Evolutionary Biology PDF
Document Details
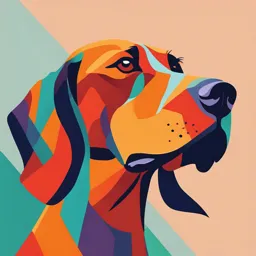
Uploaded by AppropriateBromeliad5222
Tags
Summary
This document discusses concepts in evolutionary biology, such as microevolution, macroevolution, Darwinian fitness, and artificial selection. It explores the evidence for relationships between species, divergent and convergent evolution, and different mechanisms for speciation.
Full Transcript
1. Compare the two scales of evolution Evolution can be examined on two primary scales: microevolution and macroevolution. Microevolution refers to small-scale changes that occur within a population over time. This often involves changes in allele frequency due to mechanisms like natural selection,...
1. Compare the two scales of evolution Evolution can be examined on two primary scales: microevolution and macroevolution. Microevolution refers to small-scale changes that occur within a population over time. This often involves changes in allele frequency due to mechanisms like natural selection, genetic drift, mutation, and gene flow. For instance, in a population of mice, microevolution might be evident in changes in fur color in response to the environment, such as darker colors becoming more prevalent in areas with dark soil. Macroevolution, on the other hand, encompasses larger-scale evolutionary changes that occur over long periods, often leading to the emergence of new species (speciation), the evolution of major evolutionary novelties, and large-scale patterns of change in biodiversity. This can include phenomena such as the evolution of mammals from reptilian ancestors or the origin of birds from theropod dinosaurs. Macroevolution is often observed in the fossil record and can be inferred through comparative anatomy, biogeography, and molecular biology. Both scales intertwine: microevolutionary changes can accumulate over time, potentially leading to macroevolutionary outcomes. Understanding the distinction and connection between these scales is key to grasping the comprehensive picture of how life evolves. 2. Describe Darwinian fitness Darwinian fitness, often simply referred to as "fitness," quantifies an organism's ability to survive and reproduce in a given environment. It is not solely about strength or health but rather pertains to reproductive success. Here are key points to understand: Survival: An organism must navigate environmental challenges, such as predation, competition for resources, and disease. Those that are better suited to their environment are more likely to survive long enough to reproduce. Reproductive Success: Fitness incorporates both the number of offspring produced and the likelihood of those offspring surviving to reproduce themselves. For example, if a particular phenotype has the advantage of more appealing coloration in a mate-selection context, these individuals may have higher reproductive success. Relative Measure: Fitness is usually a comparative concept. It is measured relative to the reproductive output of other individuals in the population. The more successful an organism is in producing fertile offspring, the higher its fitness regarding the population's gene pool dynamics. 3. Outline artificial selection Artificial selection is the intentional breeding of organisms with desired traits by humans. This process serves as a mechanism of evolution where human preferences drive changes rather than environmental pressures. Key points include: Human Intervention: Unlike natural selection where environmental pressures dictate survival, artificial selection is controlled by humans who select which individuals contribute to the next generation based on specific traits, such as size, color, or behavior. Examples: Classic examples include domestic dogs, where various breeds were developed from wolves based on traits like size, temperament, and appearance. Crop plants have also undergone extensive artificial selection, leading to varieties with enhanced yield, disease resistance, or flavor. Implications: While artificial selection can lead to rapid changes in populations, it can also produce unintended consequences such as reduced genetic diversity or health issues in extreme phenotypes, which affects the population’s long-term viability. 4. Explain how natural selection can change one population into several distinct species Natural selection can drive speciation through several mechanisms: Geographic Isolation: When populations of a species become separated geographically (e.g., by mountains or waters), they can experience different environmental pressures. Over time, if these separate groups adapt to their specific environments, they may evolve distinct traits, leading to reproductive barriers and, ultimately, speciation. Genetic Drift: In small populations, random changes in allele frequencies can drastically affect the populations' genetic structure over generations, particularly when combined with natural selection. This can promote divergence from the original population. Adaptive Radiation: This occurs when a single ancestral species rapidly diversifies into multiple distinct species that occupy various ecological niches. A classic example is Darwin’s finches in the Galápagos Islands, where a common ancestor evolved into multiple species with adaptations suited for different feeding strategies. Reproductive Isolation: Through mechanisms like behavioral isolation, temporal isolation, and mechanical isolation, populations can become reproductively isolated as they adapt to different environments, making them unable to interbreed even if they come back into contact. 5. Describe the evidence for relationships between species Evidence for evolutionary relationships among species stems from various disciplines: Morphological Evidence: Comparative anatomy reveals homologous structures—similar structures in different species due to shared ancestry. For example, the forelimb of humans and the wings of bats have a similar bone structure but serve different functions. Molecular Evidence: DNA and RNA sequencing have revealed genetic similarities among species. By analyzing the genetic material of different organisms, scientists can infer evolutionary relationships and the time of divergence from common ancestors. Fossil Records: The fossil record provides chronological evidence of organisms that lived in the past and shows transitional fossils that reveal shifts in characteristics and the gradual changes among species. Biogeography: The geographical distribution of species can explain evolutionary relationships. For instance, unique species on isolated islands (like the Galápagos tortoises) can be explained by their evolutionary history linked to continental drift and historical migrations. 6. Compare divergent and convergent evolution Divergent Evolution occurs when two or more related species become more dissimilar over time, often due to different environmental pressures or niches. The classic example is the evolution of marsupials in Australia versus placental mammals in other parts of the world. Although they share a common ancestor, they have diverged significantly in characteristics. Convergent Evolution refers to the process where unrelated species develop similar traits or adaptations in response to similar environmental challenges. An example is the evolution of wings in bats (mammals) and birds (aves). Despite coming from different evolutionary trajectories, these wings serve similar functions, demonstrating convergence in form and function. In evaluating these concepts, you can see that both divergent and convergent evolution illustrate how organisms adapt to their environments but do so through different evolutionary pathways. a. Compare a homologous structure to an analogous structure Homologous Structures: These structures arise from a common ancestor and have similar morphological features but may serve different functions. For instance, the forelimbs of humans, whales, and bats all share the same underlying skeletal arrangement (humerus, radius, ulna) but are adapted to various functions (manipulation, swimming, flying). Analogous Structures: These structures serve similar functions but do not share a common evolutionary origin. Instead, they arise independently in different species due to similar environmental pressures (convergent evolution). An example is the wings of insects and birds. While they both serve the function of flight, their structural differences indicate a lack of a recent common ancestor. In summary, understanding the relationships and distinctions between homologous and analogous structures highlights the various mechanisms of evolution and how they shape the diversity of life. Homologous structures exemplify shared ancestry, while analogous structures illustrate adaptation to environmental pressures.