Glycolysis.pdf
Document Details
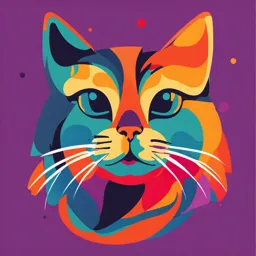
Uploaded by IntimateDatePalm
Tags
Related
Full Transcript
Tymoczko Berg Gatto Stryer Biochemistry: A Short Course Fourth Edition CHAPTER 16 Glycolysis © 2019 Macmillan Learning Why start with glycolysis? We are looking at glycolysis first out of the many metabolic pathways possible because it is found in nearly all prokaryotic and eukaryotic cells! In euka...
Tymoczko Berg Gatto Stryer Biochemistry: A Short Course Fourth Edition CHAPTER 16 Glycolysis © 2019 Macmillan Learning Why start with glycolysis? We are looking at glycolysis first out of the many metabolic pathways possible because it is found in nearly all prokaryotic and eukaryotic cells! In eukaryotic cells, this pathway occurs in the cytosol, which means that as soon as glucose is transported into the cell, the enzymes of glycolysis can immediately act on it. Glycolysis is the sequence of reactions that converts one molecule of glucose to two molecules of pyruvate. Glycolysis serves two major functions First, this pathway generates ATP. Second, a number of the intermediates of this pathway serve as building blocks for the biosynthesis of other biomolecules such as amino acids and fatty acids. We start our study with glycolysis due to its central role in metabolism. Glycolysis Learning Objectives When we have finished this section, you should be able to do the following: 1. Characterize the priority and placement of the glycolytic pathway with respect to the greater context of metabolism. 2. Detail the reactions, enzymes and intermediates of glycolysis. 3. Identify the different biological inputs and outputs of glycolysis. 4. Outline the points and mechanisms of regulation of glycolysis. The Big Picture – Central Role in Metabolizing Glucose The capturing of energy from food occurs in three stages: 1. Large molecules in food are broken down into smaller molecules in the process of digestion. 2. The many small molecules are processed into key molecules of metabolism, most notably acetyl CoA. 3. ATP is produced from the complete oxidation of the acetyl component of acetyl CoA. Digestion ofDietaryCarbohydrates Starch, Glycogen are degraded to glucose, maltose and oligosaccharides by salivary and pancreatic amylases In ruminants, cellulose in converted to glucose by cellulase. In small intestine Lactose (milk sugar) is converted to glucose and galactose by lactase (βgalactosidase) Sucrose (common sugar) is converted to glucose and fructose by sucrase (invertase) Maltose is converted to glucose by maltase Galactose and Fructose can be further converted to glucose Monosaccharides arethen transported into the cells and subsequently into the bloodstream Glucose must be transported across cellular membranes Q: What does KM mean? Q: What are normal glucose levels in the blood stream? Let’s Take an Overview of The Pathway As we watch the video pay attention and ask yourself: Where is/are steps that are likely regulated? How are high energy bonds created? What steps involve oxidation of the substrate molecule? What cofactor aids these steps? The reactions of glycolysis: https://www.youtube.com/watch?v=hDq1rhUkV-g We will see later how glycolysis through to pyruvate can result in different outputs, depending on the organism and/or the tissue and conditions. Glycolysis Can Be Divided into Two Parts 1. Stage 1 traps glucose in the cell and modifies it so that it can be cleaved into a pair of phosphorylated 3-carbon compounds. – Net input of energy. 2. Stage 2 oxidizes the 3-carbon compounds to pyruvate while generating two molecules of ATP – Net output of energy. Let’s Look at Some of The Reactions First step: phosphorylation of glucose – when glucose enters the cell, it is rapidly phosphorylated by hexokinase to form glucose-6-phosphate. This is the first step in glycolysis. Note that “kinases” transfer a phosphate to their substrate from ATP This is a very important step since phosphorylation of glucose traps it in the cell and prevents it from being transported out. Figure: Phosphorylation of glucose by hexokinase. Source: Tymoczko, J., Berg, J. & Stryer, L. (2015). Biochemistry: A Short Course (3rd ed.). New York, NY: W. H. Freeman and Company, p. 284. Permission: Courtesy of MacMillan Learning. In the 2nd step, glucose-6-P is converted to fructose-6-P. This is an isomerization reaction, meaning that there are no atoms lost, but only a rearrangement of the atoms occurs. In this case, it involves the conversion of an aldose to a ketose by the enzyme phosphoglucose isomerase. Figure: Conversion of glucose-6-P to fructose-6-P by phosphoglucose isomerase. Source: Tymoczko, J., Berg, J. & Stryer, L. (2015). Biochemistry: A Short Course (3rd ed.). New York, NY: W. H. Freeman and Company, p. 286. Permission: Courtesy of MacMillan Learning. Step 3. Fructose-6-P is phosphorylated at a second carbon to form fructose-1,6bisphosphate: this irreversible reaction is catalyzed by phosphofructokinase which is regulated allosterically. This is an important regulatory step in glycolysis that we will revisit later. Figure: Formation of fructose 1,6 –bisP by phosphofructokinase. Source: Tymoczko, J., Berg, J. & Stryer, L. (2015). Biochemistry: A Short Course (3rd ed.). New York, NY: W. H. Freeman and Company, p. 287. Permission: Courtesy of MacMillan Learning. DID YOU KNOW? The prefix bis – in bisphosphate means that two separate monophosphates are present, whereas the prefix di – in diphosphate (as in adenosine diphosphate) means that two phosphoryl groups are present and are connected by an anhydride linkage. Step 4. Cleavage of fructose 1,6-bisphosphate to two different 3-carbon molecules: In this step, fructose 1,6-bisphosphate is cleaved by aldolase to dihydroxyacetone phosphate and glyceraldehyde-3-phosphate. Figure: Cleavage of fructose-1,6-bisP by aldolase. Source: Tymoczko, J., Berg, J. & Stryer, L. (2015). Biochemistry: A Short Course (3rd ed.). New York, NY: W. H. Freeman and Company, p. 287. Permission: Courtesy of MacMillan Learning. Step 5. Note that glyceraldehyde-3-P is the molecule that proceeds directly onward in the glycolytic pathway. However, dihydroxyacetone phosphate is not wasted or lost from the pathway, since it is readily converted to glyceraldehyde-3-P by an isomerase in a reversible reaction. Figure: Interconversion of glyceraldehyde 3-P and dihydroxyacetone phosphate. Source: Tymoczko, J., Berg, J. & Stryer, L. (2015). Biochemistry: A Short Course (3rd ed.). New York, NY: W. H. Freeman and Company, p. 287. Permission: Courtesy of MacMillan Learning. Step 6. Oxidation of glyceraldehyde-3-P powers the formation of 1,3bisphosphogycerate which has high phosphoryl-transfer activity: In this step, 1,3bisphosphoglycerate is generated by the oxidation of glyceraldehyde-3-P by the enzyme glyceraldehyde-3-P dehydrogenase. Note that dehydrogenases are enzymes that catalyze redox reactions. Mixed acid anhydride bond has a high phosphoryl potential Figure: Formation of 1,3-bisphosphoglycerate from glyceraldehyde 3-P. Source: Tymoczko, J., Berg, J. & Stryer, L. (2015). Biochemistry: A Short Course (3rd ed.). New York, NY: W. H. Freeman and Company, p. 288. Permission: Courtesy of MacMillan Learning. High Energy Bonds Important Point: While the energy released by ATP hydrolysis is high enough to drive a variety of cellular functions, it is actually intermediate among biologically important phosphorylated molecules. This allows it to function as a carrier of phosphate groups as it can act both as a donor and a recipient. Step 6. Phosphoryl transfer from 1,3-bisphosphoglycerate to ADP to form ATP: This is the first energy producing step in glycolysis, and is catalyzed by phosphoglycerate kinase. ATP formed in this manner is termed substrate-level phosphorylation, since the donor of the phosphate group is a substrate in the reaction. Figure 2-7: Formation of 3-phosphoglycerate results in the production of ATP. Source: Tymoczko, J., Berg, J. & Stryer, L. (2015). Biochemistry: A Short Course (3rd ed.). New York, NY: W. H. Freeman and Company, p. 289. Permission: Courtesy of MacMillan Learning. Remember that there are actually two ATPs formed at this step for every molecule of glucose started with, since one glucose generates two 3-carbon molecules that flow this pathway. Steps 7-10. More ATP is formed as we get to the end of glycolysis: 3-phosphoglycerate is converted to 2-phosphoglycerate by phosphoglycerate mutase; 2-phosphoglycerate is then converted to phosphoenolpyruvate (or PEP) by the enzyme enolase. PEP is a high phosphoryl-transfer compound, and is able to donate its phosphate group to ADP in the last step of glycolysis catalyzed by pyruvate kinase. This is another example of substrate-level phosphorylation. Figure: Final steps in glycolysis that lead to the final product, pyruvate. Source: Tymoczko, J., Berg, J. & Stryer, L. (2015). Biochemistry: A Short Course (3rd ed.). New York, NY: W. H. Freeman and Company, p. 290. Permission: Courtesy of MacMillan Learning Net Glycolysis Reaction Now that we have discussed all of the reactions of glycolysis, we can write the net reaction for the entire pathway: Glucose + 2 Pi +2 ADP + 2 NAD+ 2 pyruvate + 2 ATP + 2 NADH + 2 H+ + 2 H2O The individual reactions of glycolysis are shown in Table 2-2 below. Note that the overall free energy change is about -22 kcal/mol. Thus, while some individual reactions are reversible, glycolysis as a pathway only flows in one direction, that being towards the production of pyruvate. Standard Conditions Cellular Conditions Irreversible Irreversible Irreversible Determining ΔG To determine ΔG, we need to take into account the nature of the reactants and the products, as well as their concentration. Consider the following reaction: A + B ←------→ C + D The ΔG of the forward reaction is given by: ΔG = ΔG° + RT ln [C][D]/[A][B] Here, ΔG° is the standard free energy change, R is the gas constant, T is the absolute temperature, and [A,B,C,D] are the molar concentrations of the reactants and products. ΔG° is the free energy change under standard conditions, that is where the reactants and products are present at 1.0 M concentrations, atmospheric pressure of 1, and the temperature is 298 K (or 25 degrees Celsius). For biochemical reactions, we have adopted an additional term, ΔG°', which refers to the standard free energy change at pH 7. Note that the pathway from glucose to pyruvate has no requirement for oxygen, which is why this is referred to as anaerobic glycolysis. We will see a bit later that much more energy can be obtained from glucose in the presence of oxygen as it allows further metabolism of pyruvate. Pyruvate Has Different Metabolic Fates If the conversion of glucose to pyruvate stopped there, and went no further, a problem would arise with the redox balance in the cell. In the reaction catalyzed by glyceraldehyde-3-P dehydrogenase, a molecule of NAD+ is consumed which is not regenerated elsewhere in the pathway. Since the cell has a limited amount of NAD+, the pathway would not continue for long unless there was a way to regenerate it. The metabolism of pyruvate achieves this, although how this occurs is variable depending on the organism and whether oxygen is available. Three Possible Fates of Pyruvate Figure: Diverse fates of pyruvate. Source: Tymoczko, J., Berg, J. & Stryer, L. (2015). Biochemistry: A Short Course (3rd ed.). New York, NY: W. H. Freeman and Company, Pyruvate can be converted to ethanol This pathway occurs in some microorganisms and in yeast, with the latter being important in the production of beer and wine. Most importantly, from a metabolic perspective, is that NAD+ is regenerated in the second step of this conversion. Note that the conversion of pyruvate to ethanol consists of a decarboxylation (loss of CO2) reaction to form acetaldehyde, and a redox reaction whereby acetaldehyde becomes reduced to ethanol from electrons donated by NADH. Figure: Ethanol is produced from pyruvate by decarboxylation and oxidation-reduction reactions. Source: Tymoczko, J., Berg, J. & Stryer, L. (2015). Biochemistry: A Short Course (3rd ed.). New York, NY: W. H. Freeman and Company, p. 292. Permission: Courtesy of MacMillan Learning. Pyruvate can be directly converted to lactate In this pathway, which consists of only one reaction, NADH is used to reduce pyruvate to lactate, in the process regenerating NAD+. Thus, the conversion of glucose to lactate is also redox neutral i.e., no net oxidation-reduction. Figure: Lactate is formed by the reduction of pyruvate. Source: Tymoczko, J., Berg, J. & Stryer, L. (2015). Biochemistry: A Short Course (3rd ed.). New York, NY: W. H. Freeman and Company, p. 293. Permission: Courtesy of MacMillan Learning. Pyruvate to Lactate This anaerobic pathway occurs in some microorganisms (i.e. lactic acid bacteria, like those used to make yogurt) Moreover, pyruvate to lactate also occurs in muscle when the oxygen supply becomes limiting, requiring the muscles to function anaerobically for short periods. As lactate levels build up in the muscle, muscle fatigue sets in. This occurs because the lactate lowers the pH of the muscle to as low as 6.3 in extreme exercise, which inhibits phosphofructokinase, the main regulatory point of glycolysis. This slows the ability of muscle to metabolize glucose for energy purposes, and fatigue sets in. Pyruvate to Acetyl CoA Pyruvate can also be converted to acetyl CoA. This is the pathway whereby the most energy is obtained from pyruvate, since acetyl CoA is the entry point into the citric acid cycle and the electron transport chain, which oxidizes glucose all the way to CO2 and H2O in an aerobic manner. You will look at this pathway later. Note that NAD+ is not regenerated in this reaction but rather more is consumed. As You will see in later sections, NAD+ is regenerated by the electron transport chain. Pyruvate to Acetyl CoA Figure : Conversion of pyruvate to acetyl CoA. Source: http://schoolbag.info/chemistry/mcat_biochemistry/62.html Permission: This material has been reproduced in accordance with the University of Saskatchewan interpretation of Sec.30.04 of the Copyright Act. Fructose and Galactose are Converted into Glycolytic Intermediates While the main sugar metabolized by glycolysis is glucose, there are two other sugars that are worth examining since they are commonly present in our diet. These are fructose and galactose. Fructose is a component of sucrose, or table sugar, as well as in corn syrup which is used as a sweetener in many foods and drinks. Galactose is a component of lactose, known as milk sugar. Both of these sugars are metabolized by glycolysis, although they enter the pathway at different points. Galactose: is converted to glucose-6-P by a series of four reactions and thus enters glycolysis at the point shown. The reactions involve: the phosphorylation of galactose (1-P); the transfer of galactose 1-phosphate to an activated carrier (UDP); and then the rearrangement of one hydroxyl group on galactose 1phosphate to form glucose 1phosphate by an epimerase enzyme, which is an isomerase that repositions hydroxyl groups. Figure: Entry points in glycolysis for fructose and galactose. Source: Tymoczko, J., Berg, J. & Stryer, L. (2015). Biochemistry: A Short Course (3rd ed.). New York, NY: W. H. Freeman and Company, Fig.16.7, p. 295. Permission: Courtesy of MacMillan Learning. The product, glucose-1-P, is then converted to glucose-6-P by an isomerase called phosphoglucomutase. Clinical Insight – Lactose Intolerance About 75% of the world population is lactose intolerant, or has trouble metabolizing lactose found in milk and milk products. It is due to a deficiency in the enzyme lactase found in our small intestine, which breaks down lactose into glucose and galactose. Let’s watch this short that visually describes the problem and resulting gastrointestinal effects. https://www.youtube.com/watch?v=w_KR6k6YIIs Fructose Metabolism While the previous diagram showed two entry points for fructose into glycolysis, the vast majority is metabolized by the liver so we will focus our attention on that route. In liver, fructose is first phosphorylated to fructose -1-P by fructokinase. This is then split into two 3carbon sugars, dihydroxyacetone phosphate and glyceraldehyde by a special aldolase, fructose 1-phosphate aldolase. Figure: Fructose metabolism. Source: Tymoczko, J., Berg, J. & Stryer, L. (2015). Biochemistry: A Short Course (3rd ed.). New York, NY: W. H. Freeman and Company, Fig.16.8, p. 295. Permission: Courtesy of MacMillan Learning. Fructose Metabolism While dihydroxyacetone phosphate is an intermediate of glycolysis and thus can directly enter the pathway, glyceraldehyde has to be phosphorylated to glyceraldehyde-3P in order for it to enter glycolysis. This is carried out by triose kinase using ATP as the phosphate donor. Figure: Fructose metabolism. Source: Tymoczko, J., Berg, J. & Stryer, L. (2015). Biochemistry: A Short Course (3rd ed.). New York, NY: W. H. Freeman and Company, Fig.16.8, p. 295. Permission: Courtesy of MacMillan Learning. Clinical Insight: Excessive Fructose Consumption can lead to obesity, fatty liver, and type 2 diabetes Excess fructose consumption has been linked to obesity, fatty liver, and the development of type 2 diabetes. Because of the manner in which fructose is processed in the liver, the key regulatory enzyme of glycolysis, phosphofructokinase, is bypassed. Excess acetyl CoA is synthesized and converted to fats. Figure : The discoloration of fatty liver due to large amounts of fat deposits. Source: http://www.drsharma.ca/wp-content/uploads/sharma-obesity-fattyliver-disease1.jpg. Friday – Regulation of Gylcolysis The Glycolytic Pathway is Tightly Regulated Concepts to Remember: 1. Glycolysis has two roles: to degrade glucose to generate ATP, and to provide building blocks for biosynthetic processes (fatty acids, amino acids, glycogen). 2. Enzymes the catalyze irreversible reactions are potential sites of control. Glycolysis has three such enzymes: hexokinase, phosphofructokinase, and pyruvate kinase. Each of these enzymes has their catalytic activity increased or decreased by the binding of allosteric effectors or through covalent modification. We also need to consider the particular tissue in which glycolysis is occurring, and the metabolic role it plays in that tissue as this varies. Thus, we will consider the regulation of glycolysis in muscle and liver, since the role of glycolysis in these two tissues has several fundamental differences. Muscle In muscle glycolysis provides ATP that is used for muscle contraction. Not surprisingly, glycolysis is primarily controlled by the energy state of the cell, which is represented by the ATP:AMP ratio. The higher the ratio, the greater the energy state and less need for glycolysis; the lower the ratio, the lower the energy state and thus more need for glycolysis. The enzyme phosphofructokinase (PFK) is the most important control point in mammalian glycolysis, regardless of the tissue. PFK is controlled allosterically. ATP binds to a site on this enzyme, and not surprisingly inhibits its catalytic activity by decreasing the enzyme’s affinity for one of its substrates, fructose-6-P. (ATP:AMP ratio) AMP competes with ATP for the same site, but when it itself is bound, does not inhibit the enzyme (ATP:AMP raio). A drop in pH in the muscle also inhibits PFK by enhancing the effect of ATP. (during lactic acid buildup) Muscle Why isn’t hexokinase (as the first reaction of glycolysis, and an irreversible reaction) the control point in the pathway? It turns out that the phosphorylation of glucose to glucose-6-phosphate, while being the first step in glycolysis, is not the first committed step for this pathway. That’s because glucose-6-P can enter other pathways, including the pentose phosphate pathway and glycogen synthesis Muscle Pyruvate kinase, which catalyzes the last and irreversible step in glycolysis, is the third enzyme that is regulated in muscle glycolysis. Not surprisingly, it is allosterically inhibited by ATP and activated by fructose-1,6-bisP. The inhibition by ATP makes sense from an energy state perspective. But why activation by fructose-1,6-bisP? This metabolite is an intermediate in glycolysis, and the product of phosphofructokinase, the major rate-limiting enzyme. A rise in fructose-1,6-bisP is a clear indication of an increased flux through the pathway, and thus it makes sense that pyruvate kinase activity would be increased to handle the increased flux that is headed in its direction. (It’s a bit like opening up Gardiner Dam ahead of a heavy spring melt in the Rocky Mountains to handle the anticipated extra flow of water.) Figure: Regulation of glycolysis in muscle during rest and exercise. Source: Tymoczko, J., Berg, J. & Stryer, L. (2015). Biochemistry: A Short Course (3rd ed.). New York, NY: W. H. Freeman and Company, Fig.16.13, p. 301. Permission: Courtesy of MacMillan Learning. Liver Liver obviously does not need energy for contraction, and in fact it obtains most of its energy needs from the breakdown of fatty acids (more in a later module). So what is the purpose of glycolysis in this tissue if it is not for energy production, and what impact does this have on how glycolysis is regulated? One of the major functions of the liver is to maintain glucose levels in the blood. The glucose that it takes up is either stored as glycogen (a polymer of glucose); used to generate reducing power in the form of NADPH which is used for biosynthesis (this is done by the pentose phosphate pathway); or converted via glycolysis to molecules that serve as building blocks for the synthesis of other biomolecules. Thus, the regulation of glycolysis is different than in muscle. Liver Phosphofructokinase (PFK) is still the major point of regulation similar to muscle, however the specifics of its regulation are different. ATP and pH, the major regulators of PFK in muscle, play less of a role because it doesn’t have the sudden intense need as does contracting muscle, and no lactate is formed in liver. Because glycolysis in liver is primarily used to provide building block molecules, signals indicating whether these building blocks are abundant or scarce are the primary signals. Citrate is a major inhibitor of PFK in liver, since citrate is formed from acetyl CoA which in turn is a product of pyruvate metabolism. Thus, a high level of citrate is an indicator that biosynthetic precursors are at sufficient levels, and metabolizing more glucose through glycolysis can be slowed. Liver Glycolysis in the liver is able to respond to high blood sugar in a very unique way. When glucose rises in the blood after a meal, the liver takes up much of this; as a result, there is more flux through glycolysis which leads to build up of fructose-6-P levels. The enzyme PFK obviously needs to be activated to handle the increase in this metabolite. One way this is achieved is that some of the fructose-6-P is converted to fructose-2,6bisP (rather than fructose-1,6-bisP) Fructose-2,6-bisP is an allosteric activator of PFK, It functions by increasing PFK’s affinity for one of its substrates and by blunting the inhibitory effect of ATP. In this way, glycolysis is accelerated when glucose is abundant. This is called feedforward stimulation. Fructose-2,6-bisP will be discussed in more detail in a future section. Hexokinase Is Regulated In the Liver Liver has a unique isoform of hexokinase, called glucokinase, that phosphorylates glucose once it gets transported into the cell. Glucokinase is unique from muscle hexokinase in two key ways. 1. Glucokinase has a 50-fold higher Km for glucose than hexokinase, which means that glucose-6-P is formed only when glucose is abundant, such as after a meal. It also gives the brain and muscle first dibs at the glucose, but allows liver to take up what isn’t needed by the other cells in the body and either store it as glycogen or metabolize it to other compounds such as fatty acids and amino acids. 2. Glucokinase is not inhibited by its product, glucose-6-P. This feature also facilitates the liver to be able to use the excess glucose that it takes up from the blood so it is not wasted