Efficient Z-Selective Semihydrogenation of Internal Alkynes PDF
Document Details
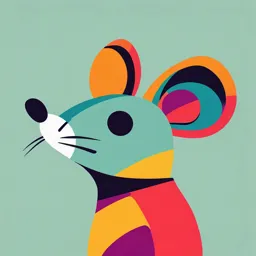
Uploaded by TolerablePlanet4004
Université Paul Sabatier (Toulouse III)
2019
Nikolaus Gorgas, Julian Brünig, Berthold Stöger, Stefan Vanicek, Mats Tilset, Luis F. Veiros, Karl Kirchner
Tags
Related
Summary
This paper details the synthesis and application of new cationic iron(II) hydride complexes for the efficient and highly Z-selective semihydrogenation of internal alkynes, 1,3-diynes, and 1,3-enynes. The authors demonstrate high selectivity and a broad scope of substrates and discuss the mechanistic details.
Full Transcript
Article Cite This: J. Am. Chem. Soc. 2019, 141, 17452−17458 pubs.acs.org/JACS...
Article Cite This: J. Am. Chem. Soc. 2019, 141, 17452−17458 pubs.acs.org/JACS Efficient Z‑Selective Semihydrogenation of Internal Alkynes Catalyzed by Cationic Iron(II) Hydride Complexes Nikolaus Gorgas,† Julian Brünig,† Berthold Stöger,‡ Stefan Vanicek,§ Mats Tilset,§ Luis F. Veiros,# and Karl Kirchner*,† † Institute of Applied Synthetic Chemistry and ‡X-Ray Center, Vienna University of Technology, Getreidemarkt 9, A-1060 Vienna, Austria § Department of Chemistry, University of Oslo, P.O. Box 1033, Blindern, N-0315 Oslo, Norway # Centro de Química Estrutural, Instituto Superior Técnico, Universidade de Lisboa, Av. Rovisco Pais No. 1, 1049-001 Lisboa, Downloaded via UNIV TOULOUSE III - PAUL SABATIER on September 11, 2024 at 14:54:40 (UTC). See https://pubs.acs.org/sharingguidelines for options on how to legitimately share published articles. Portugal * S Supporting Information ABSTRACT: The bench-stable cationic bis(σ-B−H) amino- borane complex [Fe(PNPNMe-iPr)(H)(η2-H2B = NMe2)]+ (2) efficiently catalyzes the semihydrogenation of internal alkynes, 1,3-diynes and 1,3-enynes. Moreover, selective incorporation of deuterium was achieved in the case of 1,3-diynes and 1,3- enynes. The catalytic reaction takes place under mild conditions (25 °C, 4−5 bar H2 or D2) in 1 h, and alkenes were obtained with high Z-selectivity for a broad scope of substrates. Mechanistic insight into the catalytic reaction, explaining also the stereo- and chemoselectivity, is provided by means of DFT calculations. Intermediates featuring a bisdihydrogen moiety [Fe(PNPNMe-iPr)(η2-H2)2]+ are found to play a key role. Experimental support for such species was unequivocally provided by the fact that [Fe(PNPNMe-iPr)(H)(η2-H2)2]+ (3) exhibited the same catalytic activity as 2. The novel cationic bisdihydrogen complex 3 was obtained by protonolysis of [Fe(PNPNMe-iPr)(H)(η2-AlH4)]2 (1) with an excess of nonafluoro-tert-butyl alcohol. INTRODUCTION The semihydrogenation of alkynes to alkenes is an important Within this context, homogeneous catalysts based on first row transition metals attracted particular interest. Apart from transformation for the industrial manufacture of bulk and fine obvious advantages such as their low price and high chemicals.1 Since the reduction of CC triple bonds to abundance, it is their intrinsic properties that may provide alkenes may potentially lead to the formation of (E)- or (Z)- new opportunities to pave the way to unprecedented alkenes as well as saturated hydrocarbons, it remains a reactivities and selectivities in catalytic transformations.10 challenging task to control the chemo- and stereoselectivity Iron may be considered as a particularly promising candidate of this reaction.2 For example, (Z)-olefins are traditionally in this respect.11 For example, much progress could be obtained by heterogeneous hydrogenation, with the Lindlar achieved in the field of iron-catalyzed hydrogenations of catalyst being the most prominent example.3 However, these olefins.12 Concerning the selective reduction of alkynes, some systems require a careful control of the reaction conditions in iron complexes could successfully be applied that operate via order to avoid isomerization or overreduction of the products. hydrofunctionalization or transfer hydrogenation procedures.13 Although significant progress could be achieved in the field However, only two examples are currently known that can of heterogeneous catalysis and homogeneous transfer hydro- promote this transformation using hydrogen gas as the genations, the number of molecular defined catalysts that reductant (Scheme 1).8a14 operate under an atmosphere of H2 is surprisingly low.4−6 In 1989, Bianchini et al. discovered that the nonclassical Concerning the stereoselectivity of the reaction, recent polyhydride [Fe(PP3)(H)(η2-H2)]+ is capable of catalyzing the examples were shown to deliver (E)-alkenes which, except in semihydrogenation of terminal alkynes.14 More recently, the some cases,7 are formed due to secondary isomerization group of Milstein reported on a novel acridine-based pincer- processes.8 Consequently, the development of new and type complex that bears an imino borohydride coligand.8a This selective homogeneous semihydrogenation catalysts that are able to produce (Z)-alkenes without isomerization or over- Received: September 13, 2019 reduction would still be of great advantage.9 Published: October 7, 2019 © 2019 American Chemical Society 17452 DOI: 10.1021/jacs.9b09907 J. Am. Chem. Soc. 2019, 141, 17452−17458 Journal of the American Chemical Society Article Scheme 1. Iron Catalysts for the Semihydrogenation of Table 1. Semihydrogenation of Alkynes Catalyzed by 2 Alkynes complex was found to reduce internal alkynes selectively to the respective (E)-olefins. The reaction requires several hours and rather high reaction temperatures (90 °C) to achieve high yields and selectivities. Additional studies revealed that the hydrogenation initially affords (Z)-alkenes which, however, are isomerized to the respective (E)-olefins by the same catalyst. We describe here the application of the well-defined bench- stable cationic aminoborane complex [Fe(PNPNMe-iPr)(H)- (η2-H2B = NMe2)]+ (2) described recently15 as a highly efficient precatalyst for the semihydrogenation of internal alkynes, 1,3-diynes, and 1,3-enynes with molecular hydrogen a under mild conditions. We take advantage of the fact that the Conditions: (a) alkyne (1.0 mmol), 2 (1.0 mol %), CH2Cl2 (1 mL), aminoborane ligand, which is coordinated to the metal center 25 °C, 1 h, conversion and selectivity determined by 1H NMR. via two weak σ-B−H bonds in η2-fashion, is substitutionally Isolated yields given in parentheses. labile and upon dissociation readily provides two vacant b Conditions: 3 (2.0 mol %), 2 h reaction time. cConditions: 7 (1.5 coordination sites to bind dihydrogen and alkynes. mol %), MeOH/CH2Cl2 (1:9, 1 mL), 1 h. RESULTS AND DISCUSSION The aminoborane complex [Fe(PNPNMe-iPr)(H)(η2-H2B = NMe2)]+ (2) was tested as a precatalyst for the hydrogenation A20). In any case, the respective (Z,Z)-butadienes could be of a variety of different alkyne substrates in order to examine obtained with excellent stereoselectivity (Table 2). Functional the general applicability and functional group tolerance of this groups such as esters or amine groups are tolerated, whereas novel system. Apart from 1-phenylpropyne, also dialkyl and the CC double bond of the enyne substrates remained diphenyl substrates could be reduced to the respective (Z)- unaffected. alkenes (Table 1, A1−A3) without isomerization of the This circumstance was further examined by conducting the products. Neither an increase of the catalyst loading nor higher hydrogenation in the presence of D2 (Scheme 2). The reaction pressures led to a further reduction of the alkene. Terminal of 1,3-enyne A16b with D2 catalyzed by 2 resulted in complete alkynes, however, are further hydrogenated to yield the and selective deuterium incorporation at the former alkyne saturated alkanes. Overreduction could be prevented by the carbons, whereas an isotope scrambling into the existing olefin introduction of trimethylsilyl (TMS) moieties as protecting C−H positions was not observed. On the other hand, the groups which were tolerated throughout without cleavage of respective 1,3-diyne A16a gave the fully deuterated butadiene the C−Si bond. Even those substrates were quantitatively product under the same reaction conditions. Thus, the novel reduced, affording the respective alkenes with excellent (Z)- iron hydride complex represents an interesting catalytic system selectivity (except for the TMS-protected phenylacetylene A4 that allows for the selective hydrogen isotope labeling of for which minor amounts of the trans-isomer were found in the butadiene derivatives. isolated product). The reaction proceeds well also in the case Based on the experimental observations, it is likely that the of diynes (A13, A14) which could successfully be converted to reaction proceeds via a classical insertion mechanism. Due to the corresponding (Z,Z)-dienes. the labile nature of the dihydrogen and bis(σ-B−H) amino- Encouraged by these results, we extended the substrate borane ligands, the precatalyst is activated by the addition of scope to 1,3-diynes (A16a) as well as 1,3-enynes (A16b− the substrate in the presence of dihydrogen. In contrast, 17453 DOI: 10.1021/jacs.9b09907 J. Am. Chem. Soc. 2019, 141, 17452−17458 Journal of the American Chemical Society Article Table 2. Hydrogenation of 1,3-Diynes and 1,3-Enynes A series of DFT calculations were carried out in order to Catalyzed by Complex 2 gain more detailed insight into the catalytic cycle as well as the origin of the observed selectivity.16 A simplified catalytic cycle for the semihydrogenation of internal alkynes is depicted in Scheme 3. The cycle starts with an alkyne dihydrogen complex Scheme 3. Simplified Catalytic Cycle for the Semihydrogenation of Internal Alkynes Catalyzed by 2 faut détailler le mécanisme et expliquer la structure of Iron Catalysis a Conditions: (a) alkyne (1.0 mmol), 2 (1.0 mol %), CH2Cl2 (1 mL), r.t., 1 h, conversion and selectivity determined by 1H NMR. Isolated yields given in parentheses. b Conditions: (b) 10 bar H2. Scheme 2. Selective Deuterium Incorporation through Hydrogenation of 1,3-Diynes and 1,3-Enynes by 2 with D2 of the type [Fe(PNPNMe-iPr)(H)(η2-H2)(η2-MeCCPh)]+ as the active species. The η2-coordinated alkyne inserts into the iron hydride bond, resulting in a dihydrogen vinyl species (I). After coordination of a second equivalent of H2 (II), this intermediate split the H−H bond under release of the alkene product and recovery of the initial hydride dihydrogen complex (III). dissociation of the aminoborane ligand in 2 under an A more detailed picture is provided by the free energy profile atmosphere of dihydrogen to form 3 was not observed.15 in Scheme 4, employing 1-phenylpropyne as the substrate. The hydrogenation of A1 was conducted at different Insertion of the alkyne into the iron hydride bond proceeds hydrogen pressures as well as substrate concentrations, and easily with an activation barrier of 1 kcal/mol in an exergonic the initial turnover frequencies were determined after a step (ΔG = −11 kcal/mol). This occurs with a H-shift between reaction time of 5 min. A significant increase of the initial two adjacent coordination positions and results in intermediate TOFs could be observed at higher hydrogen pressures (see 1-B with trans-dihydrogen and vinyl ligands. Coordination of a Supporting Information, Table S1). At 20 bar H2, after 5 min H2 molecule to the resulting iron vinyl complex affords the product was obtained in >99% yield, corresponding to a intermediate 1-D in which there are two dihydrogen ligands TOF of 2400 h−1. In contrast, essentially no acceleration took parallel to the P−Fe−P axis. 1-D formation overcomes a place when the substrate concentration was raised. These barrier of only 3 kcal/mol, and that step is exergonic with ΔG results indicate that the rate-determining step of the = −5 kcal/mol. From 1-D, a second H transfer with mechanism should be loss of the aminoborane ligand and concomitant reorientation of the H2 ligand produces 1-E, formation of the active species being in line with the very small where the recently formed olefin is loosely coordinated as a energy barrier (11 kcal/mol) calculated for the catalytic C−H σ-complex. Requiring 11 kcal/mol, this step represents reaction (vide infra). the highest barrier in the catalytic cycle. The overall free energy 17454 DOI: 10.1021/jacs.9b09907 J. Am. Chem. Soc. 2019, 141, 17452−17458 Journal of the American Chemical Society Article Scheme 4. Free Energy Profile for the Hydrogenation of 1-Phenylpropynea a Free energies (kcal/mol) refer to intermediate 1-A. Scheme 5. Free Energy Profile for the Hydrogenation of Phenylacetylenea a Free energies (kcal/mol) refer to intermediate 2-A. balance of the cycle is favorable with ΔG = −22 kcal/mol, and alkane in our calculations (right side of the profile in Scheme closing the cycle with liberation of the olefin and addition of a 4). Here, the loosely bonded olefin in intermediate 1-E new alkyne molecule regenerates the initial species (1-A) in a rearranges to η2-coordinated (in 1-G). This process occurs in slightly exergonic process (ΔG = −3 kcal/mol). two steps. First there is H-exchange, bringing the H2 ligand Regarding the chemoselectivity of the reaction, we also back to its original position, i.e., opposite to the Npy atom, in considered a second hydrogenation step to the respective intermediate 1-F. Then, a reorientation of the olefin brings it 17455 DOI: 10.1021/jacs.9b09907 J. Am. Chem. Soc. 2019, 141, 17452−17458 Journal of the American Chemical Society Article to the η2-coordination mode present in intermediate 1-G. In order to obtain support for the mechanistic studies from These steps have negligible barriers (≤1 kcal/mol), and 1-G is an experimental point of view, in particular the existence of 7 kcal/mol more stable than 1-E, reflecting the additional bisdihydrogen intermediates, we prepared the novel cationic stability associated with stronger coordination of the olefin. bisdihydrogen complex [Fe(PNPNMe-iPr)(H)(η2-H2)2]+ (3). From 1-G, there is H-transfer to the inner C atom of the CC This complex was readily obtained by reacting [Fe(PNPNMe- double bond with formation of an alkyl complex (1-H). The iPr)(H)(η2-AlH4)]2 (1)15 with an excess of nonafluoro-tert- process occurs with concomitant H-transfer between the two butyl alcohol (Scheme 6) in THF at room temperature. The adjacent coordination sites, and thus, both the H2 as well as the alkyl ligands occupy two apical positions trans to each other, in Scheme 6. Preparation of a Cationic Nonclassical Fe(II) 1-H. This step corresponds to a formal olefin insertion in a Polyhydride Complex via Protonolysis of 1a Fe−H bond and parallels the first step of the entire path (alkyne insertion, over 1-TSAB). This is an endergonic process with ΔG = 6 kcal/mol, and the associated barrier (13 kcal/ mol) is 2 kcal/mol higher than the one found for alkyne insertion, indicating that the second hydrogenation is less favorable than the first one, in agreement with the experimental results. However, the small difference between the calculated barriers may suggest the possibility of competitive processes. After H2 coordination (from 1-H to 1-I), a final H-transfer from the dihydrogen ligand to the terminal alkyl C atom produces the final intermediate, 1-J, with an alkane bonded as a C−H σ-complex. This is a facile process with a barrier of 6 kcal/mol and, also, clearly favorable with ΔG = −27 kcal/mol. The hydrogenation of phenylacetylene was also considered by means of DFT calculations, and the energy profile obtained for the corresponding reaction is depicted in Scheme 5. The mechanism is entirely equivalent to the one discussed above a Selected bond distances (Å) and angles (deg): Fe1−N1 1.9867(9), for the hydrogenation of 1-phenylpropyne. Thus, it also Fe1−P2 2.1443(3), Fe1−P1 2.1461(3), Fe1−Al1 2.3507(4), Fe1−H1 comprises two stepwise H atom additions to C−C unsaturated 1.46(2), Fe1−HAL1 1.48(2), Fe1−HAL2 1.55(2), Al1−HAL1 bonds. The first can be viewed as an acetylene insertion into a 1.82(2), Al1−HAL2 1.73(2), Al1−HAL3 1.65(2), Al1−HAL4 hydride bond and results in a vinyl complex (2-B). Then, there 1.54(2), and P2−Fe1−P1 157.22(1). is H2 coordination followed by another H-transfer producing the olefin (styrene, in this case) and regenerating a hydride ligand (intermediate 2-E). A repetition of this sequence leads coordinated [AlH4]− anion is protonated by the acidic alcohol, thereby liberating H2 with concomitant formation of a mixture to the hydrogenation of styrene and the formation of the of several poorly coordinating counterions of the types alkane (ethylbenzene) in the final intermediate, 2-J. [Al(OC(CF3)3)4−nHn]− as detected by 19F{1H} NMR spec- The most important difference between the two paths is the troscopy and ESI-MS.16 relative value of the highest barrier for each H2 addition. In the The 1H NMR spectrum of 3 in THF-d8 features a broadened case of the internal alkyne (1-phenylpropyne, Scheme 4), the triplet resonance at −14.68 ppm that integrates to five barrier for the second addition is the higher one, justifying the hydrogen atoms, while a singlet at 187.3 ppm could be observed semihydrogenation of the substrate. However, in the observed in the 31P{1H} NMR spectrum. Owing to fast case of the terminal alkyne (phenylacetylene, Scheme 5), the exchange between classical and nonclassical hydrides, it was opposite occurs. That is, the barrier for olefin hydrogenation not possible to determine separate proton resonances for the (12 kcal/mol, 2-TSFG) is lower than the one calculated for the individual hydride ligands. Even at −100 °C only a slight hydrogenation of the alkyne (15 kcal/mol, 2-TSAB). Both broadening of the hydride signal was observed. 1H NMR processes correspond to the first H-transfer, from the H2 ligand spectra recorded at variable temperatures revealed an to the corresponding C−C unsaturation. The results above extremely short relaxation time T1(min) of 12 ms (−65 °C, indicate that in the case of phenylacetylene the reaction is 500 MHz)17 which is characteristic of coordinated dihydrogen expected to go all the way until the saturated product. molecules.18,19 The observed chemoselectivity can also be related to the The experimental observations are further supported by stability of the η2-olefin complexes in each case (1-G and 2-F). DFT calculations. The cis- and trans-isomers of the bis- In fact, those are the initial species in the second H2 addition, (dihydrogen) complex differ merely by 2.0 kcal/mol, and their from olefin to alkane. Considering the competition between H2 interconversion requires an activation energy of just 5.0 kcal/ and the olefin for the sixth coordination positions (Figure S1, mol, being in line with the experimentally observed fluxional Supporting Information), it becomes clear that the olefin behavior (Scheme 7). complex is more stable in the case of the terminal olefin Complex 3 was also applied as a precatalyst for the (styrene, in 2-F), while the opposite happens in the case of the hydrogenation of alkynes. Experiments were conducted in internal olefin (1-phenylpropene, in 1-G). Therefore, the C6D6 under a H2 pressure of 5 bar at 25 °C using 1- existence of the initial species for the second hydrogenation phenylpropyne as the test substrate. By employing 1 mol % of process is favorable in the case of substrates with a terminal in situ prepared 3, the alkyne could be quantitatively reduced CC double bond, contrarily to what occurs for internal ones. to the corresponding alkene within 30 min. The product was 17456 DOI: 10.1021/jacs.9b09907 J. Am. Chem. Soc. 2019, 141, 17452−17458 Journal of the American Chemical Society Article Scheme 7. Free Energy Profile (kcal/mol) Calculated for the Interconversion of cis- and trans-Isomers of 3a * ASSOCIATED CONTENT S Supporting Information The Supporting Information is available free of charge on the ACS Publications website at DOI: 10.1021/jacs.9b09907. Synthetic procedures, NMR spectra of all compounds, and crystallographic data (PDF) Optimized Cartesian coordinates for DFT-optimized structures (XYZ) X-ray crystallographic data for 1 (CCDC entry 1951434) (CIF) AUTHOR INFORMATION Corresponding Author *E-mail (K. Kirchner): [email protected]. Tel.: (+43) 1 58801 163611. Fax: (+43) 1 58801 16399. ORCID a 1 Nikolaus Gorgas: 0000-0003-2919-1042 Inset: H NMR spectrum of 3 (hydride region, 250 MHz, THF-d8, 20 °C). Mats Tilset: 0000-0001-8766-6910 Luis F. Veiros: 0000-0001-5841-3519 Karl Kirchner: 0000-0003-0872-6159 Notes formed with >99% Z-selectivity, and no hydrogenation to the The authors declare no competing financial interest. respective alkane could be observed even when the reaction time was extended to several hours. Accordingly, complexes 2 and 3 are obviously synthons for the active catalyst which ACKNOWLEDGMENTS N.G. and K.K. gratefully acknowledge the financial support by reacts with alkynes in the presence of H2 to form [Fe(PNPNMe- the Austrian Science Fund (FWF) (Project No. P29584-N28). iPr)(H)(η2-H2)(η2-RCCR′)]+, thereby initiating the cata- N.G. thanks the COST-CHAOS action for granting a STSM to lytic cycle as shown in Schemes 3−5. It has to be noted that the University of Oslo. S.V. thanks the Austrian Science Fund the neutral nonclassical iron(II) polyhydride complex [Fe- (FWF) for an Erwin Schrödinger Postdoctoral Fellowship (PNP)(H)2(η2-H2)] did not catalyze the hydrogenation of (Project No. J4158). L.F.V. thanks Fundaçaõ para a Ciência e alkynes but the dimerization of alkynes to give 1,3-eneynes Tecnologia, UID/QUI/00100/2013. The Research Council of with high Z-selectivity.20 Norway supported this work through the Norwegian NMR Platform, NNP (226244/F50). Senior Engineer Dirk Petersen CONCLUSION (University of Oslo NMR center) is thanked for variable- The bench-stable cationic bis(σ-B−H) aminoborane complex temperature NMR assistance. This paper is dedicated to [Fe(PNPNMe-iPr)(H)(η2-H2BNMe2)]+ (2) turned out to be Professor Marko D. Mihovilovic on the occasion of his 50th an efficient precatalyst for the semihydrogenation of internal birthday. alkynes, 1,3-diynes, and 1,3-enynes. With 1,3-diynes and 1,3- enynes, deuterium could be selectively incorporated in the presence of D2. This was exemplarily shown with 1,4- REFERENCES (1) Kluwer, A. M.; Elsevier, C. J. Homogeneous Hydrogenation of diphenylbuta-1,3-diyne and (Z)-but-1-en-3-yne-1,4-diyldiben- Alkynes and Dienes. In The Handbook of Homogeneous Hydrogenation; zene as the isotopomeres, and (Z,Z-buta-1,3-diene-1,4-diyl-1,2- Wiley-VCH Verlag GmbH: Weinheim, Germany, 2007; pp 374−411. d2)dibenzene and (Z,Z-buta-1,3-diene-1,4-diyl-d4)dibenzene, (2) Swamy, K. C. K.; Reddy, A. S.; Sandeep, K.; Kalyani, A. respectively, were obtained. The catalytic reaction takes place Advances in Chemoselective and/or Stereoselective Semihydrogena- under mild conditions (1 h, 25 °C, 4−5 bar H2 or D2), and all tion of Alkynes. Tetrahedron Lett. 2018, 59, 419−429. (3) Lindlar, H. Ein neuer Katalysator für Selektive Hydrierungen. alkenes were obtained with high Z-selectivity for a broad scope Helv. Chim. Acta 1952, 35, 446−450. of substrates. Mechanistic insight into the catalytic reaction is (4) Delgado, J. A.; Benkirane, O.; Claver, C.; Curulla-Ferré, D.; provided by means of DFT calculations. The stereo- and Godard, C. Advances in the Preparation of Highly Selective chemoselectivity were fully explained in agreement with the Nanocatalysts for the Semi-Hydrogenation of Alkynes Using Colloidal experimental observations. Intermediates featuring a bisdihy- Approaches. Dalton Trans. 2017, 46, 12381−12403. drogen moiety [Fe(PNPNMe-iPr)(η2-H2)2]+ are found to play a (5) For examples of transfer semihydrogenation catalysts, see: key role. Experimental support for such species was provided (a) Hauwert, P.; Maestri, G.; Sprengers, J. W.; Catellani, M.; Elsevier, by the fact that [Fe(PNPNMe-iPr)(H)(η2-H2)2]+ (3) exhibited C. J. Transfer Semihydrogenation of Alkynes Catalyzed by a Zero- the same catalytic activity as 2. The novel cationic Valent Palladium N-Heterocyclic Carbene Complex. Angew. Chem., bisdihydrogen complex 3 was obtained by protonolysis of Int. Ed. 2008, 47, 3223−3226. (b) Hauwert, P.; Boerleider, R.; Warsink, S.; Weigand, J. J.; Elsevier, C. J. Mechanism of Pd(NHC)- [Fe(PNPNMe-iPr)(H)(η2-AlH4)]2 (1) with an excess of Catalyzed Transfer Hydrogenation of Alkynes. J. Am. Chem. Soc. nonafluoro-tert-butyl alcohol. Thus, complexes 2 and 3 are 2010, 132, 16900−16910. (c) Shen, R.; Chen, T.; Zhao, Y.; Qiu, R.; apparently synthons for the active catalyst [Fe(PNPNMe- Zhou, Y.; Yin, S.; Wang, X.; Goto, M.; Han, L.-B. Facile Regio- and iPr)(H)]+, which reacts with alkynes in the presence of H2 Stereoselective Hydrometalation of Alkynes with a Combination of to form [Fe(PNPNMe-iPr)(H)(η2-H2)(η2-RCCR′)]+, there- Carboxylic Acids and Group 10 Transition Metal Complexes: by initiating the catalytic cycle. Selective Hydrogenation of Alkynes with Formic Acid. J. Am. Chem. 17457 DOI: 10.1021/jacs.9b09907 J. Am. Chem. Soc. 2019, 141, 17452−17458 Journal of the American Chemical Society Article Soc. 2011, 133, 17037−17044. (d) Cao, H.; Chen, T.; Zhou, Y.; Han, 33, 5781−5790. (g) Sunada, Y.; Ogushi, H.; Yamamoto, T.; Uto, S.; D.; Yin, S.-F.; Han, L.-B. Copper-Catalyzed Selective Semihydroge- Sawano, M.; Tahara, A.; Tanaka, H.; Shiota, Y.; Yoshizawa, K.; nation of Terminal Alkynes with Hypophosphorous Acid. Adv. Synth. Nagashima, H. Disilaruthena- and Ferracyclic Complexes Containing Catal. 2014, 356, 765−769. Isocyanide Ligands as Effective Catalysts for Hydrogenation of (6) (a) Schrock, R. R.; Osborn, J. A. Catalytic Hydrogenation Using Unfunctionalized Sterically Hindered Alkenes. J. Am. Chem. Soc. 2018, Cationic Rhodium Complexes. II. The Selective Hydrogenation of 140, 4119−4134. Alkynes to Cis Olefins. J. Am. Chem. Soc. 1976, 98, 2143−2147. (13) (a) Wienhöfer, G.; Westerhaus, F. A.; Jagadeesh, R. V.; Junge, (b) van Laren, M. W.; Elsevier, C. J. Selective Homogeneous K.; Junge, H.; Beller, M. Selective Iron-Catalyzed Transfer Hydro- Palladium(0)-Catalyzed Hydrogenation of Alkynes to (Z)-Alkenes. genation of Terminal Alkynes. Chem. Commun. 2012, 48, 4827. Angew. Chem., Int. Ed. 1999, 38, 3715−3717. (c) La Pierre, H. S.; (b) Johnson, C.; Albrecht, M. Z -Selective Alkyne Semi-Hydro- Arnold, J.; Toste, F. D. Z -Selective Semihydrogenation of Alkynes genation Catalysed by Piano-Stool N -Heterocyclic Carbene Iron Catalyzed by a Cationic Vanadium Bisimido Complex. Angew. Chem., Complexes. Catal. Sci. Technol. 2018, 8, 2779−2783. Int. Ed. 2011, 50, 3900−3903. (d) Tseng, K.-N. T.; Kampf, J. W.; (14) (a) Bianchini, C.; Meli, A.; Peruzzini, M.; Vizza, F.; Zanobini, Szymczak, N. K. Modular Attachment of Appended Boron Lewis F.; Frediani, P. A Homogeneous Iron(II) System Capable of Acids to a Ruthenium Pincer Catalyst: Metal-Ligand Cooperativity Selectivity Catalyzing the Reduction of Terminal Alkynes to Alkenes Enables Selective Alkyne Hydrogenation. J. Am. Chem. Soc. 2016, 138, and Buta-1, 3-Dienes. Organometallics 1989, 8, 2080−2082. 10378−10381. (b) Bianchini, C.; Meli, A.; Peruzzini, M.; Frediani, P.; Bohanna, (7) (a) Radkowski, K.; Sundararaju, B.; Fürstner, A. A Functional- C.; Esteruelas, M. A.; Oro, L. A. Selective Hydrogenation of 1-Alkynes Group-Tolerant Catalytic Trans Hydrogenation of Alkynes. Angew. to Alkenes Catalyzed by an Iron(II) Cis-Hydrideη2-Dihydrogen Chem., Int. Ed. 2013, 52, 355−360. (b) Leutzsch, M.; Wolf, L. M.; Complex. A Case of Intramolecular Reaction between η2-H2 and σ- Gupta, P.; Fuchs, M.; Thiel, W.; Farès, C.; Fürstner, A. Formation of Vinyl Ligands. Organometallics 1992, 11, 138−145. Ruthenium Carbenes by Gem -Hydrogen Transfer to Internal (15) Gorgas, N.; Stöger, B.; Veiros, L. F.; Kirchner, K. Access to Alkynes: Implications for Alkyne Trans-Hydrogenation. Angew. Fe(II) Bis(σ-B-H) Aminoborane Complexes via Protonation of a Chem., Int. Ed. 2015, 54, 12431−12436. (c) Schleyer, D.; Niessen, Borohydride Complex and Dehydrogenation of Amine-Boranes. H. G.; Bargon, J. In Situ 1H-PHIP-NMR Studies of the Stereoselective Angew. Chem., Int. Ed. 2019, 58, 13874−13879. Hydrogenation of Alkynes to (E)-Alkenes Catalyzed by a Homoge- (16) (a) Parr, R. G.; Yang, W. Density Functional Theory of Atoms neous [Cp*Ru]+ Catalyst. New J. Chem. 2001, 25, 423−426. and Molecules; Oxford University Press: New York, 1989. (b) (8) (a) Srimani, D.; Diskin-Posner, Y.; Ben-David, Y.; Milstein, D. Calculations performed at the M06/(6-311++G**)//PBE0/(SDD, Iron Pincer Complex Catalyzed, Environmentally Benign, E -Selective 6-31G**) level using the GAUSSIAN 09 package. Single-point energy Semi-Hydrogenation of Alkynes. Angew. Chem., Int. Ed. 2013, 52, calculations included solvent effects (benzene) using the PCM/SMD 14131−14134. (b) Karunananda, M. K.; Mankad, N. P. E -Selective model. A full account of the computational details and a complete list Semi-Hydrogenation of Alkynes by Heterobimetallic Catalysis. J. Am. of references are provided as SI. Chem. Soc. 2015, 137, 14598−14601. (c) Tokmic, K.; Fout, A. R. (17) (a) Barbarich, T. J.; Miller, S. M.; Anderson, O. P.; Strauss, S. Alkyne Semi-hydrogenation with a Well-Defined Nonclassical Co-H2 H. Coordination of the New Weakly Coordinating Anions Al(OCH- Catalyst: A H2 Spin on Isomerization and E -Selectivity. J. Am. Chem. (CF3)2)4−, Al(OC(CH3)(CF3)2)4−, and Al(OC(Ph)(CF3)2)4− to the Soc. 2016, 138, 13700−13705. (d) Higashida, K.; Mashima, K. E- Monovalent Metal Ions Li+ and Tl+. J. Mol. Catal. A: Chem. 1998, 128, Selective Semi-Hydrogenation of Alkynes with Dinuclear Iridium 289−331. (b) Krossing, I.; Raabe, I. Noncoordinating Anions -Fact or Complexes under Atmospheric Pressure of Hydrogen. Chem. Lett. Fiction? A Survey of Likely Candidates. Angew. Chem., Int. Ed. 2004, 2016, 45, 866−868. 43, 2066−2090. (c) Krossing, I. The Facile Preparation of Weakly (9) Oger, C.; Balas, L.; Durand, T.; Galano, J.-M. Are Alkyne Coordinating Anions: Structure and Characterization of Silver- Reductions Chemo-, Regio-, and Stereoselective Enough to Provide polyfluoroalkoxyaluminates AgAl(ORF)4, Calculation of the Alkoxide Pure (Z)-Olefins in Polyfunctionalized Bioactive Molecules? Chem. Ion Affinity. Chem. - Eur. J. 2001, 7, 490−502. (d) Riddlestone, I. M.; Rev. 2013, 113, 1313−1350. Kraft, A.; Schaefer, J.; Krossing, I. Taming the Cationic Beast: Novel (10) (a) Chirik, P.; Morris, R. Getting Down to Earth: The Developments in the Synthesis and Application of Weakly Renaissance of Catalysis with Abundant Metals. Acc. Chem. Res. 2015, Coordinating Anions. Angew. Chem., Int. Ed. 2018, 57, 13982−14024. 48, 2495−2495. (b) White, M. C. Base-Metal Catalysis: Embrace the (18) Hamilton, D. G.; Crabtree, R. H. An NMR Method for Wild Side. Adv. Synth. Catal. 2016, 358, 2364−2365. Distinguishing Classical from Nonclassical Structures in Transition (11) Fürstner, A. Iron Catalysis in Organic Synthesis: A Critical Metal Polyhydrides. J. Am. Chem. Soc. 1988, 110, 4126−4133. Assessment of What It Takes To Make This Base Metal a (19) Crabtree, R. H. Dihydrogen Complexation. Chem. Rev. 2016, Multitasking Champion. ACS Cent. Sci. 2016, 2, 778−789. 116, 8750−8769. (12) (a) Bart, S. C.; Lobkovsky, E.; Chirik, P. J. Preparation and (20) (a) Gorgas, N.; Alves, L. G.; Stöger, B.; Martins, A. M.; Veiros, Molecular and Electronic Structures of Iron(0) Dinitrogen and Silane L. F.; Kirchner, K. Stable, Yet Highly Reactive Nonclassical Iron(II) Complexes and Their Application to Catalytic Hydrogenation and Polyhydride Pincer Complexes: Z -Selective Dimerization and Hydroboration of Terminal Alkynes. J. Am. Chem. Soc. 2017, 139, Hydrosilation. J. Am. Chem. Soc. 2004, 126, 13794−13807. (b) Daida, 8130−8133. (b) Gorgas, N.; Stöger, B.; Veiros, L. F.; Kirchner, K. E. J.; Peters, J. C. Considering Fe II/IV Redox Processes as Iron(II) Bis(Acetylide) Complexes as Key Intermediates in the Mechanistically Relevant to the Catalytic Hydrogenation of Olefins Catalytic Hydrofunctionalization of Terminal Alkynes. ACS Catal. by [PhBP-iPr3]Fe-Hx Species. Inorg. Chem. 2004, 43, 7474−7485. 2018, 8, 7973−7982. (c) Trovitch, R. J.; Lobkovsky, E.; Bill, E.; Chirik, P. J. Functional Group Tolerance and Substrate Scope in Bis(Imino)Pyridine Iron Catalyzed Alkene Hydrogenation. Organometallics 2008, 27, 1470− 1478. (d) Yu, R. P.; Darmon, J. M.; Hoyt, J. M.; Margulieux, G. W.; Turner, Z. R.; Chirik, P. J. High-Activity Iron Catalysts for the Hydrogenation of Hindered, Unfunctionalized Alkenes. ACS Catal. 2012, 2, 1760−1764. (e) Fong, H.; Moret, M.-E.; Lee, Y.; Peters, J. C. Heterolytic H2 Cleavage and Catalytic Hydrogenation by an Iron Metallaboratrane. Organometallics 2013, 32, 3053−3062. (f) Hoyt, J. M.; Shevlin, M.; Margulieux, G. W.; Krska, S. W.; Tudge, M. T.; Chirik, P. J. Synthesis and Hydrogenation Activity of Iron Dialkyl Complexes with Chiral Bidentate Phosphines. Organometallics 2014, 17458 DOI: 10.1021/jacs.9b09907 J. Am. Chem. Soc. 2019, 141, 17452−17458