compartment with different controlled conditions inside ➔ Advantages: tailored to the specific functions of the organelle ◆ Enzymes and metabolites can be concentrated in a small space ◆ Harmful substances are isolated ◆ Conditions such as pH can be maintained at an optimal value ◆ Membranes become dense with proteins for specific processes ➔ Examples of compartmentalization include ◆ Lysosomes: are compartmentalised to prevent uncontrolled digestion of the contents of the cytoplasm ◆ Phagocytic vacuole: ensures the cell organelles are prevented from being engulfed through phagocytosis (engulfing a larger particle with its plasma membrane) B2.2.6*: Functional Benefits of the Double Membrane of the Nucleus ➔ Eukaryotic cells have a nucleus, which serves as an information processing and administrative centre of the cell. ➔ Two major functions include: ◆ It stores genetic material ◆ Via gene expression, the nucleus coordinates the cell’s activities (growth, metabolism, protein synthesis, and division) ➔ Nucleoplasm: the semifluid matrix found inside the nucleus ➔ Chromatin: a less condensed form of DNA within the nucleus that forms chromosomes during the prophase of mitosis and meiosis ➔ Nucleoli: one or more in the nucleus, organelles that synthesize ribosomes ➔ The nuclear envelope ◆ The nucleus is surrounded by a system, of concentric membranes (inner and outer) ◆ Outer nuclear membrane: continuous with the endoplasmic reticulum ◆ The membranes are phospholipid bilayers, permeable to small nonpolar molecules ➔ Nuclear pore ◆ Nuclear pores complexes create a selective passageway in which molecules travel between the nucleus and cytoplasm ◆ About 30 times the size of a ribosome (in vertebrates, composes 50 to 100 proteins) ◆ Much larger than channel proteins ◆ A double membrane structure allows the formation of large nuclear pores (will be damaged from the big hole) ➔ Functions of nuclear pore ◆ Proteins responsible for all aspects of genome structure and function are synthesized at ribosomes in the cytoplasm and must be imported into the nucleus Includes histones, helicase, and DNA polymerases and others ◆ mRNA and tRNA are formed in the nucleus during transcription and must be exported to the cytoplasm to function in translation ◆ Ribosomes are synthesized in the nucleolus and must be exported to the ER or cytoplasm ➔ A unique feature of the nucleus is that it disassembles and re-forms each time most cells divide ➔ During prophase, the nuclear membrane and ER are fragmented into vesicles and moves to the edge of the cell ➔ During telophase, the vesicles are moved around the new sets of daughter chromosomes, and the nuclear membrane and ER are reformed B2.2.7*: Structure and Function of Free Ribosomes and of the Rough Endoplasmic Reticulum ➔ Ribosomes structure: made from dozens of proteins arranged on a scaffold of ribosomal RNA (rRNA) ◆ Both prokaryotic and eukaryotic ribosomes are composed of two subunits that come together to form a functioning structure ◆ Ribosomes have binding sites to which mRNA and tRNA bind during translation Large subunit has three binding sites for tRNA (A, P and E) and the small subunit has a binding site for mRNA ➔ Ribosomes function: catalyses the synthesis of polypeptides during translation ◆ “Free”= floating in the cytoplasm ◆ “Bound”= attached to RER, secreted from the cell or become integral proteins in the cell membrane ➔ Endoplasmic reticulum is part of the endomembrane system ◆ Endomembrane system is a system of compartmentalised sacs within the eukaryotic cell, work together to modify, process and ship molecules within and out of the cell ◆ Organelles of the endoplasmic reticulum function together to produce, transport and secrete proteins: Nuclear membrane → Rough ER → Transport vesicle → Golgi body → Secretory vesicle → cell membrane ➔ Rough endoplasmic reticulum: a series of connected flattened sacs with bound ribosomes ◆ Polypeptide can be transported elsewhere in the cell by a vesicle that buds off from the rER ◆ The typical destination of a vesicle from the rER is the golgi apparatus B2.2.8*: Structure and Function of the Golgi Apparatus ➔ The Golgi apparatus is part of the endomembrane system ➔ The endomembrane system is a system of compartmentalised sacs within the eukaryotic cell that work together to modify and process molecules in and out of the cell ➔ Each line of the golgi apparatus represents a phospholipid bilayer ➔ Golgi apparatus structure ◆ Composed of flattened membrane-enclosed sacs called cisternae ◆ Polypeptides transported in vesicles to Golgi → vesicles fuse with convex cis face (entry face) → transported through Golgi → exit at concave trans face (exit face) ➔ Golgi apparatus function ◆ Within the Golgi cisternae, polypeptides are modified into their functional state ◆ E.g. adding a carbohydrate to make a glycoprotein; combining with other polypeptides to form the quaternary structure of a protein ◆ There are two mechanisms: vesicle transport and cisternal maturation ◆ After polypeptides are modified, the trans face sorts, concentrates and packs proteins into vesicles for different purposes. B2.2.9*: Structure and function of vesicles in cells ➔ Formations of vesicles: a vesicle forms when the membrane bulges and pinches off ◆ Clathrins: a protein that plays major role in vesicle formation Created a coat that helps phospholipids create a round shape for the vesicle Clathrin coat is removed once the vesicle is formed ➔ Vesicle functions: ◆ Endocytic vesicles: formed by invagination of the plasma membrane around an extracellular substance during endocytosis ◆ Deliver the cargo to other organelles for further sorting or digestion E.g. phagocytes engulfing pathogens as a mechanism of infection control form an endocytic vesicle called a phagosome ➔ Vesicle fusion: the merging of a vesicle with another organelle or with part of a cell membrane, adding phospholipids to target structure, making it larger B2.3: Cell specialisation B2.3.1: Production of unspecialised cells following fertilisation and their development into specialised cells by differentiation ➔ Fertilisation: the fusion of a male and female gamete to produce a single cell (zygote) ◆ Fertilisation (male gamete + female gamete = zygote) ◆ Occurs in sexually reproducing organisms that produce with haploid gametes ➔ Embryonic development ◆ The zygote divides by mitosis to form an embryo composed of genetically identical cells. ◆ Cells in zygote and early embryo are unspecialised stem cells ➔ Cell specialisation and differentiation ◆ Cells specialise to perform functions more efficiently Can develop into specific shapes and sizes, create protein needed to carry out specific metabolic reactions ◆ Differentiation: the development of specialised structures and functions in cells It occurs when different cell types express different genes ◆ Gene expression: the process of turning gene information into function A sequence of DNA (the gene) is transcribed to form RNA which is then translated to form a protein Differentiation during Embryonic development ➔ Cell position in embryo determines differentiation pathway ➔ Signalling molecules (morphogens) indicate cell position and direct differentiation ◆ Example: retinoic acid is a morphogen that regulates growth, differentiation, and development B2.3.2: Properties of stem cells ➔ Stem cell: an undifferentiated cell ◆ Can divide endlessly ◆ Can differentiate into multiple types of cell B2.3.3: Location and function of stem cell niches in adult humans ➔ Stem cell niches: location that have stem cells and provide an environment that allows stem cells to regenerate and/or differentiate ◆ To remain undifferentiated over long periods of time, to proliferate rapidly and differentiate when required ➔ Stem cells in human tissues (e.g. marrow, skin and liver), give them considerable powers of regeneration and repair ➔ Example: bone marrow stem cell niche (multipotent) ◆ Bone marrow contains haematopoietic stem cells that produce huge numbers of red and white blood cells and platelets each day. ➔ Example: hair follicles (multipotent) ◆ Stem calls at the base of each hair divide repeatedly to generate the many cells needed for hair growth B2.3.4: Differences between totipotent, pluripotent and multipotent stem cells ➔ Totipotent: they can differentiate into any cell type ◆ E.g. Zygote ➔ Pluripotent: can differentiate into many (but not all) cell types ◆ E.g. Embryo, inner mass of a blastocyst ➔ Multipotent: can differentiate into a few different cell types ◆ E.g. Adult stem cells, hematopoietic stem cells B2.3.5: Cell size as an aspect of specialisation Cell type Description Benefit of size specialisation Sperm cell 50μm long, narrow cell with a strong Narrowness and small volume reduce flagellum for propulsion. resistance and allow sperm to swim to the egg more easily Egg cell 110μm large, spherical cells Large volume of cytoplasm, allow large quantities of food reserved to be stored in the cytoplasm Red blood cell 6-8μm in diameter, 1μm thick, small Small size and shape allow passage along in size, biconcave shaped narrow capillaries and gives a large surface area-to-volume ratio, so they can load and unload oxygen faster White blood cell B-lymphocyte are small (10μm) when The extra volume is cytoplasm with rough inactive, but increase in size (30μm) endoplasmic reticulum and golgi apparatus during active infection for protein synthesis Motor neurons A large cell body (20μm) with long Large size allows enough proteins to be narrow axon that can extend for a synthesised to maintain the immensely long metre or more (1,000,000 μm) axone, extended part can carry signals from the central nervous system to a distant muscle Striated muscle Very large cells with diameter The long narrow dimensions of the cells fibre cell (20-100μm), lengths (10cm) allow large and powerful muscle contractions B3.1: Gas Exchange B3.1.1: Gas exchange as a vital function in all organisms ➔ Ventilation: the exchange of air between the atmosphere and the lungs - achieved by the physical act of breathing ➔ Gas exchange: all organisms absorb one gas from the environment and release another one, the exchange of oxygen and carbon dioxide between the alveoli and bloodstream (via passive diffusion) ➔ Respiration: The release of energy (ATP) from organic molecules - it is enhanced by the presence of oxygen (aerobic) B3.1.2: Properties of gas exchange surfaces ➔ Gas exchange surfaces ◆ All gas exchange surface have features in common ◆ These features allow the maximum amount of gases to be exchanged across the surface in the smallest amount of time ➔ Features of gas exchange surfaces ◆ Large surface area, allow faster diffusion of gases across the surface ◆ Thin walls to ensure diffusion distances remain short ◆ Good ventilation with air so that diffusion gradients can be maintained ◆ Moist ➔ Maximising rate of diffusion 1. The specialised respiratory surface must have a large surface area to volume ratio (SA:V) 2. The specialised respiratory surface must be moist 3. The specialised respiratory surface must be thin 4. There must be a large concentration gradient across the respiratory surface B3.1.3: Maintenance of concentration gradient at exchange surfaces in animals ➔ Large concentration gradient ◆ For a concentration gradient to be large/steep, there must be a very high concentration of molecules on one side of the respiratory surface, and a very low concentration of the same molecule on the other side ◆ The larger the concentration gradient, the higher the rate of diffusion ➔ Maintenance of concentration gradients: single celled organisms ◆ Chlamydomonas is a single-celled organism, found in freshwater, spherical in shape, diameter of 20μm. Oxygen can diffuse across the cell wall and cell surface membrane of chlamydomonas ◆ The maximum distance that oxygen molecules would have to diffuse to reach the centre of a chlamydomonas is 10μm, this takes 100 milliseconds ➔ Maintenance of concentration gradients: root hairs ◆ Root hair cells in plants have a root hair that increases the surface area (SA) so the rate of water uptake by osmosis in greater (can absorb more water and ions than if SA were lower) ◆ An extension of the cytoplasm, increasing the surface area of the cell in contact with the soil to maximise absorption of water and minerals ➔ Maintenance of concentration gradients: Extensive capillary network ◆ The walls of capillaries only one cell thick and these cells are flattened, keeping the diffusion distance for gases short ◆ The constant flow of blood through the capillaries means that oxygenated blood is brought away from the alveoli and deoxygenated blood is brought to them ◆ This maintains the concentration gradient necessary for gas exchange to occur ➔ Maintenance of concentration gradients: Fish gills ◆ Fish gills are adapted to directly extract oxygen from water as they have a large capillary network ◆ The extensive capillary system that covers the gills ensures that the blood flow is in the opposite direction to the flow of water - it is a counter-current system ◆ The counter-current system ensures the concentration gradient is maintained along the whole length of the capillary B3.1.4: Adaptation of mammalian lungs for gas exchange ➔ All mammals use lungs for gas exchange ➔ Air in trachea → left and right bronchi/brochus → branches into bronchioles → branches into alveoli (groups of 5 to 6) ◆ A pulmonary alveolus has a diameter of 0.2mm to 0.5mm, but its wall is a single layer of cells, much is only 0.2μm thick They are surrounded by a dense and thin capillary network Air and blood are very short distances apart About 300 million in a pair of adult lungs ➔ Surfactant: reduces surface tension between air-liquid interface so alveoli don’t collapse ➔ Pleural membrane: lubricant ➔ Adaptation in mammalian lungs ◆ A ventilation mechanism also helps to maintain a concentration gradient across an exchange surface ◆ Ventilation in lungs helps to ensure that there is always a high concentration of oxygen in the alveoli than in the blood ◆ The movements involved in breathing causes the air in the alveoli to change. Exhale removes air with low oxygen and high carbon dioxide Inhale replaces air with high oxygen an low carbon dioxide B3.1.5 Ventilation of the lungs ➔ The trachea and bronchi have cartilage in their ways to ensure they remain open ➔ The bronchioles have smooth muscle fibres in their ways, allowing the width to vary ➔ Ventilation involves basic physics ◆ Large volume = lower pressure and vice versa ➔ Muscles lengthen when they relax, caused by the contraction of the “opposite muscle” ◆ Muscles that work together in the “opposite” way are called antagonistic muscles ➔ The internal and external intercostal muscles and the diaphragm and the abdominal muscles are antagonistic muscles used in ventilation Inspiration/ inhalation Expiration/ exhalation Diaphragm Contracts & flatten Relax, domed shape Abdomen wall Relax, allow pressure from diaphragm Contract, pushing the abdominal muscles to push it outwards organs and diaphragm upwards (during forced expiration) External Contract, pulling the ribcage upwards Relax, pulled into their elongated intercostal and outwards state muscles Internal Relax, pulled into their elongated state Contract, pulling ribcage inward intercostal and downwards (forced expiration) muscles Volumes or Volume of thorax increases, pressure Volume of thorax decreases, pressures decreases, sucking air in pressure decreases, forcing air out Rib Cage Upward and outward (expanding) Downward and inward movement (contracting) B3.1.6: Measurement of lung volumes ➔ Ventilation rate: the number of times that air is drawn in or expelled per minute ➔ Tidal volume: the volume of fresh air inhaled or the volume stale air exhaled with each ventilation ➔ Vital capacity: the total volume of air that can be exhaled after a maximum inhalation ➔ Inspiratory reserve volume: the amount of air a person can inhale forcefully after normal tidal inhalation ➔ Expiratory reserve volume: the amount of air a person can exhale forcefully after normal tidal exhalation B3.1.7: Adaptations for gas exchange in leaves ➔ Leaves are adapted for photosynthesis and gaseous exchange ◆ Large surface area Flat and thin, spongy mesophyll ◆ Contain openings Stomata to allow carbon dioxide into and oxygen out of leaves ◆ Moist In the spongy mesophyll ➔ Chloroplast need a supply of carbon dioxide for photosynthesis Adaption Purpose Epidermis is the thin and transparent To allow more light to reach the palisade cells Thin cuticle made of wax Air spaces allow gases to diffuse through the leaf Palisade cell layer at top of leaf To protect the leaf from infection and prevent water loss without blocking out light Spongy layer (moist) To absorb all the available light Palisade cells contain many chloroplasts To absorb more light and increase the rate of photosynthesis B3.1.8: Distribution of tissues in a leaf B3.1.9: Transpiration as a consequence of gas exchange in a leaf ➔ Exchange of oxygen and carbon dioxide only works efficiently if the gas exchange surface is moist ➔ Transpiration: the process by which plants give off water vapour through the stomata in their leaves ◆ Carbon dioxide enters, while water and oxygen exit, through a leaf’s stomata ◆ Does not occur when the stomata are closed neither does photosynthesis because there is no CO2 intake ◆ Provides the water for photosynthesis, transports mineral ions, cools the leaf as water evaporates, provides water that keeps the cells turgid ➔ Transpiration rates are affected by environmental factors Factor Change in factor Explanation Temperature Increase Increased molecular movement = more kinetic energy = higher rate of diffusion Humidity Decrease Reduces concentration gradient = slower water movement = slower diffusion Air movement Increase Removes water vapour = higher concentration gradient = more water diffusion Light intensity Increase Increase rate of photosynthesis = stomata open = diffusion increase ➔ Potometer measure the rate of transpiration ➔ Stomata ◆ Stomata control water loss and gas exchange by opening (photosynthesis) and closing ◆ Allow water vapour and oxygen out of the leaf and carbon dioxide in ◆ Guard cells are adapted to their function by allowing gas exchange and controlling water loss within the leaf When water enters the become swollen When water leaves they become flaccid ➔ Preventing excess water loss ◆ Hair around stomata traps the water and regulates water loss to maintain low concentration gradient (high water potential) ◆ Waxy cuticle on leaf which is impermeable to water ◆ Thick leaves = reduce water loss ◆ Spines/hairs = trapping moist air ◆ Stomata closed at certain times ◆ Stomata may be sunken and found in pits ◆ Leaves curl to trap moist air B3.1.10: Stomatal density ➔ Stomatal density: the number of stomata per unit area of leaf surface ➔ Two techniques: ◆ peel of epidermis and mount on slide ◆ paint/dry/peel nail polish to make a cast and study it under a microscope 𝑚𝑒𝑎𝑛 𝑛𝑢𝑚𝑏𝑒𝑟 𝑜𝑓 𝑠𝑡𝑜𝑚𝑎𝑡𝑎 ➔ Stomatal density (mm-2) = 2 𝑎𝑟𝑒𝑎 𝑜𝑓 𝑓𝑖𝑒𝑙𝑑 𝑜𝑓 𝑣𝑖𝑒𝑤 (𝑚𝑚 ) B3.1.11: Adaptions for foetal and adult haemoglobin for the transport of oxygen ➔ Haemoglobin: the oxygen transport protein carried by red blood cells ◆ Transport oxygen from the lungs to body and return CO2 from tissues back to the lungs (exhalation) ◆ It is a globular protein consisting four tightly packed polypeptide chains ◆ Changes shape based on the number of oxygen bound to it, also alters its affinity to oxygen Oxygen binding causes a conformational change, increasing affinity (attraction) for oxygen ◆ ➔ Affinity: measure of how strongly a molecule binds to another ➔ Haemoglobin is an allosteric protein ◆ This means that the binding of oxygen to one of the subunits is affected by its interactions with other subunits ➔ Oxygen saturation of haemoglobin is not directly proportional to oxygen concentration. Instead it changes from saturated to unsaturated over a relatively narrow range of concentrations ➔ Carbon dioxide bonding ◆ If something like carbon monoxide that has a stronger affinity for oxygen than haemoglobin exists in lungs it will steal all the oxygen ◆ Will cause cells to suffocate, lowers affinity for oxygen ➔ Oxygen affinity of haemoglobin increases as the number of oxygen bound increases ◆ Known as cooperativity ◆ When no oxygen is bound, haemoglobin is in tense state (T-state), with low affinity ◆ When one oxygen is bounded, shape alters to relaxed state (R-state), higher affinity ➔ Myoglobin vs Haemoglobin ◆ Myoglobin release oxygen in response to the muscles immediate needs ◆ Myoglobin is used to store oxygen while haemoglobin is used to transport ◆ Haemoglobin cooperative binding allows for respond to changes in oxygen availability ➔ Cooperative binding ◆ In red blood cells, the oxygen-binding curve for haemoglobin displays an “S” shaped called a sigmoidal curve ◆ When one site binds oxygen, the probability that the remaining unoccupied sites that will bind to oxygen will increase ➔ Maternal and Foetal haemoglobin ◆ Foetal haemoglobin has a greater affinity for oxygen of low partial pressures of oxygen than adult haemoglobin (curves shift left) B3.1.12: Bohr shift ➔ Bohr shift: a phenomenon where the the affinity of haemoglobin for oxygen is influences by pH and the presence of CO2 ➔ ↑ need for ATP = ↑ respiration = ↑ CO2 = ↓ pH = carbaminohemoglobin ◆ Carbaminohaemoglobin has a lower affinity for oxygen, so it gives up oxygen at a higher partial pressure ◆ When CO2 levels are high, it forms carbonic acid in red blood cell, lowering the pH ◆ Reduces pH decreases haemoglobin's affinity for O2, promoting the unloading of oxygen in tissues where it is needed the most ➔ Two mechanisms cause decrease in affinity ◆ CO2 + H2O → H+ + HCO3- Reduces pH of blood = reduces affinity of haemoglobin for oxygen ◆ Haemoglobin + 4CO2 ⇆ carbaminohemoglobin Reduces affinity of haemoglobin for oxygen ➔ Reduced affinity of haemoglobin for oxygen in high CO2 concentration shifts the oxygen dissociation curve to the left - Bohr shift ➔ Respiration in tissues gives high CO2 concentration, high temperature, high H+ concentration ◆ Dissociation curve shifts right ◆ These are the conditions in respiring tissues from dissociation of bicarbonate ions or production of lactic acid ◆ Lower oxygen affinity (gives out more oxygen to needed areas) ➔ Bohr effect on maternal and foetal haemoglobin B3.1.13: Oxygen dissociation curves as a means of representing the affinity of haemoglobin for oxygen at different oxygen concentrations C Interaction and Interdependence C1.1: Enzymes and Metabolism C1.1.1 Enzymes as catalysts ➔ Energy needs of life: synthesis of macromolecules, active transport, movement ➔ Bioenergetics: transformation of energy through an organism Catalyst: a substance that increases the rate of chemical reaction but is not changed by the reaction ➔ Enzymes: biological catalyst, made by living cells (speed up biochemical reactions) ◆ Substrates → products (enzymes convert substrates into products) ➔ It does not change the outcome of the reaction, do not become part of the reaction ➔ The catalyst is not used up it may be reused ➔ The amount of product and reactants remains constant C1.1.2: Role of enzymes in metabolism ➔ Metabolism: chemical changes that take place in a cell or an organism ➔ Enzymes: ◆ Biological catalysts in living things, most are proteins, required for most biological reaction, control reactions of life, highly specific ◆ Facilitate chemical reactions (↑ rate of reaction without being consumed, ↓ activation energy) ➔ Most metabolic reactions are catalysed by an enzyme, showing specificity in catalytic activity ◆ Each enzyme catalyses (speed up) one specific reaction or group of reactions ◆ Allow cells to control metabolism (enzymes are only produced for reactions that are required in the cell) C1.1.3: Anabolic and catabolic reactions Metabolism has two parts: anabolism and catabolism ➔ Anabolic reaction: build up smaller molecules into larger ones (2->1), energy required ◆ E.g. photosynthesis (CO2 + H2O + other small molecules to produce larger molecules, with light as energy source) ◆ Macromolecules are produced from monomers, using energy from ATP, condensation reaction (water is a by-product) ◆ DNA synthesis (replication) ➔ Catabolic reaction: breaks down larger molecules into smaller ones (1->2), energy is released ◆ E.g. Digestion of large molecules (macromolecules) such as protein, cellulose and starch into monomers by hydrolysis (water molecules are split) ◆ Cell respiration, glucose or fats are oxidised to release energy, with water and carbon dioxide as waste products. ◆ Digestion of food C1.1.4: Enzymes as globular proteins with an active site for catalysis ➔ Active site: a specific region on the enzyme’s surface where which substrate molecules will bind, catalysing reactions involving the substrate ◆ The shape and chemical properties of the active site and substrate match each other, allows the substrate to bind with the enzyme, while most other substances cannot Substrate is bound to active site, converted into products, then released, free to catalyse another reaction ➔ Enzymes: globular proteins, with precise 3D structure and chemical properties allow them to function as catalysts ◆ Composed of long chain of amino acids (polypeptide) that have folded into a very specific three-dimensional shape which contains an active site C1.1.5: Interactions between substrate and active site to allow induced-fit binding Stages of enzyme catalysis of a chemical reaction ➔ Substrate bind to enzyme → substrate is converted to products → products are released → active site is available for another molecule of substrate Induced-fit model ➔ Enzymes are highly specific and only catalyse reactions ➔ The specificity it due to the specific shape of the active site, which only fits the substrate for which it is intended C1.1.6: Role of molecular motion and substrate-active site collisions in enzyme catalysis Substrate collision ➔ Collision: the coming together of a substrate molecule and an active site ◆ Result of the random movements of both substrate and enzyme ◆ Substrate may be at any angle to the active site when the collision occurs ➔ Successful collisions: occur when the substrate molecule and the enzyme's active site happen to be correctly aligned to allow binding to take place ➔ ↑ concentration = ↓ collisions, ↓concentration = ↑ collisions C1.1.7: Relationship between the structure of the active site, enzyme-substrate specificity and denaturation ➔ Enzyme-substrate specificity: the active site of an enzyme is very specific to its substrates as it has a very precise shape. ◆ E.g. glucose, the only substrate that binds to active site of enzyme glucokinase ◆ Hexokinase can bind with any one of a group of hexose sugars ◆ Proteases have broad substrate specificity, can act on many different substrates ➔ Denaturation: an enzyme that has lost its normal three dimensional (tertiary) structure ◆ Cause of denaturation: exposure to high temperatures, extreme pH levels, salinity ➔ Effects of denaturation: ◆ The bonds (H bonds) holding the enzyme’s 3D shape are broken ◆ The enzyme becomes insoluble and forms a precipitate ◆ The active site of the enzyme changes shape and can no longer bind to the substrate ◆ The rate of reaction catalysed by the denatured enzyme will decrease ➔ Very few human enzymes can function at temperatures above 50°C ◆ Humans maintain a body temperature of about 37°C, exceeding 40°C will cause denaturation of enzymes ◆ High temp increases vibrations, breaks bonds between the R-groups of amino acids, changes enzyme shape ➔ Reversible denaturation (renaturation) ◆ Some proteins can return to their functional shape after denaturation, many cannot ◆ E.g. curling or straightening of hair C1.1.8: Effects of temperature, pH, and substrate concentration on the rate of enzyme activity Factors affecting enzyme function 1. Enzyme concentration a. ↑ enzyme = ↑ reaction rate, more enzymes = more frequently collide with substrate 2. Substrate concentration a. ↑ substrate = ↑ reaction rate, more substrate = more frequently collide with enzyme 3. Temperature a. Able to speed up and slow down reactions b. When temperature is cold, molecules move slowly, less opportunities for an enzyme and substrate to bump into one another (slows the rate of reaction and the effect of enzyme c. When temperature increases, molecules move quickly and bump into one another more frequently (↑ temperature = ↑ rate of reaction) d. Optimum temperature (greatest number of molecular collisions) 4. pH a. pH scale: a measure of H+ concentration and therefore acidity b. ↓ pH = ↑ H+ concentration ↑ acidity, c. ↓ pH or ↑ pH = low