GIM 201 Lesson 1 - Intro and DNA PDF
Document Details
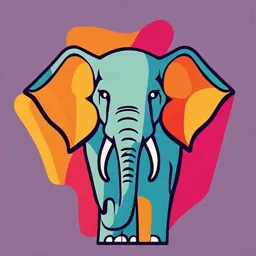
Uploaded by SereneChrysoprase640
University of Kurdistan Hewlêr
Tags
Summary
This document provides a lesson on DNA structure, replication, and how it's compacted within the cell. It details various types of DNA (like dispersed repetitive DNA, SINEs, LINEs), along with important regulatory mechanisms like histone tail modifications and their impact on gene expression.
Full Transcript
phosphate on CS it would Bases so This slide explains the different types of DNA in the human genome and how they are distributed. Here’s a simpler breakdown: 1. Types of DNA: Dispersed repetitive DNA: Makes up about 45% of the genome. These sequenc...
phosphate on CS it would Bases so This slide explains the different types of DNA in the human genome and how they are distributed. Here’s a simpler breakdown: 1. Types of DNA: Dispersed repetitive DNA: Makes up about 45% of the genome. These sequences are repeated in various places but not clustered. SINEs (Short Interspersed Elements): These are short DNA sequences, 90–500 base pairs (bp) long. A common example is the Alu element, which makes up about 10% of the genome. LINEs (Long Interspersed Elements): These are longer sequences, up to 7,000 bp long, scattered throughout the genome. Exons: These are the coding parts of the DNA that are used to make proteins. Surprisingly, they only make up 1-2% of the genome. 2. Other elements: Introns: Non-coding regions within genes. Tandem repeats and Alpha-satellite DNA: Specific sequences repeated many times in a row, often found in important structural parts of chromosomes (e.g., centromeres). Other dispersed repeats: Include transposons and other repetitive DNA. 3. Pie Chart: This shows the proportion of each DNA type in the genome. Most of the genome is made up of repetitive sequences, not coding DNA. 4. Illustrations: The first bar shows single-copy DNA, which is unique and scattered (45% of the genome). The second bar represents dispersed repetitive DNA (also 45%). The third bar shows satellite DNA, which clusters in specific areas (10%). In short, only a tiny portion of your DNA codes for proteins. Most of it consists of repeated sequences with various functions or unknown roles. Here’s a simpler explanation. 1. DNA Types: Repetitive DNA: Makes up almost half of your DNA (45%). These are repeated sequences scattered throughout your genome. SINEs (Short Repeats): Small pieces of DNA, like the Alu element, which is very common and makes up about 10% of your DNA. LINEs (Long Repeats): Longer pieces of DNA, up to 7,000 letters long, also scattered around. Exons: The useful part of DNA that makes proteins. This is only 1-2% of your whole DNA! 2. Other Stuff: Introns: Extra pieces inside genes that don’t make proteins. Tandem Repeats and Alpha-satellite DNA: Repeated DNA found in specific spots, like the centers of chromosomes. 3. Big Picture: Most of your DNA (90%) is repetitive or doesn’t code for proteins. Only a tiny bit (1-2%) actually makes the proteins your body needs. Think of your DNA like a big library: only a few books (the exons) are useful for instructions, while most of the library is filled with repeated books and extra material. Why compaction is necessary: If we stretched out all the DNA in one cell, it would be about 2 meters long! To fit inside a tiny cell nucleus, DNA is tightly packed into structures called chromatin. How DNA is compacted: 1. Nucleosomes: DNA wraps around 8 proteins called histones to form a nucleosome. Each nucleosome is about 150 base pairs of DNA, with 20-60 base pairs of linker DNA connecting them. 2. Solenoid Structure: Nucleosomes coil together, forming a solenoid, which looks like a spring with 6 nucleosomes per turn. 3. Chromatin Loops: The solenoid coils further into loops, about 100 kilobase pairs each, supported by scaffold proteins. Key Takeaway: This organization is efficient and allows DNA to be easily accessed when needed for replication or transcription while still fitting into the nucleus. pngs.at This slide is about histone tail modifications, which control how tightly or loosely DNA is packed, affecting whether genes can be turned on or off. Here’s the simple explanation: 1. What are histones? DNA wraps around proteins called histones, like thread around a spool. Histones have “tails” that can be modified to either loosen or tighten how DNA is wrapped. 2. How do modifications work? The tails of histones are positively charged, so they attract DNA (which is negatively charged), making the DNA tightly wrapped. Certain enzymes can modify these tails: Acetylation: Adds an “acetyl” group to the tail, which weakens its grip on DNA. This makes the DNA looser and allows genes to be active (transcription can happen). Methylation: Adds “methyl” groups, which can make the DNA tighter and inactive (genes are silenced). 3. Key Enzymes: HATs (Histone Acetyltransferases): Add acetyl groups to loosen DNA and make genes active. HDACs (Histone Deacetylases): Remove acetyl groups to tighten DNA and turn genes off. HMTs (Histone Methyltransferases): Add methyl groups to silence genes. Iii L 4. Two States of DNA: Active (euchromatin): DNA is loose, and genes can be read (transcribed). Inactive (heterochromatin): DNA is tightly packed, and genes are silent. In short: Histone modifications act like a switch, controlling whether genes are “on” or “off” by changing how tightly DNA is wrapped around histones. DNA replication is the process of copying DNA to ensure that each new cell gets an exact genetic copy during cell division. 1. Overview: DNA replication is semi-conservative: each new DNA molecule has one old strand and one new strand. 2. Steps of DNA Replication: Unwinding: DNA helicase separates the double helix into two single strands. This creates a replication fork. Stabilizing the strands: Single-stranded binding proteins (SSBs) keep the strands apart and stable. Primer synthesis: DNA primase makes a short RNA primer to provide a starting point for DNA polymerase. Elongation: DNA polymerase adds new nucleotides to the 3’ end of the primer, building the new DNA strand. The leading strand is synthesized continuously, while the lagging strand is synthesized in short fragments (Okazaki fragments). Joining fragments: DNA ligase seals gaps between Okazaki fragments on the lagging strand. 3. Proofreading and Error Correction: DNA polymerase has a proofreading function that checks and fixes errors during replication. This keeps the error rate extremely low (about 1 error per billion nucleotides). 4. Supercoiling Problem: As the DNA unwinds, supercoiling occurs ahead of the replication fork. Topoisomerases solve this: Type I: Makes single-strand nicks to relieve tension. Type II: Makes double-strand breaks to untangle DNA. Key Takeaways: Histone Tail Modification regulates gene activity by loosening or tightening DNA packaging. DNA Replication ensures precise duplication of DNA with high fidelity, using enzymes like helicase, polymerase, and ligase, while addressing structural challenges like supercoiling. Each incoming nucleotide has three phosphate groups. When the new nucleotide attaches, two phosphates (called pyrophosphate) are removed. This releases energy needed to create the bond. 8 DNA polymerase Here’s a simplified explanation of the points in the image: 1. DNA replication is highly accurate: Mistakes during copying (replication) happen only about once every 10 billion nucleotides (the building blocks of DNA). 2. Mechanisms to fix errors: DNA polymerase, the enzyme that builds new DNA strands, has ways to check and fix errors. 3. Pause for correction: If the wrong nucleotide is added, the process pauses, giving the enzyme a chance to fix the mistake. 4. Proofreading activity: DNA polymerase has a “proofreading” function. It can find and remove incorrectly paired nucleotides using a process called exonuclease activity. 5. Exonuclease activity: This means the enzyme can cut out nucleotides from the end of the DNA strand if they are incorrect. 6. Endonuclease activity: Sometimes, the enzyme can also cut out nucleotides from inside the DNA strand if needed. 7. Improved accuracy: This proofreading and cutting function improves the accuracy of DNA replication by 100 to 1,000 times. In short, DNA polymerase acts like a careful editor, checking for errors during DNA replication and fixing them to ensure the DNA copy is almost perfect. Key source error Exactly the same molecular make up but just a different shape 101000 10100,0 1 10,000 InN DNA Pathway A smallcut isusedtojointothing together 2. Step 1 (ATP used): DNA ligase uses ATP to activate the phosphate group at the nick. 3. Step 2 (AMP released): DNA ligase then forms a new bond between the 3′- OH and 5′-phosphate, sealing the nick and making the DNA strand continuous. This image shows how single-stranded DNA-binding proteins (SSBs) help during DNA replication: 1. Unwound DNA is unstable: After DNA is unwound, the single-stranded DNA can form tangles or “hairpins” due to base pairing within the strand. 2. SSBs bind to single-stranded DNA: These proteins attach to the unwound DNA to prevent it from folding back on itself. 3. Straightening the DNA: The SSBs stabilize the single-stranded DNA and keep it straight, making it easier for DNA polymerase to copy the strand. In short, SSBs keep the unwound DNA organized and ready for replication. This figure explains how Type I DNA Topoisomerase helps relieve strain in a DNA molecule during processes like replication and transcription. Here’s a simplified step-by-step explanation: Key Steps in the Action of Type I Topoisomerase: 1. Problem: DNA Strain DNA is a double helix, and when tightly coiled, strain builds up because the two ends of the DNA can’t rotate freely. 2. Enzyme Action Begins Type I Topoisomerase binds to the DNA. The enzyme has an active site with a tyrosine amino acid, which plays a key role in the reaction. 3. Breaking a DNA Strand The tyrosine in the enzyme breaks one strand of DNA by cleaving the phosphodiester bond (the backbone of DNA). The tyrosine forms a temporary bond with the DNA phosphate group, holding the broken strand in place. 4. Relieving the Strain The break allows the two ends of the DNA to rotate relative to each other. This rotation relieves the torsional (twisting) strain caused by tight coiling. 5. Rejoining the DNA Strand The enzyme uses the stored energy in the bond between tyrosine and the DNA phosphate to reconnect the DNA strand. This makes the process reversible and ensures no energy is wasted. 6. Completion The DNA is now relaxed, and the enzyme detaches, leaving the DNA intact and free of strain. Summary of Key Features in the Figure Left Panel (Steps 1–3): Shows the DNA strain, the enzyme binding, and the breaking of one DNA strand. The red arrow indicates how tyrosine interacts with the DNA phosphate to cleave it. Right Panel (Steps 4–6): Explains how the DNA rotates to relieve strain. The enzyme reforms the original phosphodiester bond, releasing the DNA in its relaxed state. This process ensures that DNA remains functional during replication or transcription without breaking under excessive strain. This figure shows how Type II DNA Topoisomerase untangles two interlocked DNA double helices by cutting and rejoining DNA. Here’s an easy-to-understand explanation: Step-by-Step Process 1. Interlocked DNA Molecules Two circular DNA double helices (shown as red and orange) are tangled together, creating a problem for cellular processes. 2. Topoisomerase II Binds to DNA The enzyme Type II Topoisomerase attaches to one of the DNA double helices (orange). It forms a reversible covalent bond with both strands of the orange DNA, temporarily breaking it and creating a “protein gate.” 3. DNA Untangling The protein gate opens, allowing the other DNA double helix (red) to pass through the break. 4. Rejoining the DNA The enzyme reseals the break in the orange DNA using stored energy, restoring its intact double-helix structure. 5. Final Outcome The two DNA double helices (red and orange) are now untangled and separated, ready for further cellular processes like replication or transcription. Key Points Type II Topoisomerase cuts both strands of one DNA molecule. It allows another DNA molecule to pass through. Finally, it reseals the DNA, using a reversible reaction to restore its structure. This process ensures DNA strands remain functional without being damaged or tangled.