General Biology PDF
Document Details
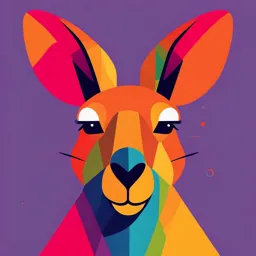
Uploaded by AmazedElder
Paclian, Mark Lord R.
Tags
Summary
This textbook covers General Biology, from cell structure and the cell theory to the different types of cells and their functions.
Full Transcript
The Discovery of Cell and the Development of the Modern Cell Theory Cell walls were first seen by Robert Hooke in 1665 as he looked through a microscope at dead cells from the bark of an oak tree. Antoni van Leeuwenhoek using his wonderfully crafted lenses and was able to vi...
The Discovery of Cell and the Development of the Modern Cell Theory Cell walls were first seen by Robert Hooke in 1665 as he looked through a microscope at dead cells from the bark of an oak tree. Antoni van Leeuwenhoek using his wonderfully crafted lenses and was able to visualize living cells, whom he called “very little animalcules” and showed to Hooke in 1674. Robert Brown in 1831 concluded that nucleus is a fundamental component of cells. Matthias Schleiden (1838) found that all parts of plants was composed of cells. Theodore Schwann in 1839 proved that cells were the basic unit of animal tissues. Rudolf Virchow (1858) proved that all cells come from free existing cell. The Modern Cell Theory states that: Every living organism is made up of one or more cells. The smallest living organisms are single cells, and the cells are the fundamental units of multicellular organisms. All cells arise from pre-existing cells. Cells are fundamental to all living organisms. All cells are related by their descent from earlier cells. During the long evolutionary history of life on Earth, cells have been modified in many ways. But although cells can differ substantially from one another, they share common features. Bacterial Cell Human Cell Plant Cell Animal Cell Cell as the basic organizational unit of all living things is observable in both unicellular and multicellular organisms. Unicellular Organism Multicellular Organism The Nucleus contains most of the cell’s genes and is usually the most visible organelle. The nuclear envelope encloses the nucleus, separating it from the cytoplasm. The nuclear membrane is a double membrane; each membrane consists of a lipid bilayer. And inside nucleus is the nucleolus, the site where the ribosome is synthesized. Ribosomes are complexes made of ribosomal RNAs and proteins. These are the cellular components that carry out protein synthesis, to make new parts and reproduce itself. Endoplasmic Reticulum (ER) is an extensive network of membranes that accounts for more than half the total membrane in many eukaryotic cells. (Endoplasmic means “within the cytoplasm,” and reticulum is Latin for “little net.”) There are two distinct, though connected, regions of the ER that differ in structure and function: smooth ER and rough ER Smooth ER lacks ribosomes in its outer surface. Its functions include synthesis of lipids, metabolism of carbohydrates, detoxification of drugs and poisons, and storage of calcium ions. is studded with ribosomes on the outer surface of the membrane and thus appears rough through the electron microscope. It makes secretory proteins and serves as a membrane factory for the cell; it grows in place by adding membrane proteins and phospholipids to its own membrane. Golgi Apparatus consists of a group of associated, flattened membranous sacs cisternae— looking like a stack of pita bread. It is here that the products of the ER, such as proteins, are modified and stored and then sent to other destinations. Lysosomes are membranous sacs of hydrolytic enzymes that many eukaryotic cells use to digest (hydrolyze) macromolecules. Lysosomes contain cellular digestive enzymes used in recycling parts and breaking of food into particles that the cell uses to grow Vacuoles are large vesicles derived from the endoplasmic reticulum and Golgi apparatus. Thus, vacuoles are an integral part of a cell’s endomembrane system. Like all cellular membranes, the vacuolar membrane is selective in transporting solutes; as a result, the solution inside a vacuole differs in composition from the cytosol. 1. Central vacuoles in mature plants contain a solution called cell sap. It is the plant cell’s main repository of inorganic ions, including potassium and chloride. The central vacuole plays a major role in the growth of plant cells, which enlarge as the vacuole absorbs water, enabling the cell to become larger with a minimal investment in new cytoplasm. 2. Contractile vacuoles in unicellular eukaryotes pump excess water out of the cell, thereby maintaining a suitable concentration of ions and molecules inside the cell. 3. Food vacuoles- fuse with lysosomes 4. Other vacuoles can hold reserves of important organic compounds, such as the proteins stockpiled in the storage cells in seeds. a. May also store compounds that are poisonous or unpalatable to herbivores. b. Some plant vacuoles contain pigments, such as the red and blue pigments of petals that help attract pollinating insects to flowers. Mitochondria (singular, mitochondrion) are the sites of cellular respiration, the metabolic processthat uses oxygen to drive the generation of ATP by extracting energy from sugars, fats, and other fuels Chloroplasts are found in plants and algae and are the sites of photosynthesis The Evolutionary Origins of Mitochondria and Chloroplasts The Endosymbiont Theory, states that an early ancestor of eukaryotic cells engulfed an oxygen-using non photosynthetic prokaryotic cell. Eventually, the engulfed cell formed a relationship with the host cell in which it was enclosed, becoming an endosymbiont (a cell living within another cell). Indeed, over the course of evolution, the host cell and its endosymbiont merged into a single organism, a eukaryotic cell with a mitochondrion. At least one of these cells may have then taken up a photosynthetic prokaryote, becoming the ancestor of eukaryotic cells that contain chloroplasts Peroxisomes are specialized metabolic compartments bounded by a single membrane. These are roughly spherical and often have a granular or crystalline core that contain enzymes that remove hydrogen atoms from various substrates and transfer them to oxygen (O2), producing hydrogen peroxide (H2O2) as a by-product (from which the organelle derives its name) Cytoskeleton gives shape and support to cells, especially to animal cells. It also positions and moves cell parts. Centrosome synthesizes microtubules of cilia and flagella. In animals, it produces spindle. Centrioles (pair) are found within the centrosome. Each is composed of nine sets of triplet microtubules arranged in a ring. Flagella and Cilia move the cell through fluid or move fluid past the cell surface. Cell membrane or plasma membrane, surrounds the cell and serves as the boundary that separates a living cell from its surroundings and controls all inbound and outbound traffic Cell wall is an extracellular structure of plant cells. It protects the plant cell, maintains its shape, and prevents excessive uptake of water. The Endomembrane System. The endomembrane system is a complex and dynamic player in the cell’s compartmental organization. An organelle is a cellular structure that performs a specific function. Cell structures can be classified into membrane bound organelles and non-membrane bound organelles. Non-Membrane-bound Membrane-bound Organelles Organelles Nucleus Mitochondria Golgi Apparatus Ribosomes Smooth ER Lysosomes Vacuoles and Vesicles Centrioles Rough ER Peroxisomes Chloroplast and other plastids Cytoskeleton Advantages of membrane-bound organelles. The compartmentalization of the cell into membrane-bound organelles: Allows conflicting functions (i.e., synthesis vs. breakdown) and several cellular activities to occur simultaneously without interference from each other. Separates the DNA material of the nucleus, mitochondria, and chloroplast. Increases the surface area-volume ratio of the cell. Prokaryotic Cell VS Eukaryotic Cell Prokaryotic Cells Prokaryote came from Greek words pro meaning before, and karyo which means kernel (pertaining to the nucleus). Prokaryotic cells lack true nucleus and other membrane-enclosed organelles. Eukaryotic Cells Eukaryote came from the Greek words eu which means true, and karyo which means kernel, pertaining to the nucleus. Eukaryotic cells have true nucleus, and internal membranes that compartmentalize cellular functions. Prokaryotes vs Eukaryotes Prokaryotes Prokaryotes are organisms that lack true nucleus and are all unicellular. Example: E. coli, pyrolobus fumarii, salmonella Eukaryotes Eukaryotes are organisms with true nucleus and are usually multicellular organisms, but some are unicellular organisms. Example: dog, narra, earthworm The term tissue is used to describe a group of cells found together in the body. The cells within a tissue share a common embryonic origin. Microscopic observation reveals that the cells in a tissue share morphological features and are arranged in an orderly pattern that achieves the tissue’s functions. From the evolutionary perspective, tissues appear in more complex organisms. For example, multicellular protists, ancient eukaryotes, do not have cells organized into tissues. Although there are many types of cells in the human body, they are organized into four broad categories of tissues: epithelial, connective, muscle, and nervous 1. Epithelial Tissue Epithelial (epi, on + thele, covering or lining) tissue is found throughout the body as covering of both internal and external surfaces. Skin on the outside of the body and linings of cavities such as the digestive tract, respiratory passages, and blood vessels. It is characterized by closely joined cells with tight junctions (i.e., a type of cell modification). The cells that make up epithelial tissues have distinct shapes and arrangements. Its classification is according to its shape and arrangement. Simple -one layer Stratified- more than one layer. Pseudostratified -it gives a wrong impression that there is more than one layer of cells but is one layer. 2. Connective Tissue Connective tissue functions: 1. Enclosing and separating. Sheets of connective tissues form capsules around organs, and layers that separate tissues and organs. 2. Connecting tissues to one another. 3. Supporting and moving. 4. Storing. 5. Cushioning and insulating. 6. Transporting. 7. Protecting. A. BLOOD Location: within blood vessels and heart. Produced by the red bone marrow. Structure: Blood cells are suspended in a fluid called plasma containing water, salts, and dissolved proteins. o erythrocytes that carry oxygen (RBC), o leukocytes for defense (WBC), and o platelets for blood clotting. Function: It transports oxygen, carbon dioxide, hormones, nutrients, wastes products and other substances. Also protects the body from infection and is involved in temperature regulation. B. CONNECTIVE TISSUE PROPER (CTP) Connective tissue proper can be classified into loose connective tissue and dense connective tissue. Loose Connective Tissue Location: Widely distributed throughout the body, it is the substance on which most epithelial tissue rests, it is the packing between glands, muscles, and nerves, it attaches the skin to underlying tissues, and forms the superficial layer of the dermis. Structure: Cells (fibroblast, macrophages, and lymphocytes) within a fine network of mostly collagen fibers, the cells and fibers are separated from one another by fluid filled spaces. Function: Loose packing and supporting for the structures with which it is associated. Adipose tissue is also an example of loose connective tissues that store fats which function to insulate the body and store energy Dense Connective Tissue Has an extracellular matrix that consists of densely packed fibers. Location: Tendons (attached muscle to bone), nonelastic ligaments (attach bone to bone), most of the dermis of the skin, and organ capsules. Structure: Matrix consists almost entirely of collagen fibers produced by fibroblast; the fibers can all be oriented in the same direction (tendons and ligaments), or in many different directions (dermis and organ capsules). Function: Able to withstand great pulling forces and resists stretching in the direction of fiber orientation C. Cartilage Are of three types: 1. Hyaline Cartilage Location: ribs, cartilage ring of respiratory tract, and in nasal cartilages; covers the end of the bones, growth plates of bones, and embryonic skeleton. Structure: Solid matrix and evenly distributed collagen→ transparent Function: Support and flexibility, forms smooth surface of joint 2. Fibrocartilage Location: found in intervertebral disks, symphysis pubis, and articulating cartilage of some joints (knee and jaw) Structure: collagen fiber is more numerous than in hyaline and elastic cartilage, and they are arranged in thick bundles. Function: Flexible and capable of withstanding considerable pressure. Connects structures subjected to great pressure. 3. Elastic Cartilage Location: external ears, epiglottis, and auditory tubes Structure: similar to hyaline cartilage but the matrix has abundant elastic fiber Function: provide rigidity but more flexible than hyaline cartilage because elastic fibers return to their original shape after being stretched. D. BONE Bone is a hard connective tissue that consists of living cells and mineralized matrix. The matrix of collagen is combined with calcium, magnesium, and phosphate ions to make the bone hard. Blood vessels and nerves are found at a central canal surrounded by concentric circles of osteons Location: all the bones of the body Structure: hard, mineralized matrix, many osteocytes (bone cells) are located within lacunae, and matrix is organized into layers called lamellae. Function: provides great strength and support and protects internal organs. Provides attachment sites to muscles and ligaments. The joints of bones allow movements. 3. Muscle Tissue The main characteristic of muscle tissue is its ability to contract, or shorten, making movement possible. It is composed of long cells called muscle fibers that allow the body to move voluntarily or involuntary as a response to signals coming from nerve cells. In vertebrates, these muscles can be categorized into the following: 1. Skeletal muscle o Location: attaches to bone o Structure: striated, cell is large, long and cylindrical with many nuclei located at the periphery. o Function: voluntary movements of the body 2. Smooth muscle o Location: walls of hollow organs and tubes o Structure: tapered at each end, not striated, and have a single nucleus o Function: regulates the size of organs, forces fluids through tubes, controls the light entering the eye; under involuntary control 3. Cardiac muscle o Location: heart o Structure: striated with a single, centrally located nucleus, they are branched and connected to one another by intercalated disk. o Function: pumps the blood and is under involuntary control 4. Nervous Tissue Nervous tissue forms the brain, spinal cord, and nerves. It is responsible for coordinating and controlling many bodily activities. Nerve tissue consists of neurons (nerve cells) and neuroglia (glial cells) that serves as support cells. Neuron is responsible for conducting action potential. It is composed of the cell body (site for general cell function), dendrite (receives action potential) and axon (conducts action potential away from cell body). The cell is the fundamental unit of life. For it to cater to its other tasks and functions the cell has to undergo modification. Cell specialization (or modification or differentiation) is a process that occurs after cell division where the newly formed cells are structurally modified so that they can perform their function efficiently and effectively. Three Types of Cell Modifications: 1. Apical modification- occurs at the top part of the cell. The main function of apical modification is for absorption, locomotion, and secretion. Example of Apical Modification in cell A. Pseudopods- known as false-feet. - It enables the organism to make temporary and irregular lobes. - formed by amoebas and some other eukaryotic cells. - It is used for movement and engulfs prey. B. Cilia and Flagella - cilia are short hair-like structure attached to the surface of the cell and have a wave-like motion. - flagella are a whip-like modification and it’s primarily for locomotion. - formed from microtubules C. Villi and Microvilli - These are finger-like projections that arise from the epithelial layer in some organs. - They help to increase surface area for faster and more efficient absorption. Microvilli are smaller projections - They arise from the cell’s surface that also increase surface area allowing faster and more efficient absorption D. Extra-Cellular Matrix (ECM) - It is a compound secreted by the cell on its apical surface - Cell wall is the extra-cellular structure in plant cells that distinguishes them from animal cells - Glycoprotein is the main ingredient of ECM in animal cells 2. Lateral modification- happens at the side of the cell. The main function of lateral modification is it serves as the intercellular connection between two adjacent cells. Example of Lateral Modification in cell A. Gap junction- It is also known as communicating junctions - it allows direct flow of molecules between cells and connects two cells directly from the plasma. B. Tight Junction- it held two adjacent cells tightly - prevents leakage of materials between cells and acts as a water seal. - regulate the movement of water and solutes between epithelial layers C. Adhering Junction- It anchors junction on the lateral surface of the cell - It is similar to the anchoring junction of the basal surface of the cell 3. Basal modification- which occurs at the bottom part of the cell. A. Desmosomes/Hemidesmosomes - The anchoring junction on the Basal surface of the cell - Rivet-like links between cytoskeleton and Extracellular matrix components such as the basal lamina that underlie epithelia - Primarily composed of keratin, integrins and cadherins - Other specialized modifications include the development of nerve cells, muscle cells, red blood cells and sperm cells. In plants, some identified specialized cells include the root hairs and the guard cells. Specialized Animal Cell Animal cells are specialized in many ways. Red blood cells are specialized in transporting oxygen. Cells specialized in producing proteins are found in the pancreas. Pancreatic cells also possess a large number of other organelles needed for protein export, including a well-developed Golgi apparatus and clusters of storage vacuoles loaded with enzymes. The human ability to move is the result of the specialized structure of muscle cells. Skeletal muscle cells are packed with fibers arranged in a tight, regular pattern. Specialized Plant Cell The guard cell is an example of a specialized plant cell. It monitors the plant's internal condition, changing its shape according to those conditions. Cell division is the process of cell reproduction where identical genetic material is passed-on to two cellular offspring. The cell division process is an integral part of the cell cycle. Cell cycle is the life of a cell from the time it is first formed during division of a parent cell until its own division into two daughter cells. (Note: Biologists use the words daughter or sister in relation to cells, but this is not meant to imply gender.) Phases of Cell Cycle) 1. INTERPHASE 90% of the cycle The growth periods. 3 Sub phases of Interphase G1 phase or Gap 1 - cell growth Cell grows by producing proteins and cytoplasmic organelles such as mitochondria and endoplasmic reticulum. S phase or Synthesis phase - duplication of chromosomes G2 phase or Gap 2 - the cell grows more in preparation for the cell division 2. M Phase In the M phase, mitosis distributes the daughter chromosomes to daughter nuclei, and cytokinesis divides the cytoplasm, producing two daughter cells. Cell Cycle Control System It is a cyclically operating set of molecules in the cell that both triggers and coordinates key events in the cell cycle. The cell cycle is regulated at certain checkpoints by both internal and external signals. A checkpoint in the cell cycle is a control point where stop and go-ahead signals can regulate the cycle. Animal cells generally have built-in stop signals that halt the cell cycle at checkpoints until overridden by go-ahead signals. The three important checkpoints are found in the G1, G2, and M phases. If a cell receives a go-ahead signal at the G1 checkpoint, it will usually complete the G1, S, G2, and M phases and divide. If it does notreceive a go-ahead signal atthat point, it may exitthe cycle,switching into a nondividing state called the G0 phase. Most cells of the human body are in the G0 phase. Example, mature nerve cells and muscle cells never divide. Other cells, such as liver cells, can be “called back” from the G0 phase to the cell cycle by external cues, such as growth factors released during injury. In 1882, a German anatomist named Walther Flemming developed dyes that allowed him to observe, for the first time, the behavior of chromosomes during mitosis and cytokinesis. Mitosis is the process of cellular reproduction that occurs in the nucleus and forms two identical nuclei. It is conventionally broken down into five stages: prophase, prometaphase, metaphase, anaphase, and telophase. Overlapping with the last stage of mitosis, cytokinesis completes the mitotic phase (M phase). From G2 of interphase, the following can be observed: The nuclear envelope encloses the nucleus. The nucleus contains one or more nucleoli (singular, nucleolus). Two centrosomes have formed by duplication of a single centrosome. (Centrosomes are regions in animal cells that organize the microtubules of the spindle. Each centrosome contains two centrioles). Chromosomes, duplicated during S phase, cannot be seen individually because they have not yet condensed. The Stages of Mitosis follows: 1. Prophase In prophase, the centrioles move toward the opposite sides of the nucleus and the nuclear envelope starts to disappear. The chromatin fibers become more tightly coiled, condensing into discrete chromosomes (observable with a light microscope). The nucleoli disappear. Each duplicated chromosome appears as two identical sister chromatids joined at their centromeres. The mitotic spindle begins to form. It is composed of the centrosomes and the microtubules that extend from them. The radial arrays of shorter microtubules that extend from the centrosomes are called asters (“stars”). The centrosomes move away from each other, propelled partly by the lengthening micro- tubules between them. Between prophase and metaphase is what we call the prometaphase It is when the nuclear envelope fragments. The microtubules extending from each centrosome can now invade the nuclear area. The chromosomes have become even more condensed. A kinetochore has now formed at the centromere of each chromatid (thus, two per chromosome). Some of the microtubules attach to the kinetochores, becoming “kinetochore microtubules,” which jerk the chromosomes back and forth. No kinetochore microtubules interact with those from the opposite pole of the spindle, lengthening the cell. 2. Metaphase The centrosomes are now at opposite poles. The chromosomes have all arrived at the metaphase plate, a plane that is equidistant between the spindle’s two poles. The chromosomes’ centromeres lie at the metaphase plate. The kinetochores of the sister chromatids are attached to kinetochore microtubules coming from opposite poles. 3. Anaphase Anaphase is initiated by the separation of sister chromatids at their junction point at the centromere. The daughter chromosomes then move toward the poles. It is the shortest stage of mitosis, often lasting only a few minutes, that begins when the cohesion proteins are cleaved. This allows the two sister chromatids of each pair to part suddenly. Each chromatid thus becomes an independent chromosome. The two new daughter chromosomes begin moving toward opposite ends of the cell as their kinetochore microtubules shorten. Because these microtubules are attached at the centromere region, the centromeres are pulled ahead of the arms, moving at a rate of about 1 µm/min. The cell elongates as the no kinetochore microtubules lengthen. By the end of anaphase, the two ends of the cell have equivalent—and complete— collections of chromosomes. 4. Telophase During telophase, the two daughter nuclei form in the cell. Nuclear envelopes arise from the fragments of the parent cell’s nuclear envelope and other portions of the endomembrane system. Nucleoli reappear. The chromosomes become less condensed. Any remaining spindle microtubules are depolymerized. The division of one nucleus into two genetically identical nuclei is now complete Cytokinesis In animal cells, cytokinesis involves the formation of a cleavage furrow, which pinches the cell in two. The first sign of cleavage is the appearance of a cleavage furrow, a shallow groove in the cell surface near the old metaphase plate. The cleavage furrow deepens until the parent cell is pinched in two, producing two completely separated cells, each with its own nucleus and its own share of cytosol, organelles, and other subcellular structures. Cytokinesis in plant cells, which have cell walls, is marked by a cell plate. During telophase, vesicles derived from the Golgi apparatus move along microtubules to the middle of the cell, where they merge to produce a cell plate. Cell wall materials carried in the vesicles collect inside the cell plate as it grows. The cell plate enlarges until its surrounding membrane fuses with the plasma membrane along the perimeter of the cell. Two daughter cells result, each with its own plasma membrane and a new cell wall arises from the contents of the cell plate. Binary Fission in Bacteria The term binary fission, meaning “division in half,” refers to a process in which the cell grows to roughly double its size and then divides to form two cells. It is the reproduction type to prokaryotes (bacteria and archaea) and to the asexual reproduction of single-celled eukaryotes, such as the amoeba. However, the process in eukaryotes involves mitosis, while that in prokaryotes does not. Chiasmata (singular, chiasma) the x-shaped regions in each homologous chromosome where crossovers occur. Kinetochore the site in the centromere where the spindle microtubule attachments Meiosis is a reduction division of the nuclear materials that each gamete contains only half as much hereditary material as the parent cell. The process involves two successive divisions of a diploid nucleus. In meiosis, chromosomes duplication in interphase, followed by two consecutive cell divisions, called meiosis I and meiosis II. These two divisions result in four daughter cells, each with only half as many chromosomes as the parent cell. Exploring Meiosis in an Animal Cell Meiosis I: The first meiotic division results in reducing the number of chromosomes (reduction division). In this process, homologous chromosomes are separated. In most cases, the division is accompanied by cytokinesis. Prophase I Centrosome movement,spindle formation, and nuclear envelope breakdown occur. Chromosomes condense progressively throughout prophase I. Duplicated homologous chromosomes (red and blue) pair up and exchange segments. During early prophase I, each chromosome pairs with its homolog, aligned gene by gene, and crossing over occurs at chiasmata: The DNA molecules of nonsister chromatids are broken (by proteins) and are rejoined to each other. Later in prophase I, microtubules from one pole or the other attach to the kinetochores, one at the centromere of each homolog. (The two kinetochores on the sister chromatids of a homolog are linked together by proteins and act as a single kinetochore.) Microtubules move the homologous pairs toward the metaphase plate (see the metaphase I diagram). Metaphase I Chromosomes line up by homologous pairs. o Pairs of homologous chromosomes are now arranged at the metaphase plate, with one chromosome of each pair facing each pole. o Both chromatids of one homolog are attached to kinetochore microtubules from one pole; the chromatids of the other homolog are attached to microtubules from the opposite pole. Anaphase I The two homologous chromosomes of each pair separate. o Breakdown of proteins that are responsible for sister chromatid cohesion along chromatid arms allows homologs to separate. o The homologs move toward opposite poles, guided by the spindle apparatus. o Sister chromatid cohesion persists at the centromere, causing chromatids to move as a unit toward the same pole. Telophase I Two haploid cells form; each chromosome still consists of two sister chromatids. When telophase I begins, each half of the cell has a complete haploid set of duplicated chromosomes. Each chromosome is composed of two sister chromatids; one or both chromatids include regions of non-sister chromatid DNA. Cytokinesis usually occurs simultaneously with telophase I, forming two haploid daughter cells. (In animal cells a cleavage furrow form. In plant cells, a cell plate forms.) In some species, chromosomes decondense and nuclear envelopes form. No chromosome duplication occurs between meiosis I and meiosis II. Meiosis II: The events in the second meiotic division are quite like a mitotic division. The difference lies, however, in the number of chromosomes that each daughter cell receives. While the original chromosome number is maintained in mitosis, the number is reduced to half in meiosis. During the second round of cell division, the sister chromatidsfinally separate, and four haploid daughter cells result, containing unduplicated chromosomes. Prophase II The spindle fiber starts to form at the opposite poles of the cell. In late prophase II, chromosomes, each still composed of two chromatids associated at the centromere, are moved by microtubules toward the metaphase II plate. Metaphase II The chromosomes are positioned at the metaphase plate as in mitosis. Because of crossing over in meiosis I, the two sister chromatids of each chromosome are not genetically identical. The kinetochores of sister chromatids are attached to microtubules extending from opposite poles. Anaphase II Breakdown of proteins holding the sister chromatids together at the centromere allows the chromatids to separate. The chromatids move toward opposite poles as individual chromosomes. Telophase II Nuclei form, the chromosomes begin decondensing, and cytokinesis occurs. The meiotic division of one parent cell produces four daughter cells, each with a haploid set of (unduplicated) chromosomes. The four daughter cells are genetically distinct from one another and from the parent cell. Significance of Mitosis for Sexual Reproduction Mitosis is important for sexual reproduction indirectly. It allows the sexually reproducing organism to grow and develop from a single cell into a sexually mature individual. This allows the organism to continue to produce through the generations. Significance of Meiosis and Chromosome Number Chromosomes are a cell's way to neatly arrange long strands of DNA. Non-sex cells have two sets of chromosomes, one set from each parent. Meiosis has sex cells with only one set of chromosomes Significance of Meiosis for Diversity One of the benefits of sexual reproduction is the diversity it produces within a population. That variety is a direct product of meiosis. Every sex cell made from meiosis has a unique combination of chromosomes. This indicates that no two sperm or egg cells have the exact same genetic makeup. New trait combinations are created with every fertilization event. This is why siblings share DNA with parents and each other but are not identical to one another. Significance of mitosis for sexual reproduction The process of mitosis indirectly affects sexual reproduction. It enables the sexually reproducing organism to grow and mature from a single cell into an adult. By doing so, organisms can keep reproducing for a long time. Defective cell cycle and cell death regulation in disease the cell cycle is the main process leading to cellular proliferation. However, there is a close link between cell proliferation and cell death. It seems likely that when a cell establishes the programmed to proliferate, it also acquires an abort pathway better dead than wrong. However, cancer is not the only disorder associated with defective cell cycle/cell death regulation. During the past several decades, many examples of a link between aberrant regulation of the cell cycle and/or cell death and the onset of disease has emerged. Many disorders can now be attributed, directly or indirectly, to defective mechanisms of regulation of these important biological processes DISEASES AND DISORDERS Cell Division in Cancer Cells o Cancer cells can divide without appropriate external signals. o Cancer cells do not exhibit contact inhibition. o Cancer cells continue dividing in the presence of genetic damage. o The uninhibited, continued division of genetically damaged cells can lead to tumor formation. Down Syndrome o Down syndrome is a genetic disorder caused when abnormal cell division results in an extra full or partial copy of chromosome 21. o This extra genetic material causes the developmental changes and physical features of Down syndrome. Alzheimer's disease o Alzheimer's disease is thought to be caused by the abnormal build-up of proteins in and around brain cells. One of the proteins involved is called amyloid, deposits of which form plaques around brain cells. The other protein is called tau, deposits of which form tangles within brain cells. As brain cells become affected, there's also a decrease in chemical messengers (called neurotransmitters) involved in sending messages, or signals, between brain cells. Levels of one neurotransmitter, acetylcholine, are particularly low in the brains of people with Alzheimer's disease. Over time, different areas of the brain shrink. The first areas usually affected are responsible for memories. Plasma Membrane Plasma membrane surrounds the cell and serves as the boundary, separating a living cell from its surroundings, and controls all inbound and outbound traffic. It is selectively permeable, allowing some substances to cross it more easily than others. Plasma membrane is primarily composed of phospholipid bilayer. It also has proteins, lipids, and some carbohydrates The ability of phospholipids to form membranes is inherent in their molecular structure. Phospholipids are amphipathic molecules meaning, molecules containing hydrophobic and hydrophilic regions. Because of this, phospholipid bilayer can exist as a stable boundary between two aqueous compartments. As a Fluid Mosaic Model The membrane looks like a mosaic of protein molecules bobbing in a fluid bilayer of phospholipids, that is why it is described as a fluid mosaic model. Proteins reside in the phospholipid bilayer with their hydrophilic regions protruding, which maximizes contact with water in the cytosol and extracellular fluid, while providing their hydrophobic parts with a nonaqueous environment. Membrane Proteins and Their Functions 6 Major Functions of Membrane proteins 1. Transport 2. Enzymatic activity 3. Signal transduction 4. Cell-cell recognition 5. Intercellular joining 6. Attachment to the cytoskeleton and extracellular matrix (ECM) 2 Types of Protein Membrane 1. Integral proteins penetrate the hydrophobic interior of the lipid bilayer. Transmembrane proteins span through the membrane. 2. Peripheral proteins are loosely bound to the surface of the membrane Carbohydrates in Membrane for Cell-Cell Recognition Cells recognize each other by binding to surface molecules, often containing carbohydrates on the extracellular surface of the plasma membrane Carbohydrates in the membrane may be covalently bonded to lipids (forming glycolipids) or, more commonly, to proteins (forming glycoproteins). Molecules and substances move in several ways that fall within two categories: passive transport and active transport. In passive transport, the heat energy of the cellular environment provides all the energy; hence, this is not energy-costly to the cell. Diffusion is a type of passive transport described as the natural tendency for molecules to move constantly. Substances diffuse down their concentration gradient, from where it is more concentrated to where it is less concentrated. Whether one or more solutes are involved, diffusion or movement of solute will always be down its concentration gradient. Net diffusion occurs when the materials on one side of the membrane have a different concentration than materials on the other side. This leads to a dynamic equilibrium: The solute molecules continue to cross the membrane, but at roughly equal rates in both directions. Osmosis is a special type of diffusion specifically associated with the movement of water molecules. Water diffuses across a membrane from the region of lowersolute concentration to the region of higher solute concentration until the solute concentration is equal on both sides The behavior of a cell in a solution is influenced by both solute concentration and membrane permeability. Both factors are considered in the concept of tonicity. Tonicity is the ability of a surrounding solution to cause a cell to gain or lose water. The tonicity of a solution depends in part on its concentration of solutes that cannot cross the membrane (nonpenetrating solutes) relative to that inside the cell. Isotonic solution: The solute concentration of the solution is the same as inside the cell, and so, no net water movement across the plasma membrane. Hypertonic solution: The solute concentration of the solution is greater than that inside the cell; thus, the cell loses water. Hypotonic solution: The solute concentration of the solution is less than that inside the cell, so, the cell gains water. Water Balance of Cells with Cell Walls In plants, cell walls help maintain water balance. o A plant cell in a hypotonic solution swell until the wall opposes water uptake called turgor pressure, and the cell becomes turgid (very firm), the healthy state for most plant cells. o If a plant cell and its surroundings are isotonic, there is no net movement of water into the cell, so the cell becomes flaccid (limp), and the plant may wilt. o In a hypertonic environment, the plant cell loses water; eventually, the membrane pulls away from the wall, resulting in a usually lethal effect called plasmolysis (wilt). Facilitated Diffusion Facilitated diffusion works when polar molecules and ions hindered by the lipid bilayer of the membrane diffuse passively with the help of transport proteins that span the membrane Aquaporins are channels that transport water. They are always open and facilitate the massive levels of diffusion of water (osmosis) that occur in plant cells and in animal cells such as red blood cells. Channel proteins provide passage that allow specific molecules or ions to cross the membrane. They work as gated channels through the membrane that opens and closes in response to ligand binding, mechanical deformation, or voltage. Collectively, channel proteins can transport polar, bulky, or charged molecules that cannot diffuse through the membrane down the electrochemical gradient. Subtypes of channel proteins are voltage-gated, ligand-gated, and mechanically gated. Carrier proteins are proteins that bind the solute on one side of the membrane and undergo a conformational change to expose it to the other side membrane. One of the subtypes of carrier proteins uniporter, which transports one type of solute in one direction. Active transport uses energy to move solutes against the concentration gradients or electrochemical gradient. Active transport enables a cell to maintain internal concentrations of small solutes that differ from concentration in its environment. Active transport can be a primary active transport or a secondary active transport. Primary Active Transport In primary active transport, pump transport solutes up the concentration gradient or an electrochemical gradient by direct use of ATP or light. Transport proteins involved for this are ATP-driven pumps like the sodium-potassium pump, and light-driven pumps in plants. The sodium-potassium pump directly uses ATP to transport Na and K. Here is how it works Secondary Active Transport Secondary active transport involves active transport of one substance, such as an ion, across the cell membrane, establishing a concentration gradient. Then the diffusion of transported substance down its concentration gradient provides the energy to transport a different substance. Note: The H+/glucose transport is an example of secondary active transport, and of cotransport/symport as well. Based on movement of materials, active transport can be a cotransport (through a symporter) or a counter transport (through an antiporter). Symporter-transport protein that transports multiple cargo types in opposite directions. Antiporter-transport protein that transports multiple solute-types in opposite directions. Cotransport/Symport: Coupled Transport by a Membrane Protein A solute that exists in different concentrations across a membrane can do work as it moves across that membrane by diffusion down its concentration gradient. Here the diffusing substance moves in the same direction as the transported substance A carrier protein, such as this H+ /sucrose cotransporter in a plant cell, can use the diffusion of H+ down its electrochemical gradient into the cell to drive the uptake of sucrose. Although not technically part of the cotransport process, an ATP-driven proton pump concentrates H+ outside the cell, resulting to H+ gradient and potential energy that can be used for active transport—of sucrose, in this case. Thus, ATP hydrolysis indirectly provides the energy necessary for cotransport. Counter transport It is the movement of the diffusing substance in the opposite direction as the transported substance. An example of this is the movement of sodium and potassium through the sodium-potassium pump. Bulk Transport The transport of large molecules,such as polysaccharides and proteins, acrossthe membrane changesthe shape of the membrane which requires energy. Transport may occur through exocytosis or endocytosis. Endocytosis Is when the cell takes in molecules and particulate matter by forming new vesicles from the plasma membrane. It is the reverse of exocytosis, involving different proteins. There are 3 types of endocytosis Phagocytosis (“cellular eating”), the transported substance is solid. Pinocytosis (“cellular drinking”), the transported substance is liquid. Receptor-mediated endocytosis, the specialized type of pinocytosis that enables the cell to acquire bulk quantities of specific substances, even though those substances may not be very concentrated in the extracellular fluid. Exocytosis is the exact opposite of endocytosis. Here the transport vesicles carrying the substance migrate to the membrane, fuse with it, and release the contents. Catalyst- A substance that speeds up a chemical reaction without being changed Enzyme- A biological catalyst (usually a protein) Substrate- The reactant molecule that an enzyme works on Active Site- The part of the enzyme where the substrate binds Enzyme-substrate complex formed when the substrate molecule collides with the active site of its enzyme Endoenzymes(intracellular) / Exoenzymes (extracellular) Activation energy- the minimum energy required to start a chemical reaction. Transition state- the intermediate stage in a reaction in which the old bonds break and new bonds are formed Components of an Enzyme Enzyme- protein macromolecules. They have a defined amino acid sequence and are typically 100-500 amino acids long. They have a defined three-dimensional structure. They act as a catalyst to a chemical or biochemical reaction, with a defined mechanism. They increase the speed of that reaction, typically by 106-1014 times faster than the rate of the uncatalyzed reaction. They are selective for a single substrate. They speed up the rate of reaction by lowering the activation energy (Ea). They are stereospecific, meaning the reaction produces a single product. Enzymes are "specific." Each type of enzyme typically only reacts with one (Fig 8.b.), or a couple, of substrates. Some enzymes are more specific than others and will only accept one particular substrate. Other enzymes can act on a range of molecules, as long as they contain the type of bond or chemical group that the enzyme targets. Enzymes are reusable. Enzymes are not reactants and are not used up during the reaction. Once an enzyme binds to a substrate and catalyzes the reaction, the enzyme is released, unchanged, and can be used for another reaction. This means that for each reaction, there does not need to be a 1:1 ratio between enzyme and substrate molecules. Components of Enzyme Apoenzyme- is an inactive enzyme, activation of the enzyme occurs upon binding of an organic or inorganic cofactor. are enzymes that lack their necessary cofactor(s) for proper functioning a Protein Holoenzyme- are the active forms of apoenzymes. (Apoenzyme plus cofactor) DNA polymerase and RNA polymerase are examples. Cofactor- mostly metal ions or small organic molecules, are inorganic and organic chemicals that assist enzymes during the catalysis of reactions. Nonprotein component (e.g., magnesium, zinc) Coenzyme- are non-protein organic molecules that are mostly derivatives of vitamins soluble in water by phosphorylation Organic cofactor (E.g.: NADH, FADH) Oxidation/Reduction Reactions Oxidation- addition of oxygen/electronegative element to a substance or removal of hydrogen/ electropositive element from a substance. Reduction- removal of oxygen/electronegative element from a substance or addition of hydrogen/ electropositive element to a substance. Oxidants- reactive molecules that are produced both inside your body and the environment that can react with other cellular molecules in your body such as protein, DNA and lipids. Reactants- a substance or compound added to a system to cause a chemical reaction, or added to test if a reaction occurs. Reagent- a substance consumed in the course of a chemical reaction. What is Oxidation/Reduction? An oxidation-reduction (redox) reaction is a type of chemical reaction that involves a transfer of electrons between two species. An oxidation-reduction reaction is any chemical reaction in which the oxidation number of a molecule, atom, or ion change by gaining or losing an electron. Redox reactions are common and vital to some of the basic functions of life, including photosynthesis, respiration, combustion, and corrosion or rusting. oxidation-reduction reactions are also called REDOX reactions all redox reactions involve the transfer of electrons from one atom to another spontaneous redox reactions are generally exothermic, and we can use their; released energy as a source of energy for other applications. Redox reactions are composed of two parts, a reduced half and an oxidized half, that always occur together. The reduced half gains electrons and the oxidation number decreases, while the oxidized half loses electrons and the oxidation number increases. Simple ways to remember this include the mnemonic devices OIL RIG, meaning "oxidation is loss" and "reduction is gain," and LEO says GER, meaning "loss of e- = oxidation" and "gain of e- = reduced." There is no net change in the number of electrons in a redox reaction. Those given off in the oxidation half reaction are taken up by another species in the reduction half reaction. What is an oxidizing and reducing agent? Oxidizing agent: a reagent which increases the oxidation number of an element of a given substance. These reagents are called oxidants. It contains the element that is reduced. Reducing agent: a reagent that lowers the oxidation number of a given element. These reagents are also called reductants. It contains the element that is oxidized. Factors Affecting Enzyme Activity Temperature o Raising temperature generally speeds up a reaction, and lowering temperature slows down a reaction. However, extreme high temperatures can cause an enzyme to lose its shape (denature) and stop working. Most enzymes have an optimum temperature, near a normal body temperature at which they catalyze. pH (abbr. power of hydrogen or potential for hydrogen) o Each enzyme has an optimum pH range. Changing the pH outside of this range will slow enzyme activity. Extreme pH values can cause enzymes to denature. Even small changes can alter the electrical charges on various chemical groups in enzyme molecules, thereby altering the enzymes ability to bind its substrate and catalyze a reaction. Enzymes catalyze a reaction most rapidly at an optimum pH, near neutral. Substrate Concentration o Increasing substrate concentration also increases the rate of reaction to a certain point. Once all of the enzymes have bound, any substrate increase will have no effect on the rate of reaction, as the available enzymes will be saturated and working at their maximum rate. At the saturation point, the reaction will not speed up, no matter how much additional substrate is added. The graph of the reaction rate will plateau. Enzyme concentration o Increasing enzyme concentration will speed up the reaction, as long as there is substrate available to bind to. Once all of the substrate is bound, the reaction will no longer speed up, since there will be nothing for additional enzymes to bind to. The higher the concentration of an enzyme the greater should be the initial reaction rate. This will last as long as the substrate is present Enzyme Inhibitors (Inhibition) o Competitive inhibitor- A molecule similar in structure to a substrate can bind to an enzymes active site and compete with the substrate. o Noncompetitive inhibitors- attach to the enzyme at an allosteric site, which is a site other than the active site distort the tertiary protein and alter the shape of the active site. Feedback Inhibition o Regulates the rate of many metabolic pathways when an end product of a pathway accumulates and binds to and inactivates the first enzyme in the metabolic pathway. Product (usually the ultimate product) of a pathway controls the rate of synthesis through inhibition of an early step (usually the first step). Conserves material and energy by preventing accumulation of intermediates.