Glycolysis.pdf
Document Details
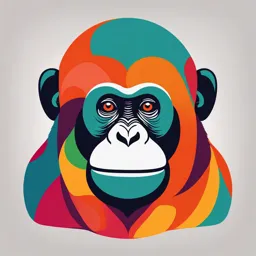
Uploaded by BraveWetland2534
Tags
Full Transcript
Glycolysis Navdeep S. Chandel Northwestern University, Feinberg School of Medicine, Chicago, Illinois 60611, USA Correspondence: [email protected] G lycolysis is an ancient pathway that evolved well before oxygen was present in the Earth’s atmosphere and is highly conserved...
Glycolysis Navdeep S. Chandel Northwestern University, Feinberg School of Medicine, Chicago, Illinois 60611, USA Correspondence: [email protected] G lycolysis is an ancient pathway that evolved well before oxygen was present in the Earth’s atmosphere and is highly conserved The overall reaction of glycolysis is exergon- ic (ΔG = −96 kJ/mol in erythrocytes): among living organisms. Glycolysis was the first glucose þ 2NADþ þ 2ADP þ 2Pi metabolic pathway elucidated and is also re- ! 2pyruvate þ 2NADH þ 2Hþ þ 2ATP ferred to as the Embden–Meyerhof–Parnas þ 2H2 O: pathway (see Box 1). The word “glycolysis” is derived from the Greek “glykys,” meaning “sweet,” and “lysis,” which means “to split.” THERE ARE THREE MAJOR FEATURES OF GLYCOLYSIS This refers to the splitting of one glucose mole- cule into two molecules of pyruvate, the end 1. It is the only pathway that can generate ATP product of glycolysis. In the presence of oxygen, in the absence of oxygen (anaerobic condi- pyruvate usually enters the mitochondria where tions) or in cells lacking mitochondria, such it is oxidized to acetyl-CoA, whereas in the ab- as red blood cells (erthyrocytes). In these sce- sence of oxygen, pyruvate is reduced into lactate. narios, pyruvate is converted into lactate. Glycolysis involves 10 reactions that take place in the cytosol and generates two ATP molecules 2. In the presence of oxygen, glycolysis gener- without the requirement of molecular oxygen. ates pyruvate, which enters the TCA cycle In contrast, oxidative phosphorylation in the (also called the citric acid cycle and the Krebs mitochondria generates 30 ATP molecules but cycle) located within mitochondria to pro- requires oxygen (see Chandel 2020a). Multiple duce ATP. simple sugars can enter glycolysis, including the 3. Many of the metabolites of glycolysis and monosaccharides glucose, galactose, and fruc- the TCA cycle can also enter anabolic tose. Most of us ingest these simple sugars pathways that generate NADPH and the through consumption of products that contain building blocks needed for generation of the disaccharides sucrose (table sugar) or lactose glycogen, lipid, nucleotide, and protein syn- (milk sugar). Sucrose is composed of one mole- thesis. The biosynthetic pathways that gly- cule of glucose and fructose, whereas lactose colytic intermediates funnel into include contains one molecule of glucose and galactose. the pentose phosphate, the hexosamine, The digestive enzymes sucrase and lactase break and the serine and glycerol biosynthetic down sucrose and lactose into simple sugars. pathways. From the recent volume Navigating Metabolism by Navdeep S. Chandel Additional Perspectives on Metabolism available at www.cshperspectives.org Copyright © 2021 Cold Spring Harbor Laboratory Press; all rights reserved; doi: 10.1101/cshperspect.a040535 Cite this article as Cold Spring Harb Perspect Biol 2021;13:a040535 1 N.S. Chandel BOX 1. OTTO FRITZ MEYERHOF AND ENERGY TRANSFORMATION IN THE CELL Archibald Vivian Hill described Otto Fritz Meyerhof (1884–1951) as “…always been betwixt and between: a physiological chemist or a chemical physiologist, perhaps we should call him a ‘chemiologist’.” These characteristics are precisely what enabled Meyerhof and his coworkers to dissect the glycolysis pathway. Meyerhof had won the 1922 Nobel Prize in Physiology or Medicine (awarded in 1923 because of a quirk of Alfred Nobel’s will) “for his discovery of the fixed relationship between the consumption of oxygen and the metabolism of lactic acid in the muscle” with Hill “for his discovery relating to the production of heat in the muscle.” Meyerhof initially thought he would pursue psychiatry and philosophy, but in 1909 he crossed paths with Otto Warburg, who persuaded him to study physiology. Early in his career, Meyerhof believed that the laws of physics and chemistry should apply to living organ- isms, and in 1913 he lectured on a theory of the thermodynamics of living matter—“The Energetics of Cell Processes.” In the late 1920s through the 1930s at the Institute of Physiology of the Kaiser Wilhelm Institute of Medical Research in Heidelberg, Meyerhof put together several key discoveries, including Gustav Embden’s isolation of AMP and his outline of the glycolysis pathway ( just before his death), Jakub Parnas’s work on phosphorolysis, and Karl Lohman’s discovery of ATP, and combined them with precise laboratory work to dissect and rebuild the glycolysis pathway and identify one-third of the enzymes involved. By identifying these intermediate reactions and showing the series of transformations that make energy avail- able to the cell, Meyerhof answered the questions posed in his 1913 lecture about how energy transformations and chemical changes affect the function of cells. He later confirmed that the glycolysis pathway in muscle and yeast was the same, thus showing it to be an essential pathway in living organisms. That Meyerhof was much respected as a mentor and investigator is reflected in the “who’s who” of researchers who passed through his laboratories in Germany— among them, David Nachmansohn, Severo Ochoa, Fritz Lipmann, George Wald, Andre Lwoff, Fritz Haber, and Otto Kahn. QUICK GUIDE TO THE ENERGY- ATP is generated by substrate-level phosphor- GENERATING CAPACITY OF ylation (see below). GLYCOLYSIS (FIG. 1) The net ATP generation is 2ATP: 4 ( payoff phase) – 2 (investment phase). Glycolysis takes place in the cytosol and does not require oxygen to generate ATP. Note that Two molecules of NAD+ are reduced to there is no net loss of carbon or oxygen atoms NADH, generated during glycolysis. in glycolysis. Nine of the 10 glycolytic intermediates are The 10 enzymatic reactions can be divided into phosphorylated metabolites, which cannot two phases: ATP investment (reactions 1–5) diffuse out of the cell because of their negative and ATP payoff (reactions 6–10). charge. Every one molecule of glucose entering gly- Glycolysis has three key regulatory steps (1, 3, colysis generates two molecules of glyceralde- and 10) catalyzed by hexokinase, phospho- hyde 3-phosphate using two molecules of fructokinase, and pyruvate kinase. These ATP during the ATP investment phase. have large negative ΔG values and are essen- tial to drive the overall flux to pyruvate. These The two molecules of glyceraldehyde 3-phos- regulatory steps are essentially irreversible. phate are metabolized into two molecules of pyruvate, generating four molecules of ATP Pyruvate can either be converted to lactate or during the ATP payoff phase. enter mitochondria to fuel the TCA cycle. 2 Cite this article as Cold Spring Harb Perspect Biol 2021;13:a040535 Glycolysis 6 CH 2 OH H 5 O H Glucose 4 OH H 1 ATP OH OH H E X OK IN ASE 3 2 ( G L UC O K IN ASE) 1 H OH ADP 6 P O CH 2 Glucose 6-phosphate H 5 O H 4 OH H 1 P H OS P H OG LUC OSE 2 I S O MER ASE OH OH 3 2 H OH AT P I N V E S TME N T 6 1 Fructose 6-phosphate P O CH 2 O CH 2 OH ATP 5 H OH 2 P HOSPHO- 3 H OH F R UC T OK IN ASE-1 4 3 ADP OH H 6 1 Fructose 1,6-bisphosphate P O CH 2 O CH 2 O P 5 H OH 2 ALD OLASE 4 H OH 4 3 OH H Glyceraldehyde 3-phosphate H O + P O CH 2 C C Dihydroxyacetone phosphate H OH P O CH 2 C CH 2 OH T R I OS E P H OSPHATE 5 I S O MERASE O H O Glyceraldehyde 3-phosphate (2) P O CH 2 C C H 2P i + 2NAD + OH GLYC E R A L D E HYD E 3 -P 6 D E H YD R O G EN ASE H 2NADH + 2H + O P O CH 2 C C 1,3-Bisphosphoglycerate (2) O P OH 2 ADP P H OS P H OGLYC ERATE 7 K IN ASE H ATP EA R N I N GS 2 ATP O P O CH 2 C C 3-Phosphoglycerate (2) O– OH P H OS P H OGLYC ERATE 8 MUTASE H O CH 2 OH C C 2-Phosphoglycerate (2) O– O EN OLASE 9 P 2H 2 O O CH 2 C C Phosphoenolpyruvate (2) O– O 2 ADP PYR UVATE K IN ASE 10 P 2 ATP O CH 3 C C Pyruvate (2) O– O TCA CYCLE H O Lactate (2) CH 3 C C O– OH Figure 1. The 10 steps of glycolysis. Cite this article as Cold Spring Harb Perspect Biol 2021;13:a040535 3 N.S. Chandel QUICK GUIDE TO BIOSYNTHETIC capacity, and ribose, the backbone of nucleo- CAPACITY OF GLYCOLYTIC tides and, hence, DNA or RNA. INTERMEDIATES (FIG. 2) Glucose 6-phosphate can generate glycogen. In addition to generating ATP, glycolytic interme- diates can funnel into multiple biosynthetic path- Fructose 6-phosphate can enter the hexos- ways. Mitochondria provide the bulk of ATP in amine biosynthetic pathway to generate ami- most cells when oxygen is abundant. Hence, the no sugars used for synthesis of glycoproteins, major function of glycolysis is to generate inter- glycolipids, and proteoglycans. mediates that fuel these biosynthetic pathways. In Dihydroxyacetone phosphate can be con- contrast, in the absence of oxygen, glycolysis’s verted into glycerol 3-phosphate to generate major role is to provide ATP for survival. lipids. Glucose 6-phosphate can enter the pentose 1,3-Bisphosphoglycerate generates 2,3-bis- phosphate pathway, resulting in the gene- phosphoglycerate, an allosteric regulator of ration of NADPH to maintain antioxidant hemoglobin, in red blood cells. Glucose GLYCOGEN ANTIOXIDANT Pentose Glucose 6-phosphate NADPH CAPACITY/ANABOLIC phosphate PATHWAYS pathway RIBOSE 5-PHOSPHATE NUCLEOTIDES GLYCOPROTEINS Hexosamine Fructose 6-phosphate AMINO SUGARS GLYCOLIPIDS pathway TRIGLYCERIDES Fructose 1,6-bisphosphate + FATTY ACIDS DIHYDROXYACETONE GLYCEROL 3-PHOSPHATE Glyceraldehyde 3-phosphate PHOSPHATE ALLOSTERIC 1,3-Bisphosphoglycerate 2,3-BISPHOSPHOGLYCERATE REGULATOR OF HEMOGLOBIN ONE-CARBON 3-Phosphoglycerate SERINE GLYCINE METABOLISM CYSTEINE 2-Phosphoglycerate Phosphoenolpyruvate Pyruvate ALANINE Figure 2. Glycolytic intermediates funnel into biosynthetic pathways. 4 Cite this article as Cold Spring Harb Perspect Biol 2021;13:a040535 Glycolysis 3-Phosphoglycerate can be converted into fact, these reactions do go in reverse during serine, a precursor for synthesis of the amino gluconeogenesis (the process of converting acids cysteine and glycine. pyruvate back to glucose). The three irreversible reactions are reactions 1, 3, and 10 because they Pyruvate can generate the amino acid alanine. all have a large negative ΔG and are, thus, highly favorable. Note that there is a difference between ΔG°0 WHAT DRIVES THE 10 REACTIONS and ΔG primarily because ΔG reflects both the OF GLYCOLYSIS FORWARD? ΔG°0 and the ratio of products/reactants (see The directionality of reactions is governed by Chandel 2020b for a discussion of the law of initial reactant (glucose), enzyme concentra- mass action) (Fig. 3). The products of each re- tion, and activity, as well as the ΔG defined as action are quickly removed by the following ΔG°0 + RT ln([ products]/[reactants]) (Chandel reaction in glycolysis, thus keeping an overall 2020b). Glucose concentration in the blood is favorable Gibbs free energy. For example, take typically ∼5 mM, which is at a higher concentra- a look at reaction 4, which requires a large tion than the Km of basal glucose transporters positive ΔG°0 (+23.8) to drive the reaction for- and hexokinases (step 1 of glycolysis) in most ward, resulting in catalysis of a six-carbon fruc- cells. The Gibbs free energy, ΔG, has to be either tose 1,6-bisphosphate into two three-carbon negative or close to 0 if glycolysis is to proceed in molecules, glyceraldehyde 3-phosphate and di- the forward direction. The ΔG°0 and ΔG of all 10 hydroxyacetone phosphate. However, these reactions are provided in Table 1. ΔG calcula- three-carbon molecules are quickly removed tions are based on the steady-state metabolite by the subsequent reactions of glycolysis, ensur- concentration of products and reactants of ing a low ratio between the concentrations of each reaction in red blood cells and, thus, are product and reactants and a negative ΔG. In different than the ΔG°0. A careful examination fact, we look at the cumulative free-energy of the table indicates that most of the reactions change from each reaction, and then we arrive (2, 4, 5, 6, 7, 8, and 9) are near equilibrium at a favorable overall Gibbs free-energy change because their ΔG is close to 0 (Table 1). This for glycolysis at –96 kJ/mol in erythrocytes indicates that these reactions are reversible. In (Fig. 3). Table 1. Free-energy changes of glycolytic reactions in erythrocytes ΔG°0 ΔG Glycolytic reaction step (kJ/mol) (kJ/mol) (1) Glucose + ATP → glucose 6-phosphate + ADP −16.7 −33.4 (2) Glucose 6-phosphate ⇌ fructose 6-phosphate 1.7 0 to 25 (3) Fructose 6-phosphate + ATP → fructose 1,6-bisphosphate + ADP −14.2 −22.2 (4) Fructose 1,6-bisphosphate ⇌ dihydroxyacetone phosphate + glyceraldehyde 23.8 0 to −6 3-phosphate (5) Dihydroxyacetone phosphate ⇌ glyceraldehyde 3-phosphate 7.5 0 to 4 (6) Glyceraldehyde 3-phosphate + Pi + NAD+ ⇌ 1,3-bisphosphoglycerate + 6.3 −2 to 2 NADH + H+ (7) 1,3-Bisphosphoglycerate + ADP ⇌ 3-phosphoglycerate + ATP −18.8 0 to 2 (8) 3-Phosphoglycerate ⇌ 2-phosphoglycerate 4.4 0 to 0.8 (9) 2-Phosphoglycerate ⇌ phosphoenolpyruvate + H2O 7.5 0 to 3.3 (10) Phosphoenolpyruvate + ADP → pyruvate + ATP −31.4 −16.7 Reproduced, with permission, from Nelson and Cox 2013, © W.H. Freeman and Company. ΔG°0 is the standard free-energy change. ΔG is the free-energy change calculated from the actual concentrations of glycolytic intermediates present under physiological conditions in erythrocytes, at pH 7. The glycolytic reactions bypassed in gluconeogenesis are shown in red. Biochemical equations are not necessarily balanced for H or charge. Cite this article as Cold Spring Harb Perspect Biol 2021;13:a040535 5 N.S. Chandel molecules from glucose, the first phase of 0 Cumulative free energy change (kJmol–1) glycolysis phosphorylates glucose using two ATP molecules and converts it from one six- – 20 carbon glucose molecule into two three-carbon glyceraldehyde 3-phosphate molecules. Subse- – 40 quently, glyceraldehyde 3-phosphate dehydro- genase couples NAD+ reduction to NADH to – 60 the addition of inorganic phosphate to glyceral- dehyde-3-phosphate, resulting in the generation – 80 of 1,3-bisphosphoglycerate, whereas subsequent reactions produce phosphoenolpyruvate. – 100 In the presence of oxygen, NADH and py- ruvate are transported into the mitochondria 0 1 2 3 4 5 6 7 8 9 10 where ATP is generated, as discussed in the Reactions of glycolysis next section. It is important to note that under ample oxygen conditions, glycolysis serves to Figure 3. Gibbs free-energy change in 10 steps of provide intermediates that can fuel the bio- glycolysis. The free-energy changes are based on Ta- synthetic pathways in most cells. Glycolytic ble 1. Note that the Gibbs free-energy changes in reactions 6–10 are multiplied by 2 because one mol- ATP generation is paramount when oxygen is ecule of glucose is converted into two molecules of limiting to the generation of mitochondrial-de- glyceraldehyde 3-phosphate. pendent ATP. There are exceptions, such as erythrocytes that contain no mitochondria or cells such as neutrophils and endothelial cells that contain few mitochondria. In these cases, WHY USE ATP TO MAKE ATP? the flux through glycolysis is high enough to A puzzling aspect of glycolysis is the use of 2ATP sustain both ATP generation and fueling of the in the first phase (ATP investment) and the gen- biosynthetic pathways. eration of 4ATP in the second phase (ATP pay- off ). Why consume 2ATP in the first place? WHAT HAPPENS TO THE NADH There are two explanations. First, the phos- AND PYRUVATE GENERATED phorylation of glucose using ATP makes the gly- DURING GLYCOLYSIS? colytic intermediates into phosphorylated me- tabolites, which cannot diffuse out of the cell The fate of NADH and pyruvate is intimately because of their negative charge. Second, the linked. In the presence of ample oxygen, both phosphorylated metabolites can be used for sub- pyruvate and NADH are transported into the strate-level phosphorylation, a process in which a mitochondria, where pyruvate enters the TCA phosphoryl (PO3) group is transferred from a cycle while NADH is oxidized to NAD+ by the phosphorylated metabolite, like 1,3-bisphospho- electron transport chain (see Chandel 2020a) glycerate, to ADP to generate ATP. The genera- and transported back into the cytosol to pro- tion of ATP requires +30.5ΔG°0 when bound to mote glycolysis (Fig. 4). The transport of Mg2+ and has to be coupled to reactions that have NADH and NAD+ in and out of mitochondria a large negative ΔG°0. There are two glycolytic involves intricate shuttling mechanisms (Chan- reactions that have a large negative ΔG°0 and are del 2020a), resulting in a slower regeneration of coupled to the generation of ATP. In step 7, the cellular NAD+ pools compared with the anaer- conversion of 2,3-bisphoshoglycerate to 2-phos- obic mechanism in which lactate dehydrogenase phoglycerate has a ΔG°0 of –37.6 kJ/mol, and in (LDH) reduces pyruvate into lactate by coupling step 10 the conversion of 2 phosphoenolpyr- this reaction to NADH oxidation to NAD+. uvate to 2 pyruvate has a ΔG°0 of –62.8 kJ/mol. Many of us experience a buildup of lactate To generate these three-carbon phosphate upon vigorous exercise. Under these conditions, 6 Cite this article as Cold Spring Harb Perspect Biol 2021;13:a040535 Glycolysis Glucose Glucose 6-phosphate Fructose 6-phosphate MITOCHONDRION Lactate Glyceraldehyde 3-phosphate NAD+ NAD+ NADH NADH 1,3-Bisphosphoglycerate LDH Phosphoenolpyruvate Pyruvate Acetyl-CoA Oxaloacetate TCA CYCLE Citrate NDR ION MITOC HO Figure 4. Regeneration of NAD+ by LDH or mitochondria. oxygen cannot be delivered fast enough to mus- two essential products required for beer, CO2 cle mitochondria to keep up with the high met- and ethanol (Fig. 5). Yeast make greater abolic demands placed on muscle cells. In the amounts of ethanol through glucose consump- absence of oxygen, the electron transport chain tion under anaerobic conditions compared with cannot regenerate NAD+, making the LDH aerobic conditions, a phenomenon first ob- reaction critical for NAD+ regeneration and served by Louis Pasteur. This slowing down of continuous flux through glycolysis. This is some- fermentation in the presence of oxygen is re- times referred to as anaerobic glycolysis or ho- ferred to as the Pasteur effect. The opposite molactic fermentation. The production of lactic effect, in which the presence of high levels of acid is one form of fermentation using pyruvate. glucose slows mitochondria from generating The other form of fermentation is the one most ATP under aerobic conditions, is called the of us think of when we use yeast to make beer. Crabtree effect. Although the molecular details This two-step process regenerates NAD+ with underlying the Pasteur and Crabtree effects are Cite this article as Cold Spring Harb Perspect Biol 2021;13:a040535 7 N.S. Chandel LA CTATE PYRUVATE ALCOH OL DEHYDROGE N ASE D ECARB OX Y LASE D E H Y D ROGEN ASE H H O O CH 3 CH C CH 3 C C CH 3 C CH 3 C H O– O– OH O O OH Lactate Pyruvate Acetaldehyde Ethanol NAD + NADH, H + CO 2 NADH, H + NAD + Mammals Lactate Pyruvate Yeast Lactate Pyruvate Acetaldehyde Ethanol Figure 5. Fermentation in mammals and yeast. not fully understood, the short-term regulation lytic intermediates are also precursors to bio- of the Pasteur and Crabtree effects is controlled synthetic pathways. Thus, regulating glycolytic by metabolites regulating glycolytic enzymes enzymes can balance between the energy- (see the next section), whereas the long-term generating and the biosynthetic capacity of regulation is controlled by transcription factors glycolytic intermediates. There are four hexokin- regulating expression of glycolytic enzymes ases (HKI–IV) that catalyze step 1 of glycolysis. (Chandel 2020c). HKI, -II, and -III have a low Km (