Forensic Biology PDF - Identification of Blood
Document Details
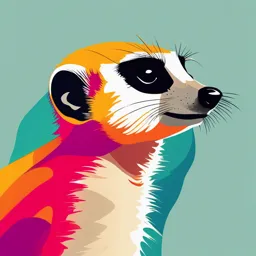
Uploaded by GreatAlpenhorn
Bundelkhand University
Tags
Summary
This document discusses the identification of blood, including its biological properties, and presumptive assays. It details the mechanisms of these assays, such as oxidation-reduction reactions, and various colorimetric, chemiluminescence, and fluorescence assays.
Full Transcript
12 Identification of Blood 12.1 Biological Properties Blood constitutes about 8% of the human body weight of a healthy individual. Plasma is the fluid portion of the blood. The cellular port...
12 Identification of Blood 12.1 Biological Properties Blood constitutes about 8% of the human body weight of a healthy individual. Plasma is the fluid portion of the blood. The cellular portion of the blood consists of red blood cells, white blood cells, and platelets, all of which are suspended in the plasma (Figures 12.1 and 12.2). 12.1.1 Red Blood Cells These cells are also called erythrocytes. Their life span in humans is approximately 3–4 months. Additionally, mature human erythrocytes do not have nuclei, and therefore lack nuclear DNA. Erythrocytes consist of hemoglobin—proteins that are responsible for the transportation of oxygen. Most adult human hemoglobin consists of four polypeptide chains, two α chains and two β chains. Thus, adult hemoglobin is designated as α2β2. Other forms of hemoglobin will be discussed in Chapter 18. Under normal physiological conditions, each hemoglobin subunit con- tains a heme moiety that binds to oxygen (Figure 12.3). A heme molecule consists of an organic component known as protoporphyrin IX and a ferrous (Fe2+) iron ion (Figure 12.4). A heme molecule is also known as ferroprotoporphyrin. The ferrous ion of heme forms four bonds with the nitrogens of protoporphyrin IX, along with a fifth bond with a hemoglobin chain and a sixth bond with a molecule of O2. Other chemicals such as carbon monoxide and cyanide also bind to the ferrous iron of the heme molecule and can cause chemical asphyxia. Heme groups are also present in the blood of various animals and in other proteins such as myoglobin in muscles and neuroglobin in the brain. 12.1.2 White Blood Cells Also called leucocytes, white blood cells are subdivided into three types: granulocytes, lym- phocytes, and monocytes. White blood cells are involved in defending the body against infection. They have nuclei and thus represent the main sources of nuclear DNA from the blood. 12.1.3 Platelets These cells are also known as thrombocytes, and they play a role in blood clotting (Chapter 16). Platelets aggregate at sites of vascular and blood vessel injury. Like erythrocytes, they lack nuclei. 231 Forensic Biology, Second Edition Plasma Centrifuge Erythrocyte Leucocyte Whole blood Cellular fraction (with anticoagulant) Platelet Figure 12.1 Basic composition of blood. Blood can be separated into two phases in the presence of an anticoagulant. The liquid portion called plasma accounts for approximately 55% of blood volume. The cellular elements include erythrocytes, leucocytes, and platelets. (© Richard C. Li.) Figure 12.2 Blood cells. (© Richard C. Li.) 12.2 Presumptive Assays for Identification 12.2.1 Mechanisms of Presumptive Assays Presumptive blood assays are designed to detect traces of blood. These assays are based on the basic principle of the oxidation–reduction reaction catalyzed by the heme moiety of the hemo- globin. As a result, colorless substrates catalyzed by heme undergo an oxidation reaction, caus- ing either chemiluminescence, fluorescence, or a change of color. These assays are very sensitive and can detect blood in samples with 10 –5−10 –6-fold dilutions. A positive reaction indicates the possible presence of blood. Additionally, most of these assays do not interfere with forensic DNA analysis. 232 12.2 Presumptive Assays for Identification Heme moiety α Chain β Chain α Chain β Chain Heme moiety Figure 12.3 Human adult hemoglobin. Four subunits, two α and two β chains, of human adult hemoglobin are shown. Each hemoglobin subunit contains a heme moiety. (© Richard C. Li.) H2C H2C CH3 CH3 H3C H3C NH N CH2 CH2 N N Fe N N N HN H 3C CH3 H3C CH3 HOOC COOH HOOC COOH (a) (b) CH2 CH3 CH2 CH3 CH3 CH3 N R N CH2 N R N CH2 Fe Fe N R N N N CH3 CH3 CH3 CH3 HOOC COOH HOOC COOH (c) (d) Figure 12.4 Chemical structures of heme and its precursor and derivatives. (a) Protoporphyrin IX. (b) Heme (ferroprotoporphyrin). (c) Hemochromagen, R = pyridine (pyridineferroprotoporphy- rin). (d) Hematin hydroxide, R = OH (ferriprotoporphyrin hydroxide); hematin chloride, R = Cl (ferriprotoporphyrin chloride). 233 Forensic Biology, Second Edition 12.2.1.1 Oxidation–Reduction Reactions An oxidation–reduction reaction involves a change in the oxidation state of a molecule. Specifically, the oxidation of a molecule means that the molecule has lost electrons, and the reduction of a mol- ecule means that the molecule has gained electrons. Chemicals that can be reduced and therefore gain electrons from other molecules are called oxidants. In contrast, reductants are chemicals that can be oxidized and therefore lose electrons to other molecules. In biochemical reactions, oxida- tion often coincides with a loss of hydrogen. Figure 12.5 depicts an example of an oxidation–reduc- tion reaction for blood identification. In presumptive assays, heme is utilized as the catalyst, and hydrogen peroxide is utilized as the oxidant for the reaction. In the presence of heme, a colorless substrate is oxidized, yielding a product with color, chemiluminescence, or fluorescence. 12.2.2 Colorimetric Assays Many procedures are available for detecting heme in blood through color reactions. The most common agents are phenolphthalin, leucomalachite green, and benzidine derivatives. The color reactions produced by these assays can be observed immediately with the naked eye. 12.2.2.1 Phenolphthalin Assay Phenolphthalein, a member of a class of indicators and dyes, is used in titrations of mineral and organic acids as well as most alkalis. The phenolphthalin assay for blood identification is also known as the Kastle–Meyer test. Kastle published a study in 1901, presenting the results of a reaction in which phenolphthalin, a colorless compound, is catalyzed by heme with hydrogen peroxide as the oxidant (Figures 12.6 and 12.7). The oxidized derivative is phenolphthalein, which appears pink under alkaline conditions. 12.2.2.2 Leucomalachite Green (LMG) Assay Malachite green is a triphenylmethane dye. The leuco base form of malachite green is colorless and can be oxidized by the catalysis of heme to produce a green color. The reaction is carried out under acid conditions with hydrogen peroxide as the oxidant (Figures 12.8 and 12.9). 12.2.2.3 Benzidine and Derivatives Historically, benzidine was used as an intermediate in dye manufacturing (Figure 12.10). Subsequently, it was used as a presumptive assay for the presence of blood after the discovery that the oxidation of benzidine can be catalyzed by heme to produce a blue to dark blue color (carried out in an acid solution). Since the blue color may eventually turn brown, the reaction must be read Heme AH2 + H2O2 A + 2H2O (Colorless) (Color) Figure 12.5 Oxidation–reduction reaction as the basis for presumptive assays for blood identifica- tion. AH2, substrate; A, oxidized substrate. HO OH HO O H2O2 Heme COOH COOH Phenolphthalin Phenolphthalein (a) reduced (colorless) (b) (c) oxidized (pink) Figure 12.6 Chemical reaction of phenolphthalin assay. 234 12.2 Presumptive Assays for Identification Figure 12.7 Photograph of phenolphthalin assay results. Negative (left) and positive (right) reac- tion. (© Richard C. Li.) N(CH3)2 N(CH3)2 H2O2 Heme N(CH3)2 NCH3 Leucomalachite green Malachite green reduced (colorless) oxidized (blue-green) Figure 12.8 Chemical reaction of leucomalachite green assay. Figure 12.9 Photograph of leucomalachite green assay results. Negative (left) and positive (right) reaction. (© Richard C. Li.) 235 Forensic Biology, Second Edition H2N NH2 (a) H 3C CH3 H2N NH2 (b) H3C CH3 H2N NH2 H 3C CH3 (c) Figure 12.10 Chemical structures of benzidine and derivatives: (a) benzidine; (b) orthotolidine; (c) tetramethylbenzidine. immediately. Benzidine was found to be a carcinogen and is therefore no longer used for forensic testing. Orthotolidine is a dimethyl derivative of benzidine. Its oxidation reaction can be catalyzed by heme to produce a blue color reaction under acidic conditions (Figure 12.11). Orthotolidine is also considered a potential carcinogen based on animal studies, and for this reason it has been replaced by tetramethylbenzidine. Tetramethylbenzidine (TMB) is a tetramethyl derivative of benzidine. The oxi- dation of TMB can be catalyzed by heme to produce a green to blue-green color under acidic condi- tions. TMB continues to be used. The Hemastix® assay kit (Miles Laboratories) is a TMB-based assay that utilizes a TMB-containing strip device. A test is carried out by applying a moistened sample to a Hemastix® strip. The appearance of a green or a blue-green color indicates the presence of blood. 12.2.3 Chemiluminescence and Fluorescence Assays Other organic compounds whose oxidation products have chemiluminescent or fluorescent properties are utilized for testing. In the chemiluminescence assay, light is emitted as a product of a chemical reaction. In this category, luminol produces chemiluminescence when blood is present. In contrast, a fluorescence assay requires the exposure of an oxidized product, such as fluorescin, to a particular wavelength of an excitation light source. The fluorescence is then emit- ted at longer wavelengths than that of the excitation light source. One advantage of chemiluminescent and fluorescent reagents is that they can be sprayed over large areas where latent bloodstains are potentially located. A positive reaction identifies blood and also reveals the patterns of bloody impressions such as footprints and fingerprints. These methods are very sensitive and can pinpoint the locations of even small traces of blood. Additionally, they are useful for detecting blood at crime scenes that have been cleaned and show no visible staining. One disadvantage of chemiluminescent and fluorescent reagents is that precautions must be taken if stains are very small or have been washed. The spraying of H3C CH3 H2O2 H3C CH3 Heme H2N NH2 HN NH Orthotolidine Orthotolidine reduced (colorless) oxidized (blue) Figure 12.11 Chemical reaction of orthotolidine assay. 236 12.2 Presumptive Assays for Identification presumptive assay reagents may further dilute a sample and thus lead to difficulty in isolating sufficient amounts of DNA for forensic DNA analysis. 12.2.3.1 Luminol (3-Aminophthalhydrazide) Luminol is usually utilized as a chemiluminescent reagent. The oxidation reaction of luminol catalyzed by heme produces light in the presence of an oxidant (Figures 12.12 through 12.14). The light emitted from a positive reaction can only be observed in the dark, which limits the O O H2O2 NH O Heme + N2 + H2O + NH hv O NH2 O NH2 O Luminol 3-Aminophthalate Figure 12.12 Chemical reaction of luminol assay. Figure 12.13 Results obtained from testing a drop of blood on a glass plate with luminol. From left to right: drop of blood; drop of blood after spraying with luminol; drop of blood after spraying with luminol and viewing in the dark. A blue chemiluminescence indicates the presence of blood. (From Bergervoet, P.W., et al., J Hosp Infect, 68, 329–333, 2008. With permission.) Figure 12.14 Detecting latent bloodstains on a floor with luminol. Left: with the light on; right: immediately after the light is switched off. A blue chemiluminescence indicates the presence of blood traces. (From Bergervoet, P.W., et al., J Hosp Infect, 68, 329–333, 2008. With permission.) 237 Forensic Biology, Second Edition applications of luminol. The photodocumentation of a luminol-enhanced pattern should be done immediately before it fades away. 12.2.3.2 Fluorescin Fluorescin is another reagent that is used to test for the presence of bloodstains at a crime scene (Figures 12.15 and 12.16). When oxidized and catalyzed by heme, fluorescin demonstrates fluo- rescent properties. Usually, fluorescin-sprayed stains are exposed to light in the range of 425– 485 nm using an alternate light source device. In a positive reaction, the oxidized fluorescin emits an intense yellowish-green fluorescent light, which indicates the presence of a bloodstain. The light emitted from fluorescin-sprayed stains lasts longer than that of luminol. 12.2.4 Factors Affecting Presumptive Assay Results The catalytic assays discussed in the previous sections are not specific to blood only, which can possibly lead to the observation of false-positive or false-negative results (Figure 12.17). 12.2.4.1 Oxidants Chemicals that are strong oxidants may cause a false-positive reaction. Such chemicals can catalyze the oxidation reaction even in the absence of heme and result in a false-positive reac- tion. Certain metal salts, such as copper and nickel salts, household bleaches and cleaners that contain hypochlorite ions, and hair-coloring products that contain hydrogen peroxide, work as oxidants. To address this problem, a two-step catalytic assay should be performed. The substrate HO O OH COOH Figure 12.15 Chemical structure of fluorescein. Figure 12.16 Detecting diluted bloodstains on black cotton fabric with fluorescin (left) and Bluestar (right). (From Finnis, J., Lewis, J., and Davidson, A., Sci Justice, 53, 178–186, 2013. With permission.) (1) Oxidant (2) Peroxidase AH2 + H2O2 A + 2H2O (colorless) (3) Reductant (color) Figure 12.17 Factors affecting presumptive assay results. Strong oxidant and peroxidase may cause false-positive results; reductant may cause false-negative results. 238 12.3 Confirmatory Assays for Identification is applied first to the sample in question. A color change occurring before the addition of hydro- gen peroxide indicates a false-positive result due to a possible oxidant in the sample. If a color change is observed after the addition of hydrogen peroxide, the result is a true positive. 12.2.4.2 Plant Peroxidases Many types of plants such as horseradish contain peroxidases. Plant peroxidases may also cata- lyze oxidation reactions and lead to false-positive results. However, plant peroxidases are usu- ally heat sensitive and may be inactivated by high temperatures. Because the heme molecule is relatively stable at high temperatures, samples can be retested after heating. This will inactivate any plant peroxidases in a sample. 12.2.4.3 Reductants Although not common, a false-negative result can occur when a strong reductant is present in a sample. Strong reductants such as certain metal ions including lithium and zinc may inhibit the oxidation reaction. 12.3 Confirmatory Assays for Identification 12.3.1 Microcrystal Assays Microcrystal assays apply chemicals to treat bloodstains, forming crystals of heme molecules. The morphologies of the resulting crystals are distinctive for heme and can be compared with a known standard using a microscope. A positive microcrystal assay strongly indicates the pres- ence of blood. However, confirmatory assays are usually not as sensitive as presumptive assays. Additionally, these assays cannot distinguish between human and animal blood. 12.3.1.1 Hemochromagen Crystal Assay Hemochromagens are heme derivatives in which the ferrous iron of the heme forms two bonds with nitrogenous bases (Figure 12.4). The method for forming hemochromagen crystals was documented in 1864. Since then, various modifications have been reported. The Takayama crys- tal assay, published in 1912, has been the method preferred by many forensic laboratories. A bloodstain is treated with pyridine and glucose (a reducing sugar that is capable of reducing ferric ion) under alkaline conditions to form crystals of pyridine ferroprotoporphyrin (Figure 12.18). Figure 12.18 Microcrystal assays using the Takayama method. (© Richard C. Li.) 239 Forensic Biology, Second Edition Figure 12.19 Microcrystal assays using the Teichmann method. (From James, S.H., Nordby, J.J., and Bell, S., Forensic Science: An Introduction to Scientific and Investigative Techniques, 4th edn., CRC Press, Boca Raton, 2014. With permission.) 12.3.1.2 Hematin Crystal Assay This assay is also known as the Teichmann crystal assay. In 1853, Teichmann documented a method of forming crystals of blood specimens. When blood specimens are treated with glacial acetic acid and salts, and subsequently heated, hematin chloride (ferriprotoporphyrin chloride), a prismatic brown-colored crystal, is formed (Figure 12.19). Hematin (Figure 12.4) is a heme derivative; its iron is in the ferric (Fe+3) state. This hematin assay has a similar sensitivity and specificity as hemochromagen assays. The hematin assay has the advantage of being more reli- able than hemochromagen assays for aged blood samples. 12.3.2 Other Assays Additional techniques may be used to confirm the presence of hemoglobin. For example, chro- matographic and electrophoretic methods can identify hemoglobin by its mobility characteris- tics. Spectrophotometric methods for identifying hemoglobin are based on measurements of the Table 12.1 Application of RT-PCR Assay for Blood Identification Gene Symbol Gene Product Description Further Reading HBA1 Hemoglobin α1 Hemoglobin α1 chain Waye and Chui (2001) (abundant in erythrocytes) PBGDa Porphobilinogen Erythrocyte-specific isoenzyme Gubin and Miller (2001) deaminase of heme biosynthesis pathway SPTB β-Spectrin Subunit of major protein of Amin et al. (1993) erythrocyte membrane skeleton Source: Adapted from Juusola, J. and Ballantyne, J., Forensic Sci Int, 152, 1–12, 2005; Nussbaumer, C., Gharehbaghi-Schnell, E., and Korschineck, I., Forensic Sci Int, 157, 181–186, 2006. a Also known as hydroxymethylbilane synthase (HMBS). 240 Bibliography characteristic light spectra, with peak absorbance at 400–425 nm, absorbed by hemoglobin and its derivatives. Finally, immunological methods utilize antihuman hemoglobin antibodies. This antibody can be used to detect human hemoglobin and thus indicate the presence of human blood (see Chapter 13). Recently, RNA-based assays have been developed to identify blood. These assays are based on the fact that certain genes are specifically expressed in certain cell types (Chapter 11). Thus, the techniques used in the identification of blood are based on the detection of specific types of mes- senger RNA (mRNA) that are expressed exclusively in erythrocytes. These assays utilize reverse transcriptase polymerase chain reaction (RT-PCR; see Chapter 7) methods to detect the gene expression levels of mRNAs for blood identification. Table 12.1 summarizes the tissue-specific genes utilized for blood identification. Compared with conventional assays that are used for blood identification, the RNA-based assays have higher specificity and are amenable to automa- tion. However, one limitation is that RNA is unstable due to degradation by endogenous and environmentally born ribonucleases. Bibliography Amin, K.M., et al., The exon-intron organization of the human erythroid beta-spectrin gene. Genomics, 1993, 18(1): 118–125. Andersen, J. and S. Bramble, The effects of fingermark enhancement light sources on subsequent PCR-STR DNA analysis of fresh bloodstains. J Forensic Sci, 1997, 42(2): 303–306. Anderson, S.E., G.R. Hobbs, and C.P. Bishop, Multivariate analysis for estimating the age of a bloodstain. J Forensic Sci, 2011, 56(1): 186–193. Anderson, S., et al., A method for determining the age of a bloodstain. Forensic Sci Int, 2005, 148(1): 37–45. Andrasko, J., The estimation of age of bloodstains by HPLC analysis. J Forensic Sci, 1997, 42(4): 601–607. Anslinger, K., et al., Ninhydrin treatment as a screening method for the suitability of swabs taken from con- tact stains for DNA analysis. Int J Legal Med, 2004, 118(2): 122–124. Arany, S. and S. Ohtani, Age estimation of bloodstains: A preliminary report based on aspartic acid racemi- zation rate. Forensic Sci Int, 2011, 212(1–3): e36–e39. Barnett, P.D., et al., Discussion of “Effects of presumptive test reagents on the ability to obtain restriction fragment length polymorphism (RFLP) patterns from human blood and semen stains”. J Forensic Sci, 1992, 37(2): 369–370. Bauer, M., S. Polzin, and D. Patzelt, Quantification of RNA degradation by semi-quantitative duplex and competitive RT-PCR: A possible indicator of the age of bloodstains? Forensic Sci Int, 2003, 138(1–3): 94–103. Bergervoet, P.W., et al., Application of the forensic Luminol for blood in infection control. J Hosp Infect, 2008, 68: 329–333. Botoniic-Sehic, E., et al., Forensic application of near-infrared spectroscopy: Aging of bloodstains. Spectroscopy (Santa Monica), 2009, 24(2): 42–48. Boyd, S., et al., Highly sensitive detection of blood by surface enhanced Raman scattering. J Forensic Sci, 2013, 58(3): 753–756. Bremmer, R.H., et al., Remote spectroscopic identification of bloodstains. J Forensic Sci, 2011, 56(6): 1471–1475. Bremmer, R.H., et al., Forensic quest for age determination of bloodstains. Forensic Sci Int, 2012, 216(1–3): 1–11. Budowle, B., et al., The presumptive reagent fluorescein for detection of dilute bloodstains and subsequent STR typing of recovered DNA. J Forensic Sci, 2000, 45(5): 1090–1092. Caldwell, J.P., W. Henderson, and N.D. Kim, ABTS: A safe alternative to DAB for the enhancement of blood fingerprints. J Forensic Sci, 2000, 45(4): 785–794. Cheeseman, R., Direct sensitivity comparison to the fluorescein and luminol bloodstain enhancement tech- niques. J Forensic Ident, 1999, 49(3): 8. Cheeseman, R. and L.A. DiMeo, Fluorescein as a field-worthy latent bloodstain detection system. J Forensic Ident, 1995, 45(6): 1. 241