General Protein Metabolism NUB 2022 PDF
Document Details
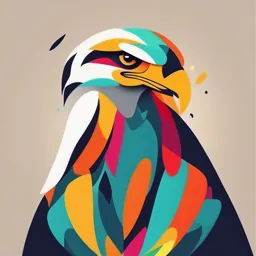
Uploaded by WarmheartedOlive
Nahda University
Tags
Summary
This document provides a comprehensive overview of protein metabolism, covering topics like biological value, essential amino acids, and different pathways of protein turnover. It explores nitrogen balance and the fate of absorbed amino acids, including anabolic pathways and the urea cycle.
Full Transcript
GENERAL ASPECTS OF PROTEIN METABOLISM Proteins are macromolecules composed of polymers of covalently linked amino acids. They are involved in every cellular process such as: 1. Enzymatic catalysis 2. Transport and storage. 3. Musculo-skeletal coordination. 4. Immune system functions....
GENERAL ASPECTS OF PROTEIN METABOLISM Proteins are macromolecules composed of polymers of covalently linked amino acids. They are involved in every cellular process such as: 1. Enzymatic catalysis 2. Transport and storage. 3. Musculo-skeletal coordination. 4. Immune system functions. 5. Generation and transmission of nerve impulse. 6. Control of growth and differentiation. Biological Value of Proteins: The biological value of a protein means its ability to satisfy the requirement of the body for proteins and to maintain its nitrogen balance. To be of high biological value the protein must be easily digested and should contain all the essential amino acids in adequate amounts. Accordingly, proteins can be classified into high biological value protein and low biological value protein. 1-Animal proteins are generally of high biological value. They are found in eggs, milk and its products, meat, fish and poultry. 2-Plant proteins are generally of lower biological value than animal proteins. They are found in legumes, cereals, nuts, and tubers. A variety of proteins of low biological value may give a mixture of high biological value. - Essential Amino Acids: They are not formed in the animal body. Their deficiency decreases the rate of growth and protein synthesis (produces negative nitrogen balance). It is essential to supply them in diet. They are 9 in number: valine, leucine, isoleucine, threonine, methionine, lysine, phenylalanine, tryptophan and histidine. - Half-essential or Semi-essential Amino Acids: They are formed in the body at a rate enough for adult but not for growing animals. Arginine is the only half-essential amino acid. - Non-essential Amino Acids include the rest of amino acids. - The recommended daily allowance of dietary protein of normal healthy adult is 0.8g/kg body weight. It increases during growth, lactation, pregnancy and convalescence. - The caloric value of protein is 4kcal/g which is nearly equal to that of carbohydrates and less than half that of fat (9 kcal/g). The presence of carbohydrates and fats in the diet spares proteins for oxidation and production of energy. Protein Turnover: - The continuous degradation and synthesis of cellular proteins occur in all forms of life. Each day, humans turn over 1–2% of their total body protein, principally muscle proteins. - High rates of protein degradation occur in tissues that are undergoing structural rearrangement, for example, uterine tissue during pregnancy, skeletal muscles in starvation. - Of the liberated amino acids, 75% approximately are reutilized. NITROGEN METABOLISM Nitrogen forms about 16% of proteins. An average protein intake of an adult is about 90 gm daily i.e. 14.5 g of nitrogen. Food contains very small amounts of other nitrogenous compounds, e.g. nucleic acids, phospholipids, and glycosaminoglycans. The Nitrogen Balance It is the quantitative difference between the nitrogen intake and output. Since most of the nitrogen of the diet is protein nitrogen, and most of the nitrogenous excretory products are derived from protein catabolism, the balance between the two represents the balance between protein anabolism and catabolism. Three states may exist: 1- Nitrogen balance It exists when output equals intake. It occurs in the normal healthy adult on an adequate diet. 2- Positive nitrogen balance It exists when intake exceeds output. It occurs whenever new tissues are built e.g. during growth, pregnancy, muscular training, and convalescence from states of negative nitrogen balance. 3- Negative nitrogen balance This may be due to one of the following causes: a) Inadequate protein intake. This occurs in cases of starvation, malnutrition, deficiency of one or more of the essential amino acids and gastrointestinal diseases. b) Loss of protein. This occurs in cases of chronic hemorrhage, albuminuria, and during lactation on an inadequate diet. c) Increased protein catabolism. This occurs in cases of diabetes mellitus, Cushing's syndrome, hyperthyroidism, and infectious fevers. Fate of Absorbed Amino Acids: Absorbed amino acids are mixed with the amino acids produced from hydrolysis of body protein and those synthesized in the body to form a common amino acid pool. This pool is drawn upon for anabolism and for catabolism of amino acids. Anabolic Fate: - These include the synthesis of proteins e.g. tissue, milk, and plasma proteins, enzymes, and some hormones. - They also include the synthesis of other nitrogenous substances e.g. glutathione, adrenaline, thyroxine, melanin, niacin, purines, pyrimidines, aminosugars, and the nitrogenous bases of phospholipids. Catabolic Fate: - Most catabolic reactions are preceded by cleavage of the amino acids into ammonia and the carbon skeleton (usually in the form of an -keto acid). - The ammonia is mostly converted to urea, which is excreted in urine. - Little ammonia is also excreted in urine. The carbon skeleton may be converted to glucose or ketone bodies, or may be oxidized to carbon dioxide and water. Fate of the Carbon Skeleton of Amino Acids (α- keto acids) 1-Glucogenic amino acids The carbon skeleton of these amino acids after deamination can give pyruvate or any of the intermediates of the citric acid cycle, which can yield glucose by gluconeogenesis. They are called glucogenic. Most of amino acids (except the members of the other two groups) are considered as pure glucogenic amino acids e.g. alanine, glycine, serine, cysteine, aspartate and glutamate. 2- Ketogenic amino acids The carbon skeleton of these amino acids after deamination can give acetyl-CoA or acetoacetyl-CoA. This group includes only leucine and lysine. 3- Both glucogenic and ketogenic amino acids: The carbon skeleton of these amino acids after deamination can give both glucose and ketone bodies. They include only phenylalanine, tyrosine, tryptophan and isoleucine. REMOVAL OF AMINO ACID NITROGEN Most of the catabolic pathways of amino acids begin with the removal of the amino group from the carbon skeleton of the amino acids with production of the corresponding - keto acids. -Removal of amino acid nitrogen in humans ends by urea formation in the following steps: transamination with α-ketoglutarate to form glutamate, oxidative deamination of glutamate and finally synthesis of urea and its excretion. Removal of Amino Acid Nitrogen Transamination -amino acid -keto acid -ketoglutarate Glutamate Urea Synthesis Transamination Oxidative deamination Urea NH3 CO2 TRANSAMINATION Transamination is the transfer of an amino group usually from an -amino acid to an -keto acid forming a new -keto acid and a new -amino acid. The enzymes that catalyze this type of reaction are called aminotransferases (transaminases). Transamination Reaction R1 – CH – COOH Aminotransferase R1 – C – COOH NH2 O PLP -Amino acid -Keto acid R2 – C – COOH R2 – CH – COOH O NH2 -Keto acid -Amino acid -They are present in the cytosol and mitochondria of all tissues especially the liver. -The reaction is reversible, so it is important in deamination and in the biosynthesis of the nonessential amino acids. It requires pyridoxal phosphate (PLP) as intermediate carrier of the amino group. -Several transaminases exist, each specific for one amino acid and the corresponding -keto acid. -Ketoglutarate acts as an amino group acceptor in all transamination reactions, forming glutamate. Thus, glutamate, acts as a “collector” of nitrogen from amino acids. -Aminotransferases are named after the specific amino group donor, because the acceptor of the amino group is almost always -ketoglutarate. -Transamination involves neither the uptake nor the release of free ammonia. All amino acids can be transaminated except lysine, threonine and proline. -The most two important and active aminotransferase reactions are catalyzed by alanine aminotransferase (ALT) and aspartate aminotransferase (AAT or AST). Reaction of Alanine Aminotransferase (ALT) COOH ALT COOH H2NCH C=O PLP CH3 CH3 Alanine -KG Glutamate Pyruvate Reaction of Aspartate Aminotransferase (AST) CH2COOH AAT CH2COOH H2NCH-COOH PLP O=C-COOH Aspartate Oxaloacetate -KG Glutamate Diagnostic Value of Plasma Aminotransferases Aminotransferases are normally intracellular enzymes, with the low levels found in the plasma representing the release of cellular contents during normal cell turnover. The presence of elevated plasma levels of aminotransferases indicates damage to cells rich in these enzymes. Two aminotransferases AAT and ALT are of particular diagnostic value, they are elevated in nearly all liver diseases, e.g. severe viral hepatitis and toxic injury. Aminotransferases may be also elevated in non-hepatic disease, such as myocardial infarction and muscle disorders. Oxidative Deamination There are 2 enzymes concerned with oxidative deamination of amino acids, they are: L- amino acid oxidase and L-glutamate dehydrogenase L-Amino Acid oxidase -This enzyme is a flavoprotein containing FMN. It is of low activity. It works only in the liver and kidneys. -It acts on L-amino acids with production of the corresponding α-keto acids, NH 3 and H2O2. The latter is converted by catalase enzyme into H2O. Reaction of L-Amino Acid Oxidase R – CH – COOH L-amino acid oxidase R – C – COOH NH2 NH -Amino acid FMN FMNH2 -Imino acid H2O Spontaneously H2O2 O2 NH3 X2 Catalase R – C – COOH 2H2O O -keto acid L-Glutamate dehydrogenase (GDH) -It is a mitochondrial enzyme. This enzyme is NAD(P)+-dependent dehydrogenase that converts L-glutamate into -Ketoglutarate. -It is of high activity and widespread distribution in tissues. -It is an essential component of transdeamination reaction, therefore, important for the deamination of most amino acids. -The reaction is reversible, therefore, it catalyzes resynthesis of L-glutamate by the use of free ammonia and NAD(P)H. Reaction of L-Glutamate Dehydrogenase CH2COOH L-Glutamate dehydrogenase CH2COOH CH2 CH2 H2NCH-COOH O=C-COOH NAD(P)+ NAD(P)H,H+ Glutamate H2O NH3 -Ketoglutarate Transdeamination -As noted before, removal of ammonia from amino acids cannot be explained by transamination alone (no free ammonia is liberated), nor by oxidative deamination alone (works efficiently only on L-glutamate). It can be explained by a combination of the two mechanisms, as follows: -First transamination occurs from an amino acid to -ketoglutarate, forming glutamate. -This is followed by oxidative deamination of glutamate, regenerating -ketoglutarate, which can be reutilized in a new transamination reaction. Reaction of Transdeamination -amino acid -ketoacid Aminotransferase PLP CH2COOH CH2COOH CH2 CH2 O=C-COOH H2NCH-COOH -Ketoglutarate L-Glutamate Glutamate Dehydrogenase NH3 + NAD(P)H, H+ H2O + NAD(P)+ Specific Methods of Amino Acid Deamination These methods are specific for certain amino acid, which include the following: Specific Methods of Amino Acid Deamination 1- Glycine cleavage system (Glycine CH2=THF+NH3+CO2) 2- Glycine oxidase (Glycine glyoxalate+NH3+H2O2) 3- Serine dehydratase (Serine pyruvate) 4- Cysteine desulfhydrase (Cysteine pyruvate) 5- Homoserine deaminase (Homoserine -ketobutyrate) 6- Threonine dehydratase (Threonine -ketobutyrate) 7- Histidase (Histidine Urocanate) 8- Hydrolytic deamination - Glutaminase (Glutamine Glutamate) - Asparaginase (Asparagine Aspartate) 9- Reductive deamination: It occurs mainly in large intestine by the action of intestinal bacteria on amino acids (putrefaction) with production of the corresponding organic acids. METABOLISM OF AMMONIA Sources of Ammonia - Deamination of amino acids. - Other minor sources include the deamination of purines and pyrimidines as well as the catabolism of the latter. - Bacterial putrefaction in the intestine produces small amounts of ammonia, which become of great importance in cases of liver cirrhosis and hepatic coma. Fate of Ammonia I- Anabolic pathways 1-The ammonia removed by deamination may be used for the synthesis of non-essential amino acids from α keto acids by reversal of transdeamination. 2- Synthesis of glutamine. The amide group of glutamine may be used for synthesis of asparagine and other nitrogenous compounds as purines, pyrimidines and amino sugars. II- Catabolic and excretory pathways -Although ammonia is constantly produced in the tissues, it is present at very low levels in blood. -This is due both to: the rapid removal of blood ammonia by the liver and to the fact that several tissues, particularly the brain, dispose ammonia in the form of glutamine. 1. Urea: Formation of urea in the liver is quantitatively the most important disposal route for ammonia. Urea travels in the blood from the liver to the kidneys, where it passes into the urine. 2. Glutamine: Glutamine is synthesized from glutamate and ammonia by glutamine synthetase. It is the major mechanism for the removal of ammonia in the brain. Glutamine is also synthesized in the skeletal muscle, the liver and other extrarenal tissues. -In liver: glutamine, either synthesized in the liver or received from extrahepatic tissues. It is deaminated to glutamate by glutaminase then it is converted to α-ketoglutarate and ammonia by glutamate dehydrogenase (GDH). The ammonia produced by both enzymes is removed by urea synthesis. -In the kidneys: Glutamine received from the brain and other tissues is degraded by glutaminase to form glutamate and release ammonia. The released ammonia is excreted in urine representing 60% of the total urinary ammonia. The remaining 40% of urinary ammonia comes from deamination of amino acids in the kidney. N.B. A good part of the amino acid nitrogen in muscles and gut is converted to alanine by ALT, then it is transported to the liver (glucose-alanine cycle). In liver by transdeamination, ammonia is removed for urea synthesis. Catabolic and Excretory Pathways of Ammonia Glutamine Liver Glutaminase Extrahepatic Tissues NH3 NH3 NH3 Glutamate NADP+ Urea α-KG Glutamate Glutamine GDH ATP ADP+Pi NH3 NADPH,H+ NADP+ NADPH,H+ GDH Glutamine α-KG synthetase Kidneys Urine Alanine Liver Muscles NH3 α-Amino acid α-KG Alanine Pyruvate Transaminase ALT Urea Gluconeogenesis α-Keto acid Glutamate Pytuvate Glucose Gycolysis Glucose Kidneys Urine Urea Cycle Definition and Importance This is the principal pathway of disposal of ammonia resulting from the deamination of amino acids, in a neutral non-toxic form. Urea is an end product that is excreted in urine. Site Urea formation occurs only in the liver as arginase is present only in the liver. The first 2 reactions occur in the mitochondria and all subsequent reactions occur in the cytosol. Steps The overall reactions of urea cycle are as follows: Aspartate + NH3 + CO2 + 3ATP Urea + fumarate + 2ADP + 2 Pi +AMP + PPi Reactions of Urea Cycle NH3 + CO2 + ATP ATP + Carbamoyl phosphate N-acetyl-glutamate synthetase I ADP + Pi ADP O NH2 – C – O ~ P Carbamoyl phosphate NH2 – R Ornithine Ornithine transcarbamoylase Pi O Citrulline H2N – C – NH – R Mitochondria Ornithine-Citrulline Antiporter Mitochondrial Membrane Cytosol ATP CH2-COOH Arginino α-KG H2NCH-COOH succinate AST synthetase Glu PLP Aspartate AMP + PPi Oxaloacetate NH CH2-COOH + NADH,H R – HN – C – HN – CH-COOH Malate dehydrogenase Argininosuccinate + NAD Argininosuccinase L-Malate (Argininosuccinate lyase) HC-COOH NH Fumarase HOOC-CH H2N – C – NH – R Fumarate H2O Arginine H2O Arginase NH2 – R Ornithine O H2N – C – NH2 Urea The different steps include 1-Free ammonium ion and bicarbonate (CO2) are condensed, at the expense of 2 ATP, to form carbamoyl phosphate. The reaction is catalyzed by carbamoyl phosphate synthetase I (CPSI), which occurs in the mitochondrial matrix, and uses ammonia as a nitrogen donor. It is absolutely dependent on N-acetylglutamate for its activity. [N.B. Carbamoyl phosphate synthetase II (CPSII): It is another enzyme with similar activity. It is a cytosolic enzyme and uses the amide group of glutamine for pyrimidine synthesis, and not affected by N-acetylglutamate]. 2- Formation of citrulline is catalyzed by ornithine transcarbamoylase in the mitochondrial matrix. Citrulline is transported out of the mitochondria to the cytosol (in exchange with ornithine), where the other reactions of the urea cycle occur. 3- Formation of argininosuccinate is catalyzed by argininosuccinate synthetase, which requires hydrolysis of ATP to AMP and PPi (the equivalent of hydrolysis of two molecules of ATP). 4- Cleavage of argininosuccinate by argininosuccinate lyase produces fumarate and arginine. 5- Arginine is cleaved by arginase to ornithine and urea. Ornithine returns back to the mitochondrial matrix for another turn of the cycle. Regulation of Urea Cycle 1- N-acetylglutamate is an essential activator for carbamoyl phosphate synthetase rate-limiting step in the urea cycle. N-Acetylglutamate is synthesized from acetyl CoA and glutamate, in a reaction for which arginine is an activator. Therefore, the intrahepatic concentration of N- acetylglutamate increases after ingestion of a protein-rich meal, which provides both the substrate (glutamate) and the regulator of N-acetylglutamate synthesis. This leads to an increased rate of urea synthesis Regulation of Activity of CPSI Arginine CO2 + NH3 2 ATP + CoA Glutamate + Acetyl-CoA N-Acetylglutamate + CPSI N-Acetyglutamate synthase 2 ADP + Pi O Carbamoyl phosphate NH2 – C – O ~ P 2- Factors affecting levels of urea cycle enzymes: - High protein diet: major changes in diet can increase the concentration of individual urea cycle enzymes 10 to 20 folds. - Administration of the protein catabolic hormones increases the concentration of urea cycle enzymes. - Starvation elevates urea cycle enzyme levels to cope with the increased production of ammonia that accompanies enhanced degradation of proteins. Hyperammonemia The urea formed by the liver goes, via the blood (plasma level 10-50 mg/dL), to the kidneys to be excreted in the urine. In renal failure, the plasma level of urea increases. In hepatic failure, ammonia remains in the blood, leading to hyperammonemia (ammonia intoxication) with decrease of plasma urea level. The capacity of the hepatic urea cycle exceeds the normal rates of ammonia generation, and the levels of blood ammonia are normally low (