Forrester Cardiovascular Physiology Fall 2024 PDF
Document Details
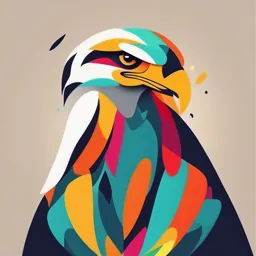
Uploaded by UpscaleQuantum
USC Alfred E. Mann School of Pharmacy and Pharmaceutical Sciences
2024
K. Forrester
Tags
Summary
This document provides an overview of cardiovascular physiology, specifically focusing on the electrical activity of the heart and the cardiac conduction system. It details the different phases of the cardiac action potential and how these relate to normal heart rhythm.
Full Transcript
Biological Systems I (PHRD 503) Fall 2024 K. Forrester, Pharm.D. Electrical Activity of the Heart Rhythmic impulses generated cause rhythmical contraction of heart muscle Normally. atria contracts ahead of ventricles allowing filling of ven...
Biological Systems I (PHRD 503) Fall 2024 K. Forrester, Pharm.D. Electrical Activity of the Heart Rhythmic impulses generated cause rhythmical contraction of heart muscle Normally. atria contracts ahead of ventricles allowing filling of ventricles All portions of ventricles contract almost simultaneously for effective pressure generation Heart disease, ischemia may impair the conduction system producing abnormal heart rhythm or abnormal sequence of contraction Cardiac Conduction System 1. S-A node: normal rhythmic self-excitatory impulses are generated. to the atria 2. Internodal pathways: conduct impulses from the S-A node to the A-V node 3. A-V node: impulses from the atria are delayed before passing into the ventricles 4. A-V bundle: conducts impulses from the atria to the ventricles 5. Purkinje fibers: conducts cardiac impulses to all parts of the ventricles Local differences in the action potential The cardiac action potential does not have the same shape in every cardiac cell. The action potentials that differ most radically from the Purkinje fiber model are found in the SA node and the AV node. Notice the slow depolarization phases (phase 0) in these action potentials Slow depolarization occurs because the SA nodal and AV nodal tissues lack active, rapid sodium channels and are thought to depend on the slow calcium channels for depolarization Because the speed of depolarization (the slope of phase 0) determines conduction velocity, the SA and AV nodes conduct electrical impulses slowly. Phase 0 – Na+ influx (Depolarization) Phase 1 - Early repolarization Phase 2 – Repolarization, plateau, Ca++ influx Phase 3 - Late repolarization, K+ efflux Phase 4 - Resting potential, automaticity, Na+ / K+ ATP pump 2 Action potential duration & Refractory period 3 The S-A node as Pacemaker Intrinsic rate of SA node discharge 70-80 times/min VS. AV node (40-60/min) and Purkinje fibers (15-50/min) SA node emits a new impulse before either AV node or Purkinje fibers reach their threshold for excitation Automaticity The property of cardiac sells to depolarize spontaneously Most cardiac fibers are capable of self-excitation, but normally only cells of the SA node, the AV node, and Purkinje system possess automaticity Fibers of the S-A node display self-excitation to the greatest extent Mechanism of S-A nodal automaticity Lesser negativity of SA nodal fiber during the resting potential Inactivated (blocked) sodium channels at this membrane potential Slow calcium channels can open "leaky" sodium channels make membrane potential less negative causing RMP to gradually rise to threshold potential Abnormal Pacemakers (ectopic pacemaker) Some other part of the heart develops a rhythmic discharge rate more rapid than the SA node Occurs if transmission of impulses from SA node is blocked May cause abnormal sequence of contraction Autonomic Control of Rhythmicity and Conduction Both sympathetic and parasympathetic fibers richly supply the SA and AV nodes. In the remainder of the heart’s electrical system, sympathetic innervation is abundant, while parasympathetic innervation is sparse. Thus, changes in parasympathetic tone have a relatively greater effect on the SA nodal and AV nodal tissues than they do on other tissues of the heart In general, an increase in sympathetic tone causes; enhanced automaticity (pacemaker cells fire more rapidly), increased conduction velocity (electrical impulses spread more rapidly), and decreased/shorter refractory periods (cells are ready for repeated depolarizations more quickly). Parasympathetic tone has the opposite effect; depressed automaticity, decreased conduction velocity, and increased/longer refractory periods 4 Acetylcholine (ACH) reduces rate of spontaneous depolarizations ACH increases the no. of open K+ channels, decreases no. of open Ca ++ channels Norepinephrine opens Ca ++ channels and closes K+ channels 5 Abnormal Rhythms of the Heart (Arryhthmogenesis) 1. Abnormal impulse generation Abnormal rhythmicity of the pacemaker Shift of the pacemaker from SA node to other parts of the heart Spontaneous generation of abnormal impulses in almost any part of the heart 2. Abnormal impulse conduction Blocks in transmission of impulses through the heart Abnormal pathways of impulse transmission Mechanisms of bradyarrhythmia a. Failure of impulse formation b. Failure of impulse Mechanisms of tachyarrhythmias a. Reentry (abnormal conduction) – accounts for most clinically significant tachyarrhythmias b. Enhanced Automaticity c. Triggered activity Reentry 6 The Electrocardiogram The cardiac action potential represents the electrical activity of a single cardiac cell. The surface EKG reflects the electrical activity of the entire heart. The instantaneous wave of depolarization can be followed across the heart by studying the EKG. Continuous record of cardiac electrical activity Provides info about rate and rhythm of excitation as well as pattern of conduction P wave: caused by electrical currents generated as atria depolarize QRS complex: ventricular depolarization prior to contraction T wave: ventricles recover from state of depolarization P-R interval: time from the onset of the P wave to the start of the QRS complex. It reflects conduction through the AV node (normal is 0.12-0.20 seconds) Q- T interval: time from beginning of the QRS complex to the end of the T wave. It represents the time it takes for the ventricles to depolarize and repolarize. The interval is longer when the heart rate is slower and shorter when the heart rate is faster, so it's necessary to calculate the corrected QT interval (QTc). 7 The Cardiac Pump Cardiac Cycle End of one heart contraction to the end of the next Initiated by the spontaneous generation of an action potential in the SA node Contraction begins a few milliseconds after the action potential and continues to contract a few milliseconds after the action potential ends Period of relaxation (diastole) followed by a period of contraction (systole) Function of ventricles as pumps During ventricular systole, blood accumulates in the atria, closed atrioventricular valves (mitral & tricuspid) Aortic/pulmonary valves open when left ventricular pressure exceeds aortic pressure When both mitral & aortic valves are closed, ventricles contract isovolumetrically (no change in ventricular volume). Contraction causes ventricular pressure to rise At ~ 80 mm Hg, aortic valve opens, blood flows from ventricles into aorta When rate of ejection begins to fall, ventricular pressure drops below atrial pressure, mitral valve opens allowing accumulated blood in the atrium during systole to flow rapidly into the ventricles Under normal resting conditions, atrial systole is not essential for ventricular filling, but when increased cardiac output is required, the absence of atrial systole can limit ventricular filling End-diastolic volume, End-systolic volume, Stroke volume During diastole, filling of the ventricles increases the volume of each ventricle to ~ 120-130 ml (end- diastolic volume) As the ventricles empty, volume decreases about 70 ml (stroke volume) Remaining volume in each ventricle about 50-60 ml (end-systolic volume) Fraction of the end-diastolic volume that is ejected (ejection fraction) is about 60% 7 Heart Sounds A. Aortic and pulmonic valves opening B. Atrioventricular valves closing D. Aortic and pulmonic valves closing C. Ventricles contracting E. Atrioventricular valves opening F. Ventricles relaxing First heart sound Second heart sound S1 (first heart sound): signals the beginning of systole. Occurs as ventricles contract and ventricular pressure rises above atrial pressure causing atrioventricular valves to close low pitched ("lub") caused by vibration of the valves and walls of the heart S2 (second heart sound): occurs at the end of systole when the aortic and pulmonary valves close. high pitched ("dub") may have two components corresponding to aortic valve closure and to pulmonary valve closure. Splitting of sound may be heard with inspiration and sometimes disappears with exhalation S3 (third heart sound): due to passive rapid filling of ventricles and can signify the presence of a cardiac abnormality (example: heart failure) S4 may be heard during atrial systole caused by blood movement due to atrial contraction. More common in patients with abnormality Cardiac Output CO = HR x SV (HR=heart rate, SV= stroke volume) Volume of blood pumped regulated by two basic factors: a. Response to changes in blood flowing into heart (venous return) b. Reflex control of the heart by the autonomic nervous system Factors influencing cardiac output I. Stroke volume A. Force of contraction 1. End-diastolic fiber stretch (preload, Frank-Starling's law) 2. Contractility a. Sympathetic stimulation mediated via beta-receptors b. Drugs (digoxin, anesthetics, toxins) c. Disease (coronary artery disease, myocarditis, etc.) B. Afterload II. Heart rate 8 Mechanism of Frank-Starling Law As stretch increases, the volume of blood ejected with each systolic contraction (stroke volume) increases When cardiac muscle is stretched an extra amount, as it does when extra amounts of blood enters the heart chambers, the stretched muscle contracts with a greatly increased force, thereby pumping extra blood into the arteries ( increase stretch → increase contractility) Volume of blood in ventricles at end of diastole (preload), sometimes expressed as pressure (LVEDP) Effects of contractility Increased contractility → more blood ejected Increased with increased sympathetic nerve activity Altered by drugs, disease Effects of hypertrophy Long term adaptation of the heart to stress Repeated bouts of increased cardiac output result in increased synthesis of contractile proteins and enlargement of cardiac muscle cells Ventricular cell enlarges, walls thicken and is capable of greater force development 9 Effect of Afterload Measurement of Cardiac Output The Fick principle Based on the principle described by Adolfo Fick in 1870, according to which the total uptake or release of a substance by an organ is the product of the blood flow through the organ and the arteriovenous concentration difference of the substance Because systemic arterial blood has the same O2 content in all parts of the body, the arterial O2 content can be measured in a sample obtained from any convenient artery CO = VO2 CaO2–CvO2 VO2 is the oxygen consumption by the lungs CaO2– CvO2 is the arteriovenous difference in oxygen Marked limitations in patients with lung abnormalities Costly Considered the most accurate method available to evaluate patients with low cardiac output Thermodilution Relies on a principle similar to indicator dilution, but it uses temperature rather than color as an indicator Uses a catheter (Swan-Ganz) inserted from a central vein into the pulmonary artery. ◦ A cold solution (ex. normal saline) is injected into the right atrium from a proximal catheter port. ◦ This solution causes a decrease in blood temperature, which is measured by a thermistor (sensor) placed in the pulmonary artery catheter ◦ The decrease in temperature is inversely proportional to the dilution of the injectate Advantages: (1) saline is completely innocuous (2) the cold is dissipated in the tissues so recirculation is not a problem; easy to make repeated determinations. Risks: Pneumothorax, dysrythmias, perforation of the heart chamber, tamponade and valve damage 10 Circulation and Hemodynamics Hemodynamics describes the relationships governing the physical principles of pressure, flow, resistance and compliance as they relate to the cardiovascular system Poiseuille's Law Fluid flows when a pressure gradient exists Volume of fluid flowing through a rigid rube per unit time (flow) is proportional to the pressure difference between the ends of the tube 1. Blood Flow = ΔP π r4 8nL Most important determinants of blood flow are ∆P and r 2. Effect of blood viscosity (hematocrit) Percentage of blood that is cells Greater the Hct, greater the friction Contribution is minimal 3. Resistance to flow R= P/Flow = 8nL π r4 Not measured by any direct means (calculated from P and Flow) Depends on the diameter of blood vessels 11 Streamlined or Turbulent Flow Streamlined Flow Orderly, silent Fluid exerts least resistance to flow Concentric layers of fluid slip past each other Turbulent Flow Chaotic, disorganized Crosscurrents Clinical Significance: a. Turbulence is important because it can damage platelets and induce development of blood clots. b. Diameter decreased i. Constriction of a blood vessel ii. Narrowing of a heart valve c. Rate of flow increased i. Pregnancy ii. Hyperthyroidism d. Viscosity decreased i. Anemia Factors Contributing to Turbulence a. high flow velocity b. large tube diameter c. high fluid density d. low viscosity 12 Reynolds Number A dimensionless number in fluid mechanics Convenient parameter for predicting if a flow condition will be laminar or turbulent. o Less than 2100: flow tends to be laminar o Greater than 4000: leads to turbulent flow o Transitional Flow prevails between these two limits 13 Cross-sectional areas and velocity of blood flow cm2 Aorta 2.5 Small arteries 20 Arterioles 40 Capillaries 2500 Venules 250 Small veins 80 Vena Cava 8 Velocity of blood flow inversely proportional to cross-sectional area Pressures and resistance in various segments of systemic circulation Small arteries, arterioles & capillaries account for 90% of vascular resistance Pressure falls progressively (R. atrium = 0 mm Hg) Decrease in arterial pressure in each segment is directionally proportional to the vascular resistance in the segment SVR is frictional resistance to blood flow 14 Relationship of SVR to individual tissue and organ resistance For a system in series SVR = Rsmall arteries + Rarterioles + Rcapillaries + Rvenules + Rsmall veins For a system arranged in parallel, each receives a percentage of total cardiac output 1 = 1 + 1 + 1 + 1 + 1 SVR Rbrain Rcoronary Rrenal Rmuscle Rskin Overview: As blood flows through one arterial vascular segment after another: 1. Number of vessels: 2. Diameter of vessels: 3. Total cross· sectional area: 4. Velocity of blood flow: 5. Pressure: 6. Vascular resistance: 15 The Arterial System Converts the intermittent flow generated by the heart to a virtual steady flow through the capillaries Compliance Vessels expand in proportion to compliance when blood in them is under pressure C = V/P V = change in volume P = distending pressure (Pinside - Poutside) = transmural pressure With age, aorta becomes less compliant and aortic pressure rises more for a given increase in aortic volume Veins have greater volume capacity Systolic and Diastolic pressures Pressure in arterial system rises and falls with each beat Normal BP? Pulse pressure = SBP - DBP Factors influencing pulse pressure a. Stroke volume b. Compliance Mean Arterial Pressure Arterial Pressure = CO x PR (PR = total resistance in systemic circulation) determined by volume of blood in arteriole system MAP = DBP + (SBP-DBP) 3 16 Factors affecting blood pressure 1. Age 2. Gender 3. Diet 4. Weight 5. Pregnancy 6. Behavioral (smoking, Etoh intake, illicit drugs, medications) 17 Microcirculation and Lymphatic Systems Microcirculation 1. Exchanges nutrients, water, gases, hormones and waste products a. Diffusion (concentration gradient) b. Bulk Flow (pressure gradient) 2. Regulates vascular resistance to maintain adequate arterial pressure Local control of blood flow through the microcirculation flow controlled mainly in proportion to individual tissue needs the greater the degree of metabolism in the organ, the greater the blood flow 1. Acute control (short term) Occurs through autoregulation and vasomotion Rapid changes in blood flow occurring within seconds to minutes a. Oxygen demands decreased [O2] → vessels relax → blood flow increased b. Release of vasoactive substances EDRF (probably nitric oxide) → relaxation of vascular smooth muscle ACH, histamine, ADP, ATP may also be involved Endothelin (vasoconstrictor) 18 2. Long-term control Slow change in blood flow (hours, days, weeks) May involve increase or decrease in sizes and numbers of vessels supplying the tissue Provides "fine tuning" of blood flow through tissues/organs a. Changes in tissue vascularity - May result from constant lack of oxygen or stretch - Age dependent, change more rapid in younger individuals - Influenced by aerobic exercise b. Development of Collateral Circulation - A1ternate routes - Result of blocked blood flow to tissue or relative lack of oxygen to level of metabolism - Especially important in the heart Nervous system control of blood flow Affects blood flow in large segments of the systemic circulation Sympathetic vasoconstrictor nerves innervate blood vessels arterioles > > Small arteries > venules > Large arteries > > capillaries Vessels in partial state of contraction (vasomotor tone) Neurotransmitter (norepinephrine) stimulates alpha receptors → vasoconstriction Effective under special circumstances (example: Humoral control of blood flow substance in blood: hormones, ions, other chemicals can cause local increase or decrease in tissue blood flow or a widespread generalized change potentiates vasoconstriction: epinephrine, norepinephrine, angiotensin, vasopressin potentiates vasodilation: bradykinin, histamine, prostaglandins Epinephrine: Angiotensin: Histamine: 19 Capillary Dynamics Diffusion 1. Diffusion of lipid-soluble substances - Diffuses directly through cell membranes - ex. oxygen, carbon dioxide 2. Diffusion of water molecules - Occurs through "pores" in capillary membrane 3. Diffusion of water-soluble, Lipid-insoluble substances Occurs through capillary pores ex. sodium, chloride, glucose ' Effect of molecular size Permeability of pores for different substances varies according to molecular diameters. Capillaries in different tissues have differences in their permeability Effect of concentration difference Net rate of diffusion is proportional to the concentration difference. Slight concentration differences affect transport between plasma and interstitial fluid Bulk Flow - Effect of Hydrostatic pressure difference More water and dissolved substance move through membrane than can be accounted for by increase in diffusion alone Occurs when hydrostatic pressure is different on two 'sides of the capillary membrane. Effect of Osmotic Pressure difference Osmotic pressure exerted by substances with large MW unable to move through capillary membrane primarily exerted by plasma proteins 20 Forces that determine fluid movement 1. Capillary pressure Tends to move fluid outward through capillary membrane, averages 30-40 mm Hg at arterial end and 10–17 mm Hg at the venous end 2. Interstitial fluid pressure 3. Plasma colloid osmotic pressure - Causes osmosis of fluid inward through capillary membrane 4. Interstitial fluid colloid osmotic pressure - Cause osmosis of fluid outward - Due to plasma proteins that "leak" into interstitial spaces Lymphatic System Accessory route which returns fluid from interstitial spaces to blood Also transports proteins and large particulate matter Important in body defense against bacterial invasion 21 Formation of lymph Derived from interstitial fluid that flows into lymphatics Protein concentration averages about 2 grams per cent Thoracic duct lymph may contain as much as 1-2 percent fat Determinants of lymph flow rate 1. Interstitial fluid pressure (a) Elevated capillary pressure (b) Decreased plasma colloid osmotic pressure (c) Increased interstitial fluid protein (d) Increased capillary permeability 2. The lymphatic pump Valves External compression Edema from abnormal capillary dynamics Edema = presence of excess interstitial fluid in the tissues 1. Increased capillary pressure Venous obstruction - blood clots Arteriolar dilation Cardiac failure 2. Decreased plasma proteins Extensive burns - albumin lost in large quantities Nephrotic syndrome - kidney losses Nutritional 3. Lymphatic obstruction Infection 4. Increased capillary permeability Burns - destroys the integrity of capillary endothelium Allergic reactions – histamine release 22 Pressures in the Cardiovascular System 1. Hemodynamic Produced by systolic contractions Stored in elastic walls of blood vessels 2. Hydrostatic Caused by force of gravity acting on the fluid (wt. of blood vessels) Effect of gravity on systemic circulation a. Central venous pressure (rt. atrial pressure) Important! Pressure in veins of thoracic cavity Good indicator of central blood volume Regulated by balance between (I) ability of heart to pump blood out of rt. atrium and (2) tendency for blood to flow from peripheral vessels to rt. atrium Approximately 2-6 mm Hg (may range from -5 to + 30 mm Hg) b. Peripheral venous pressure range from 0 - 90 mm Hg In person lying down: (numbers not necessary to memorize) 5 mm Hg in feet 5-6 mm Hg in jugular vein 7 mm Hg in arm due to compression of rib CVP = 2-6 mm Hg In person standing upright CVP = 2-6 mm Hg Pressure in ankles = 90 mm Hg (standing still) Sinuses in head, pressure may fall below 0 mm Hg In person walking around: Pressure in ankles 25 mm Hg 23 C. Venous return assist mechanisms (i) Venous pump or muscle pump - moving the legs/tightening leg muscles squeezes blood out of veins - pressure in gravity-dependent veins is lowered (ii) Abdomino-thoracic pumping mechanism contraction/relaxation of diaphragm squeeze veins in abd. cavity and forces blood towards heart 24 Overall Cardiovascular Control BP = CO x TPR SNS Heart Stroke Rate Distensibility Volume of vessels Pacemaker Diastolic Myocardial Activity Vent. Volume Contractility ANS Venous Filling SNS Return Pressures Blood Volume Renal Mechanisms Reflex Control of Systemic Blood Pressure a. Rapid acting pressure control mechanism Decrease in ability to control arterial pressure over hours to days, if BP remains elevated b. Long-term mechanism for pressure regulation Carotid Sinus baroreceptors and Aortic baroreceptors Oppose increases and decreases in arterial pressure Increased pressure stretches receptors → transmit signals to CNS → feedback signals sent through ANS to the circulation to decrease art. pressure to normal level Quick change in BP (within seconds) Designed to maintain cerebral blood flow Lower limit of response approx. 60 mm Hg (carotid) and 100 mm Hg (aortic) 25 Adaptation of baroreceptors Short term, adapt after 1-2 days to new blood pressure level (Fill in the diagram) BP Nerve Activity Examples of function: (Fill in the examples) a. Orthostatic (postural) hypotension b. Hemorrhage c. CV shock d. Heart failure 2. CNS Ischemic Response Emergency response, last resort to maintain blood flow Vasomotor center response to diminished brain blood flow Powerful mechanism, degree of sympathetic vasoconstriction may cause total occlusion of some peripheral vessels Activated below 40 mm Hg Special case = Cushing Reaction ◦ Results from increased pressure in cranial vault (ex. head injury and accumulation of fluid in brain) ◦ Compression of arteries decreases blood supply in brain ◦ Activation raises art. pressure above CSF pressure to relieve ischemia 26 3. Chemoreceptor Reflexes Control of art. pressure by carotid and aortic chemoreceptors Monitors content of blood (O2, CO2, H+) At critical level, chemoreceptors stimulated and signals transmitted to vasomotor center Does not respond strongly until art. pressure falls to 40-60 mm Hg 4. Hormonal mechanisms for rapid control of arterial pressure a. Vasopressin release mechanism b. Renin-angiotensin mechanism (See #10 below) c. Norepinephrine-epinephrine release mechanism Epi released from adrenal medulla in response to sympathetic nervous system stimulation Circulated in blood up to 3 mins. & provides slightly longer excitation of the circulation Able to reach parts of circulation with no sympathetic nervous supply Same effect in controlling art. pressure as direct sympathetic stimulation 5. Other stretch receptors: Bainbridge reflex Atrial reflex control of heart rate Stretch receptors respond to increased atrial pressure(volume) Reflex causes increase in heart rate & prevents damming of blood in veins, atria 6. Overview of Reflex Blood Pressure Control (Fill in the blanks) Carotid baroreceptors:____________________________________________________ Aortic baroreceptors: _____________________________________________________ CNS ischemic response:___________________________________________________ Chemoreceptors: ________________________________________________________ 7. Capillary Fluid Shift Control of Blood Pressure Vascular Fluid Interstitial Fluid Volume Volume Independent of nervous system Activated within minutes (up to 30 mins.) Fluid shifts in response to increase/decrease capillary pressure 27 8. Stress- Relaxation Mechanism of Blood Pressure Control Pressure Time Independent of nervous system Activated within minutes (up to 30 mins.) Important in veins Responds to loss of volume and conditional increases in volume (transfusions) Slow relaxation/constriction of walls of veins in response to pressure changes 9. Renal-Body Fluid Mechanism Long term mechanism for arterial pressure regulation Powerful but slow Kidneys control BP in “0 to infinity range” Urine Output-Blood Pressure Curve Kidneys balance urine output with dietary intake of Na+ & H20 to maintain BP 28 10. Renin-Angiotensin-Aldosterone Mechanism Independent of nervous system Slow, long-term mechanism 11. Overall Influences on Blood Pressure Extracellular Blood Volume Central Venous Fluid Volume Pressure Renal Blood CO Venous Return Function Pressure To Heart Renin-Angiotensin Aldosterone System 29 Coronary Circulation Coronary circulation is responsible for delivering blood to the heart tissue itself (the myocardium) Close parallel between the level of myocardial metabolic activity and the magnitude of the coronary blood flow About 4-5% of total cardiac output Myocardial ischemia results when the arterial blood supply fails to meet the needs of the heart muscle, for oxygen and/or metabolic substrates In most tissues, blood flow peaks during ventricular systole due to increased pressure in the aorta and its branches. However, blood flow through the coronary vessels, seems paradoxical and peaks during ventricular diastole ◦ This unusual pattern is a result of external compression of coronary vessels by myocardial tissue during systole Control of Coronary Blood Flow Local metabolic regulators of arteriolar tone are usually the most important for coronary flow regulation Whenever the heart activity is increased, the rate of coronary flow is also increased. This also applies to decreased activity Major factor is oxygen demand, vasodilator substances released (CO2, O2, Adenosine, NO) Several conditions exist that can lead to such a mismatch between oxygen demand and delivery to the myocardium It appears that a decrease in the ratio of O2 supply to O2 demand (whether produced by a reduction in O2 supply or by an increment in O2 demand) releases vasodilator substances from the myocardium 30 Cerebral Circulation Brain function critically depends on a close matching between metabolic demands, appropriate delivery of oxygen and nutrients, and removal of cellular waste. This matching requires continuous regulation of cerebral blood flow (CBF) Supplies oxygen, glucose, and nutrients Removes CO2, lactic acid and metabolites Using a simplified approach, mechanisms that regulate CBF can be divided into four distinct components: 1. Autoregulation - relates to the response of the cerebral circulation to changes in cerebral perfusion pressure. Cerebral perfusion pressure is determined by BP, ICP, and venous pressure 2. Chemoregulation - vascular responses to changes in CO2, pH, pO2, or O2 content. Changes in these variables (ex. hypocapnia and hypercapnia) elicit strong CBF responses 3. Neuronal regulation - consists of the influence of nerves or neurons on CBF (sympathetic discharge) 4. Endothelium-dependent regulation - relates to the effects of vascular endothelial cells on vascular tone and, therefore, CBF Blood flow remains fairly constant, over a wide range of arterial pressure changes by autoregulation Exact mechanisms of autoregulation unknown Microvessels sensitive to [CO2]and [ H+] Cerebral vasculature also dilates if [O2l content of art. blood is low, but vasodilatory effect of elevated [CO2] more powerful Relatively insensitive to hormone and sympathetic nerve activity Adapts to chronic high blood pressure