Final Micro Lab PDF
Document Details
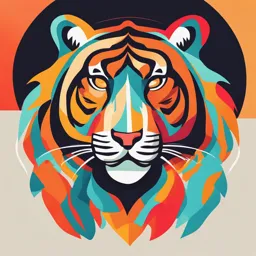
Uploaded by SpellbindingThorium
Faculty of Science, Department of Biological Sciences
Tags
Summary
This document is a laboratory guide for a microbiology course. It covers safety rules, instructions on using a light microscope, and practical procedures. The document describes the parts of a compound light microscope, including objective lenses, eyepieces, and the substage. It also explains handling procedures and techniques for using a microscope and preparing slides.
Full Transcript
Microbiology Laboratory 0490-105 Faculty of Science Department of Biological Sciences Microbiology Program 1 Safety Rules 1. Lab coats must be worn during the lab session. 2. Long hair must be tied back in such a manner as to not be...
Microbiology Laboratory 0490-105 Faculty of Science Department of Biological Sciences Microbiology Program 1 Safety Rules 1. Lab coats must be worn during the lab session. 2. Long hair must be tied back in such a manner as to not be in danger of catching fire. 3. NO MOUTH PIPETTING. 4. Students must know where the safety equipment is located; they will be informed of this at the beginning of the semester by the instructor. 5. No unauthorized or unsupervised experiments are permitted. 6. No eating or drinking is permitted in the lab. 7. Students should wash their hands at the conclusion of every lab. 8. Fingers, pencils and other instruments are to be kept out of the mouth. 9. Students should avoid touching (rubbing) their eyes, mouth or skin before washing of hands. 10. Clean and sterilize your working area before and after working in the lab. 11. Use sterile tools and media. 12. All accidents are to be reported to the lab instructor. 13. Culture or reagent spills are to be reported to and cleaned up only by the instructor. The student responsible for the spill is required to inform the instructor & class members of any such accidents immediately. 14. Cultures, reagent and other materials can not be removed from the laboratory unless specific permission is granted. 15. Any accidents involving the release of BLOOD (e.g. glass cuts etc) must be reported to the lab Instructor immediately. 16. At the end of each lab session, make sure that you have placed all culture tubes and plates in their designated areas. 17. All used cultures, pipettes, tubes, and glass slides must be placed at designated places at the end of each lab session. 18. Make sure that you return the microscope to its proper place after cleaning it. 19. Turn off the gas burners when not in use. 2 Practical (1) The use of Light Microscopy In this Lab session, you will learn: 1. The parts of a compound light microscope, 2. The functions of those parts, and the proper use and care of the microscope. 3. You will also learn the technique of preparing wet-mount slides. 4. Use of oil immersion lens. 5. Use of the stereo microscope. Introduction The light microscope, so called because it employs visible light to illuminate small objects, is probably the commonly used research tool in biology. Most microbial specimens are too small to be seen by the eye alone, thus requires the use of an instrument that magnifies, or visually enlarges, the object. One such instrument, which is of great importance to microbiologists and other scientists, is the bright-field microscope. It is called a bright-field microscope because it forms a dark image against a bright background. Modern microscopes consist of two sets of lenses (objective lenses and ocular lenses, Figure 1), therefore also referred to as a compound light microscope. A compound light microscope consists of a light source or mirror that illuminates, an objective lens that magnifies the image of a specimen, and an eyepiece (ocular lens) that further magnifies the image of the object and projects it into the viewer’s eye (Figure 1.). Specimens, to be observed under a microscope are generally either temporary prepared on slides in the lab or are permanent commercial slides made to last a long time. Temporary slides are made to last only a short time—usually one laboratory period. 3 Compound Light Microscope 1. Care of the Compound Light Microscope The microscope is an expensive precision instrument that requires special care and handling. Always carry the microscope with both hands. Grasp the arm of the microscope with one hand and place your other hand under the base. Always hold the microscope in an upright position so that the eyepiece cannot fall out. Place a microscope on your worktable or desk at least 10 cm from the edge. Position the microscope with the arm facing you. Before you use the microscope, clean the lenses of the objectives and eyepiece with lens paper. Note: To avoid scratching the lenses, never clean or wipe them with anything other than lens paper. Use a new piece of lens paper on each lens you clean. Never touch a lens with your finger. The oils on your skin may attract dust or lint that could scratch the lens. 2. Parts of a Compound Light Microscope Referring to Figure 1. of the compound microscope at the end of this lab, locate the following features of the microscope available in your laboratory; it may have all or most of the features described: A. Ocular Lens The oculars are the lenses you look through. The oculars of most binocular microscopes can be adjusted to match the distance between the eyes of different observers (interpupillary adjustment). Oculars of different microscopes may have different magnifications, although most are 10X. You may have to remove the ocular from its holder to determine its magnification. B. Body Tube Light travels from the objectives through a series of magnifying lenses in the body tube to the ocular. In some microscopes, the body tube is straight; in others, the oculars are held at an angle and the body tube contains a prism that bends the light rays so that they will pass through the oculars. C. Objective Lens Attached to a rotating nosepiece, at the base of the body tube are a group of 3 or 4 Objective lenses or objectives. Rotate the nosepiece and notice that a click is heard as each objective comes into position. The objectives focus the light that comes 4 through the specimen, up the body tube, and through the oculars. Each objective has numbers stamped on it. One of these numbers identifies the magnification of the objective (e.g., 43X). Objectives are usually named according to their magnifying power, as follows: Scanning power - 4X; Low power - 10X; High power - 40X; Oil immersion - 100X. Note the magnification of each of the objectives on your microscope. D. Stage The surface or platform on which you place the microscope slide is the stage. Note the opening (stage aperture) in the center of the stage. On some microscopes, the stage is stationary and has clips to hold the slide in place. On other microscopes, the stage is movable and is called a mechanical stage. Movement is controlled by 2 knobs located on the top, side, or bottom of the stage. Note the horizontal and vertical scales on the mechanical stage. E. Substage The area under the stage, called the substage, may contain a diaphragm, a condenser, or both. i. Diaphragm: regulates the amount of light passing from the light source through the specimen and through the lens system of the microscope. By properly adjusting the diaphragm, you can provide better contrast between the surrounding medium and your specimen, thus greatly improving your image of the specimen. An iris diaphragm consists of a circle of overlapping thin metal plates. The lever that projects from the side of the diaphragm opens and closes these plates, thereby regulating the amount of light that enters the microscope. Most of you have a microscope with an iris diaphragm. ii. Condenser consists of a series of lenses that focus light onto the specimen. It is moved up and down by a knob at its side or by a lever projecting from the condenser housing. By properly adjusting the condenser, you can greatly improve the clarity of the specimen. A filter holder may be attached to the bottom of the condenser. It usually contains a blue filter. F. The Light Source Your microscope has an illuminator built into the base of the microscope and controlled by an on/off switch. You can control the amount of light entering the specimen by adjusting the diaphragm. You can also control the light intensity by adjusting the voltage of a transformer attached to the illuminator. Use low or medium voltage settings for most microscopic observations. You will need a higher setting when using the oil-immersion lens. 5 G. Coarse and fine adjustment knobs You can focus your microscope by using the coarse and fine adjustment knobs these raises or lower either the body tube or the stage, depending on the type of microscope you are using. Try this with the low-power objective in position. Rotate the coarse adjustment knob 1/2 turn clockwise while watching the low-power objective. Do the same with the fine adjustment knob. Figure 1. Parts of a microscope 6 3. The microscope field of view The microscope field of view (FOV) is the diameter of the area when looking through the eyepiece (Figure 2.). The field of view can be changed to observe different areas of the specimen on a slide by moving the stage controller. The FOV is largest at the lowest magnification (scanning objective lens), i.e., more of the specimen will be seen. When a specimen is viewed using a high magnification objective lens, a smaller area of the specimen will be seen, therefore the FOV might need to be changed. Figure 2. The microscope field of view (FOV). As the magnification increases the FOV decreases showing a smaller portion of the specimen. 7 4. Magnification at eye point Notice the numbers engraved on the objectives and on the eyepiece. Each number is followed by an “X” that means “times.” For example, the low-power objective may have the number “10X” on its side. That objective magnifies an object 10 times its normal size). The total magnification of a microscope (magnification at eye point) is calculated by multiplying the magnification of the objective by the magnification of the eyepiece. For example: Magnification X magnification = total of objective of eyepiece magnification 10X X 10X = 100X 5. Parfocality Parfocality refers to objective lenses adjusted so that when used interchangeably, the specimen in view will not be out of focus. Ideally, light microscopes should be parfocal so that it the image in view will remain in relative focus with other objective lenses on the microscope. 6. Image resolution An important feature of the microscope is to produce not only a magnified image but a clear one too. Therefore, the solution power of the objective lenses is important. Resolution power is the ability of an objective lens to distinguish between small objects that are close together. 7. The objective lens working distance The working distance is the distance between an objective lens and the surface of a specimen on a slide when it is in sharp focus. The working distance becomes small with the use of a high magnification objective lens and produces a higher resolving power. 8 8. Light Refraction & the Use of Immersion Oil The most powerful lens of the light microscope is the 100x oil immersion objective. Because light is refracted every time it passes through a medium with a different refractive index, (air to glass or vice versa) the quality of the image is reduced with each passage. Thus, by reducing the number of such passages to a minimum, the clarity, brilliance and resolving power is preserved. Immersion oil has been formulated so that it has a refractive index identical to that of glass. Thus, there is no refraction of light when it passes from glass to oil and vice versa. Thus, two changes in refractive index can be eliminated by placing a drop of immersion oil on the specimen, and immersing the 100x oil immersion objective directly into the drop. You should be struck by the clarity those results. Figure 3. The use of oil immersion. 9 Stereo Microscope Other than the compound microscope, a simpler instrument for low magnification use may also be found in the laboratory. The stereo microscope or dissecting microscope usually has a binocular eyepiece tube, a long working distance, and a range of magnifications typically from 5x to 100x (Figure 4). Some instruments supply lenses for higher magnifications, but there is no improvement in resolution. Such "false magnification" is rarely worth the expense. In a microbiology lab, the stereo microscope is used to examination microbial cultures in Petri dishes and macroscopic specimens. In contrast to a compound light microscope, a stereo microscope provides a 3-D image rather than a 2-D one. Figure 4. Parts of a stereo microscope. 10 Lab activities 1. Cleaning the microscope 2. Preparation of a wet-mount slide of the letter ‘e’ Add a drop of Place the specimen Place the edge of a Slowly lower the water to a slide. in the water. cover slip on the cover slip to prevent slide so that it forming and trapping touches the edge of air bubble the water. 3. Using a Compound Light Microscope A. Focusing 1. Clean the oculars and objectives using lens paper. 2. Put the scanning (4X) objective in position. Hold your slide of the letter “e” so that the letter is in a normal reading position. Then place it on the stage in that position and examine it with the scanning. Also prepare a wet mount slide of unstained salt crystals. 3. Turn on the illuminator and open the diaphragm fully. If there is a condenser, position it as high as it will go, so that the top lens of the condenser unit is level with the stage aperture. Center the specimen over the stage aperture. 4. Position the scanning objective (4X) as close to the slide as possible; then, while looking through the oculars, use the coarse adjustment knob to back off slowly until the specimen comes into focus. 11 5. Use the diaphragm (and/or the transformer voltage regulator) to adjust the light intensity as necessary, and again center the specimen by moving the slide. 6. Switch from the scanning lens (4X) to the low-power objective (10X). Make certain the objective clicks into position. If the specimen stays in focus, your microscope is parfocal. You can sharpen the focus by small adjustments of the fine adjustment knob. If your microscope is not in focus after changing objectives, you may have to use the coarse adjustment knob and then the fine adjustment knob. But remember, do not do this with the high power or oil-immersion objectives in position. Ask your instructor for help if you have difficulty focusing your microscope. Re-center the specimen, adjust the diaphragm, and adjust the position of the condenser to increase the contrast of the specimen. 1. Switch to the high-power (40X) objective and adjust the focus using the fine adjustment knob. Note: These procedures are usually used when examining a wet mount or a commercially prepared micro-scope slide. Always use clean microscope slides. Always proceed from the lowest power to the highest power objectives, making minor focus and light corrections as necessary. Learn to fine-tune your microscope. 12 B. The Microscopic Image i. Orientation of the Image Move the image to the right, left, upward, and downward, and notice the direction in which you have to move the slide. When you want to point out something of interest in the field of vision to someone, you can describe its approximate location by referring to the field of vision as a clock. Thus, you could tell them to “look at 3 o’clock”, or “look just off-center toward 9 o'clock”, and so forth. Alternatively, in some microscopes, a thin black line appears to cut across the field. This is a pointer that has been added to the ocular of your microscope so that you can point out something by moving the object under observation to the end of the pointer. Record your observations in the report sheet. ii. Brightness of the Field of Vision and Working Distance Examine your unstained salt crystals slide, starting with the lowest power objective and progressing to the highest power objective (do NOT use the oil-immersion objective). Note any changes in the brightness of the field when you change objectives. When the object on your slide is in focus, for each objective, the distance between the slide and the objective lens, the working distance, decreases as the objective magnification increases. 4. The use of oil immersion lens 1. Obtain a commercially prepared slide from your lab instructor. 2. Focus your specimen as in section C. 3. Partially rotate the nosepiece so that the space between 40x and 100x objectives is directly over the center of your specimen. 4. Place a small drop of oil on the slide in the center of the lighted area. (Take care not to dribble on the stage.). Note the small drop of oil directly over the area of the specimen to be examined. 5. Rotate nosepiece so that the 100x oil immersion objective touches the oil and clicks into place. Focus only with fine focus. Hopefully, the specimen will come into focus easily. Do not change focus dramatically. If you still have trouble, move the slide slightly left and right, looking for movement in the visual field, and focus on the object which moved. 13 6. Never go back to the 10x or 40x objectives after you have applied oil to the specimen since oil can ruin the lower power objectives. [The 4x objective can be used because it is high enough to be above the oil.] 7. When you have finished for the day, wipe the 100x oil immersion objective carefully with lens paper to remove all oil. Wipe oil from the slide thoroughly. Clean the stage should any oil have spilled on it. 5. Using a Stereo Microscope 1. Place the provided bean in the center of the stage plate. 2. Adjust the eyepiece(s) so that you can look through the microscope comfortably without straining your eyes. 3. Bring your sample into focus. Note: with a stereo microscope you will often be viewing 3-D specimens that have many different levels. You will not be able to focus every feature clearly at the same time. Storage of a light Microscope 1. Clean the optical parts with lens paper and 95% alcohol. 2. Clean the non-optical parts with tissue paper. 3. Place the scanning objective lens into working position. 4. Fold the electrical cord then insert it under the stage. 5. ALWAYS cover the microscope with its dust cover before returning it to its designated place. 14 Lab Discussion 1. Why might it be a good idea to keep your microscope at least 10 cm from the edge of the table? 2. Why should a microscope slide and cover slip be held by their edges? 3. Why do scientists use microscopes? 4. Why should you use lens paper only once? 5. Why is it important to eliminate air bubbles from the slide? 15 Practical (2) Basic laboratory techniques for isolation and cultivation of microorganisms In this Lab session, you will learn: 1. the definition of the terms: pure culture, mixed culture, contamination, and aseptic pure culture techniques. 2. about the tools used in microbiology. 3. about the types of culture media and how to prepare them. 4. aseptic transfer of bacterial cultures. 5. isolation techniques. Introduction Microorganisms are ubiquitous i.e. they are found everywhere, in and on our bodies, on bench tops, in water, soil, food and sewage. The microorganisms in the environment and associated with our bodies are present in a high diversity. When trying to study them from the environment, one quickly discovers that they usually exist in mixed populations. In order to study the morphological and physiological characteristics of an individual microbial species, it is essential that the organism multiplies in number and grows under controlled conditions resulting in a microbial culture. Microorganisms in their natural habitat must be isolated from other microbes, thereby establishing a pure microbial culture. A pure microbial culture is defined as a population containing only a single microbial species or strain. When the microbial culture contains more than one species, it is referred to as a mixed microbial culture. The accidental or unintended introduction of other microbes into the pure microbial culture is known as microbial contamination, thus, the microbial culture is no longer pure. Contamination does not imply that the contaminating organism is harmful; it simply means that the contaminating organism is unwanted in the culture that you are trying to isolate and study. Special techniques, commonly referred to as aseptic pure culture techniques, must be used to obtain and maintain for a pure microbial culture to study. The growth of fungal and bacterial cells on an agar plate generates colonies. A colony is a visible mass of microorganisms growing on the surface an agar medium and usually originating from a single organism or arrangement of organisms. Before learning the techniques involved in manipulating microbial cultures, it is first necessary to become familiar with the tools, equipment and cultivation material used in a microbiology lab: Inoculating loops and needles These tools are traditionally made from nichrome or platinum with a heat insulating handle (Figure 1A). The inoculating loop is used to handle bacteria or yeasts, while the inoculating needle is used for filamentous fungi. The tools are used to pick up and transfer a small sample from a microbial culture, referred to as an inoculum. These tools should be sterilized before they are introduced in a microbial culture. Sterilization is a process that destroys all living cells, spores and biological entities. The tools must be sterilized between uses by flaming the wire part (incineration of the microorganisms) by using a Bunsen burner (Figure 1B). To flame an inoculating loop, hold the loop so that the wire part of the loop (not the handle) glows red hot. All of the loop should be flamed before sterilization is considered complete. The inoculating needle is usually composed of a thick wire that cannot glow red easily, therefore, placing the wire in the flame for 10 seconds will achieve sterilization. Figure 1. A. Inoculating loop and needle. B sterilization of a loop. Culture media (sg. medium) A general term referring to the substances upon which a microorganism is grown, transported and stored. The, culture media must contains all the nutrients required for the growth of the microorganism. Ideally, microbiological media are designed to imitate the environment in which the organism naturally grows. Culture media can be classified based on their physical nature, chemical composition and function. Physical types of culture media Microbiological media may be prepared as liquid (broth), solid or semisolid media. When a solid medium is prepared, the corresponding broth is solidified by the addition of agar to the broth. Agar is a polysaccharide found in the cell walls of some algae; it is inert and degraded by very few microorganisms. Moreover, it is an ideal solidifying agent for microbiological media because it melts at 100 oC and solidifies at ~ 45-50oC. A solid medium requires an agar concentration of about 1.5%, whereas a semi-solid medium contains agar at a concentration less than 1%. Solid media are specifically important for isolating microbes from each other to obtain pure cultures. Chemical composition of culture media A medium in which the exact amount of pure chemicals used to formulate the medium is known as a chemically defined medium (synthetic medium, Table 1). Synthetic media are usually used to culture microorganisms with specific nutritional requirements. Media that are composed of a mixture of proteins and extracts in which the exact chemical composition of some ingredients is unknown are complex media. Complex media are useful because they meet the nutritional requirements of different microorganisms. Function of culture media In addition to the distinction between chemically defined and complex, microbiological media may be further categorized as general-purpose, enriched, selective, and differential. A general-purpose medium supports the growth of many microorganisms. Two examples include nutrient agar, which allows the growth of many bacteria, and potato dextrose agar, which supports the growth of many fungi. An enrichment medium contains some important growth factors (e.g. vitamins, amino acids, blood components, or carbon sources) necessary for the growth of fastidious microbes (microorganisms with complex nutritional requirements). Selective media allow for the selection of particular microorganisms that may be present in a mixed culture (Table 2). Selective media usually contain a component that enhances the growth of the desired organism while inhibiting that of competing organisms. Differential media allow the separation of organisms based on observable changes in the appearance of the media. Any single medium may be a combination of the above categories. Table 1. Example of a chemically defined medium and a complex medium. Chemically defined medium Complex medium Minimal broth medium Nutrient agar Glucose 10.0 g Beef extract 1.5 g K2HPO4 2.5 g Yeast extract 3.0 g (NH4)2HPO4 1.0 g Peptone 6.0 g MgSO4 7H2O 0.20 g Glucose 1.0 g FeSO4 7H2O 0.01 g Agar 15.0 g MnSO4 7H2O 0.007 g water 1000 ml, pH 6.6 water 985 ml, pH 7.0 Table 2. Examples of media general-purpose, enrichment, selective and differential media. Functional type Medium General-purpose media Nutrient agar and potato dextrose agar Enrichment media Blood agar Selective media MacConkey agar Differential media Eosin methylene blue Autoclave Culture media are sterilized by a device called an autoclave (Figure 2). The media are prepared in glass bottles, flasks or tubes and then placed in the autoclave where it is sterilized by heat at 121°C for 15 to 20 minutes, which generates steam and high pressure. The autoclave is also used to sterilize glassware and heat-stable material. Figure 2. The Autoclave. A device used for the sterilization of culture media, glassware and heat stable material under heat and pressure. Petri dishes (plates) The autoclave-sterilized media containing agar are left to cool down so that they can be tolerated by human hands. The molten media are then poured into sterile round dishes with a cover; these are known as Petri dishes. Once the medium solidifies in the Petri dish, microbes can be introduced on the surface of the culture medium to utilize the nutrients and grow (Figure 3). Figure 3. A Petri dish. A shallow container with a lid used to hold solid culture media. Incubator Each group of microorganisms can grow optimally at a specific temperature. Once a culture medium, whether broth or solid, is inoculated with a microorganism, it is then placed in an incubator (Figure 4). An incubator is a device which provides the suitable temperature for microbial growth. Figure 4. An incubator. A device used to grow and maintain microorganisms by providing an optimum temperature. Aseptic techniques When working with microbial cultures, it is essential that you use proper aseptic culture techniques. Aseptic techniques are applied for the manipulation of microorganisms such that contamination by undesirable organisms is prevented to maintain a pure culture. Aseptic techniques not only protect a laboratory culture from becoming contaminated, but also protect the worker and the environment from becoming contaminated by the microorganisms. You should be able to perform standard aseptic techniques with ease and precision so that isolation and cultivation of microorganisms in pure culture may be accomplished. The following are common aseptic practices applied in a microbiology lab: Cleaning the work area with Dettol or 70% alcohol (dispose tissue in an autoclave bag) Working near the Bunsen burner flame Using sterile loops and needles for microbial inoculation Work away from open windows and doors Isolation of Pure Cultures from Mixed Cultures Several different methods for getting a pure culture from a mixed culture are available. These include: 1. Streak plate technique (four-way streak) 2. Pour plate technique (will be discussed in lab 8) 3. Spread technique (will be discussed in lab 8) In this laboratory, you will be learning the streak plate technique. During the streak plate technique, an inoculum is applied to the surface of an agar plate. By streaking the inoculum across the surface of the agar, a spatial separation of cells is achieved. Theoretically, a single separated cell will reproduce, forming a visible mass of microbial cells. Therefore, all members of a colony are descendants of that original cell. For economy of materials and time, the streak plate method is best. It requires some skill to achieve a plate that reveals well-isolated colonies; however, good technique is forthcoming with experience and practice. A properly executed streak plate technique should achieve good spacing between the colonies. The isolated colonies can then be transferred by an inoculating loop to a sterile medium, a process known as sub- culturing. Lab activities Before each experiment the Petri dishes and tubes must be labelled correctly. Proper labeling will remind you of which microorganism was inoculated and under which conditions. It will also avoid the accidental exchange of plates and tubes between groups or individuals. How to properly label Petri dishes and tubes The following information should be on the Petri dishes and tubes: a. Your name or group no. b. The date c. Name of medium d. The incubation temperature e. The name of the microorganism (e.g. E. coli) f. Course no. and section The Petri dishes should be labeled at the back side of the plate and NOT ON THE LID (Figure 5). The writing should be at the edge of the plate and NOT IN THE MIDDLE. Figure 5. Labeling of a Petri dish. The tubes should be labeled horizontally around the top part of the tube and NOT VERTICALLY (Figure 6). Figure 6. Labeling of a tube. A. Aseptic transfer from a broth culture to a broth medium Broth cultures are useful because very high cell densities can be achieved. Also, some characteristics like oxygen requirements can be observed. Handling the broth tube requires specific steps to be followed (Figure 7). 1. Grasp both the stock culture and the sterile medium in one hand and the inoculating loop in the other. a. Loosen, but don’t remove the caps on the tubes. b. Hold the inoculating loop at the end of the rubber/plastic portion of the handle. 2. Stick the loop into the flame until it becomes RED HOT. Flame the entire wire loop and about half of the metal portion of the handle (because part of the metal portion will extend into the test tubes). 3. Remove the caps and hold them in the same hand as the loop. a. To avoid contamination of the caps: i. don’t set them down on the bench top; ii. don’t touch the bottom (opening) of the caps; iii. try to keep the caps pointed downwards (so that airborne contaminants don’t fall into them). 4. Flame the mouths of the test tubes. The mouths may be contaminated. We don’t want to drag the loop across the mouth of a tube and contaminate our culture. 5. Cool the loop. Cooling is essential. 6. Insert the loop into the stock culture to obtain a small number of cells. You don’t need to see cells on the loop to have enough. 7. Inoculate a broth medium, making sure to introduce only the sterilized area of the loop wire. 8. Re-flame the mouths of the tubes, and then put the caps back on. 9. Re-flame the inoculating loop. Figure 7. Steps of inoculating a solid media or a broth culture. Notice that the source of the inoculum is a broth culture. 2. Isolation of pure cultures from mixed cultures B. Isolation of a pure bacterial culture from a mixed broth culture (4- way Streak plate inoculation) Broth cultures are problematic because it is difficult to determine if contamination has occurred (because all the cells mix together in a broth). Therefore, the streak plate technique of a broth culture inoculum is performed to check the purity (Figure 8). 1. Label your plate. 2. Flame the loop and wire and streak a loopful of broth as at A in the diagram. 3. Re-flame the loop and cool it. 4. Streak as at B spreading a small portion of section A onto section B. 5. Re-flame the loop and cool it. 6. Streak as at C. 7. Re-flame the loop and cool it. 8. Streak as at D. 9. Sterilize the loop again. 10. Replace the lid and invert the plate. Incubate the plate. 11. Label the plate and incubate it inverted. Figure 8. Streak plate technique C. Isolation of single colonies from a mixed culture plate When maintaining a pure microbial culture, poor aseptic conditions might lead to contamination resulting in a mixed culture of different organisms. We have already previewed the use of an inoculating loop in inoculating bacteria. In this activity, you will also practice using an inoculating needle to purify a filamentous fungal colony from a mixed culture. i. Isolation of bacterial colonies Take an inoculum from a well-isolated single colony from the mixed culture plate using a loop. Repeat the streak plate technique described in activity 2. ii. Isolation of fungal colonies TAKE CARE WHEN OPENING A FUNGAL CULTURE PLATE. The Petri dish should be open slightly and NOT FULLY OPEN TO AVOID SPORE DISPERSAL. 1. Label your plate. 2. Flame the needle for 10 seconds then wait for the needle to cool down. 3. Open the plate just enough to take from the fungal colony. 4. Obtain a small amount of fungal filaments by going down to the base of the filaments, taking a small portion of the agar. 5. Place the inoculum in the center of a potato dextrose agar plate. 6. Incubate the plate at 25ºC. DO NOT INVERT THE PLATE. Lab Discussion 1. Can a pure culture be prepared from a mixed broth culture? Explain 2. Explain why the following steps are essential during subculturing: a. Flaming the inoculating tool before and after each inoculation. b. Cooling the inoculating tool before obtaining the inocula. 3. How can you Isolate two different colonies that are attached together? Practical (3) Bacteria I In this lab session you will learn: 1. the terms used to describe the appearance of bacterial colonies on agar plates. 2. the terms used to describe the growth patterns of bacteria in a broth culture. 3. about the different morphological characteristics of bacteria (cell shape and arrangement). 4. how to examine bacterial motility. 5. the characteristic features of cyanobacteria. Introduction The five-kingdom classification scheme proposed by Whittaker grouped prokaryotes (“pre-nucleus”) such as bacteria in a single kingdom, Monera. Currently, organisms are grouped into three domains based on the sequences of the ribosomal RNA (rRNA) (Figure 1.). In this classification scheme, prokaryotes are placed in either the domain Bacteria or Archaea. The prokaryotes that you will examine in this laboratory are classified in the Domain Bacteria. Figure 1. The three domains classification system of organisms. Bacteria are relatively small (1 – 10 μm) and simple, single-celled organisms that have existed on earth for over 3.5 billion years. These prokaryotes most-likely have the greatest distribution of all organisms. They are found almost everywhere from the soil to the air and even water. In these various habitats, some bacteria can withstand extreme temperatures and chemically harsh environments. Bacteria have developed numerous modes of nutrition. They can generally be classified as either an autotroph, using CO2 as a carbon source or a heterotroph, using organic carbon. These two nutritional modes can be further subcategorized based upon whether they use light, inorganic or organic compounds. Most prokaryotes, however, are heterotrophic in that they obtain nutrients from their surrounding environment. Photosynthetic bacteria are grouped within the Phylum Cyanobacteria. The bacteria were once classified as "blue green algae" because of their superficial resemblance to eukaryotic green algae. Although both groups are photosynthetic, they are only distantly related. Despite the presence of chlorophyll a as in green plants, cyanobacteria lack internal organelles and a discrete nucleus. Moreover, their cell walls contain peptidoglycan. Cultural characteristics of Bacteria In natural environments, microorganisms usually exist as mixed populations. However, if we are to study, characterize, and identify microorganisms, we must have the organisms in the form of a pure culture. Techniques for obtaining pure cultures from a mixed population were described in Lab 2. Microorganisms may show distinguishing gross morphologies when cultured on different media. This macroscopic appearance of bacteria (characteristic growth patterns which can be observed with the naked eye) is often used in their identification. Colony Morphology and Pigmentation A colony is a visible mass of microorganisms growing on the surface an agar medium and usually originating from a single organism or arrangement of organisms. Different microorganisms will frequently produce colonies that differ in their morphological appearance. Single colonies can be examined under the stereo microscope and described using standard terms (Figure 2). Probably the most visual characteristic is pigmentation (color). Some microorganisms produce pigments during growth and are said to be chromogenic. Often, however, formation of pigments depends on environmental factors, such as temperature, nutrients, pH and moisture. For example, Serratia marcescens produces a deep red pigment at 25°C, but does not produce this pigment at 37°C. Figure 2. Cultural characteristics of bacterial colonies on agar plates Bacterial Growth Patterns in Broth Media Bacteria may form different growth patterns in broth media. These patterns are described as the distribution and appearance of the growth using the following terms: a. Turbid –the medium is cloudy. b. Pellicle – thick, pad-like growth on the surface. c. Sediment – concentration of growth at the bottom of the broth culture. Figure 3. Bacterial growth in broth media Microscopic morphology Despite the simplicity and small size of bacteria, they differ in shape and size (Figure 4.). Bacteria are so small that the highest magnification of the ordinary compound microscope must be used to study their shape. The two most common shapes are cocci (spherical cells) and bacilli (rod-shaped cells). There are less common shapes of bacteria, such as spirilla (spiral-shaped cells) and vibrios (comma-shaped cells). Cocci and bacilli cell shapes can exist singly or can be of a characteristic arrangement (Figure 5.) Figure 4. Bacterial cell shapes Figure 5. Bacterial cell arrangements Bacterial Motility Many bacteria are motile (able to move under their own power). Most motile bacteria drive themselves by special organelles termed flagella. The bacterial flagellum is a non- contractile, semi-rigid, and helical tube composed of protein and anchors to the bacterial cytoplasmic membrane and cell well by means of disk-like structures. The flagella can be present in different numbers arranged differently on the cell’s surface (Figure 6.). Motile bacteria exhibit taxis, which is the movement towards and attractant or away from a repellent to keep the cells in an optimal environment. Under the microscope, motile bacteria seem to move in a decisive way, though they may frequently change direction. However, the motion which causes most problems is Brownian motion, first observed in 1827 by the English botanist Robert Brown. This phenomenon is due to random molecular bombardment of tiny bacterial cells with the molecules of the solvent. A microbiologist needs to learn how to distinguish the effects of Brownian motion from true bacterial motility. Figure 6. Arrangement of bacterial flagella Cyanobacteria Cyanobacteria have an elaborate and highly organized system of internal membranes that function in photosynthesis. They have the photosynthetic pigment chlorophyll a and several accessory pigments. They may be single-celled or colonial, depending on the species and environmental conditions. Vegetative cells are the normal, photosynthetic cells formed under favorable growing conditions. Some filamentous cyanobacteria have heterocysts, which are large, transparent, and thick-walled cells that contain enzymes for atmospheric nitrogen fixation. Cells develop heterocysts for nitrogen gas fixation when the environment is deprived of nitrogen and ammonia (the preferred nitrogen source). The fixed nitrogen is then employed as a nitrogen source for the construction of protein and nucleic acids. Unlike some cyanobacteria, plants do not have the ability to fix atmospheric nitrogen. Nitrogen-fixing cyanobacteria contribute greatly to the health of the rice paddies of Asia, which feed about 75% of the world's human population. In this lab we will study the following representatives of cyanobacteria: Domain Bacteria Phylum Cyanobacteria 1. Anabaena sp.: A chain of cocci with intercalary heterocysts. Contain akinetes which are resting cells resistant to desiccation. These dormant cells later germinate under favourable conditions to form new filaments. 2. Oscillatoria sp.: Long series of rectangular cells composing a filament. 3. Chroococcus sp.: Large cocci cells grouped in aggregates of 2-4 cells. Lab activities A. Examination of colony morphology and pigmentation Using the terms in Figure 2., describe the growth characteristics of the provided agar plates. Use a microscope to magnify the colony. B. Examination growth in broth cultures Using the terms in Figure 3., describe the growth characteristics of the provided broth culture tubes. C. Examination of bacterial cell shape and arrangement Examine the provided commercially-prepared slides under the 100X objective lens. Using Figures 4 & 5, draw and name the various shapes and arrangements observed. Draw what you see under the microscope and DO NOT COPY THE IMAGE FROM THE MANUAL. Drawing should always be in pencil. The first letter of the genus name should be capitalized. The genus and species names should be underlined if written by hand or written in italics if typed. E.g., Anabaena aequalis D. Examination of bacterial motility using a wet-mount technique 1. From a broth culture: Place one drop of the culture in the middle of the slide. From an agar plate: Place a small drop of water in the center of a slide. Transfer a small portion of a single colony to the drop with a loop and suspend (be certain to allow the loop to cool before picking up the specimen). 2. Lower a clean cover slip over the drop as though it were hinged at one side. 3. First focus with the 4x objective on the edge of the coverslip. It is easier to find and focus on the nearly transparent suspension. 4. Find a bubble in the liquid suspension, and adjust the fine focus on the edge of the bubble. 5. Switch to the 10x objective. Repeat the careful focusing. 6. Switch to the 40x objective. Repeat the careful focusing. You should be able to distinguish bacteria at this power (magnification = 400x). 7. Partially rotate the nosepiece so that the 40x and 100x objectives are on both sides of the specimen. Place a small drop of oil on the slide in the center of the lighted area. 8. Rotate the turret so that the 100x oil immersion objective touches the oil and clicks into place. 9. Focus only with the fine focus. Hopefully, the specimen will come into focus easily. Do not change focus dramatically. If you still have trouble, move the slide slightly left and right, looking for movement in the visual field, and focus on the object which moved. 10. At 1000x, maximize the depth of field by narrowing the iris diaphragm, and adjust the focus so that most bacteria are in focus. (Because of the depth of the water, not all bacteria will be in focus at a given point.) 11. Describe the type of motility you observe as true motility or Brownian motion. E. Examination of commercial slides of Cyanobacteria species Examine the specimens under the microscope and draw the species using a pencil. Write the species’ scientific name and label the distinctive features. Lab Discussion 1. Define the following terms: pure culture, inoculum, aseptic technique, and colony. 2. What is the difference between macroscopic and microscopic appearance of bacteria? 3. State the three standard terms used to describe single colonies on agar plates. 4. State and define the three types of growth that may be seen in a broth culture. 5. State three basic shapes of bacteria. 6. State and describe the different arrangements of cocci. 7. What is the difference between true motility and Brownian motion? Practical (4) Bacteria II In this lab session you will learn: 1. the chemical and theoretical bases of basic biological staining. 2. the procedures for preparing bacterial smears. 3. procedures for simple staining. 4. the chemical basis of the Gram stain. 5. the method for performing the Gram stain. Introduction Bacterial morphology (cell shape) may be examined by observing living unstained organisms (wet mount) or observing killed, stained organisms. Since bacteria are almost colorless and therefore show little contrast with the broth in which they are suspended, they are difficult to observe when unstained. Staining microorganisms enables one to see greater contrast between the organism and the background. Therefore, bacterial shape and arrangement can easily be observed under the microscope if the cells are stained. Certain staining procedures may be applied to highlight bacterial structures, such as flagella, capsules and endospores or differentiate between two bacterial groups e.g. Gram-positive and Gram-negative bacteria. Stains are generally salts in which one of the ions is colored. A salt is a compound composed of a positively charged ion and a negatively charged ion. For example, the dye methylene blue is actually the salt methylene blue chloride, which dissociates in water into a positively charged methylene blue ion that is blue in color and a negatively charged chloride ion that is colorless. Dyes or stains may be divided into two classes: basic and acidic (Figure 1). If the color portion of the dye resides in the positive ion, as in the above case, it is called a basic dye (examples: methylene blue, crystal violet, safranin). If the color portion is in the negatively charged ion, it is called an acidic dye (examples: nigrosin, Congo red). Figure 1. Classes of dyes. The cytoplasm of all bacterial cells has a slight negative charge when grown in a medium of near neutral pH, due to its chemical nature. Therefore, when using a basic dye, the positively charged color portion of the stain combines with the negatively charged bacterial cytoplasm (opposite charges attract) and the organism becomes directly stained (Figure 1). An acidic dye, due to its chemical nature, reacts differently. Since the color portion of the dye is on the negative ion, it will not combine readily with the negatively charged bacterial cytoplasm (like charges repel). Instead, it forms a deposit around the organism, leaving the organism itself colorless. Since the organism is seen indirectly, this type of staining is called indirect or negative, and is used to get a more accurate view of bacterial size, shapes, and arrangements. Fixation of cells (bacterial cell smear) Before starting most bacterial staining methods, the cells must be fixed by heat or a chemical on a glass slide. The purpose of fixation is to preserve the internal and external structures of a specimen and fix its position on a slide. The process inactivates enzymes that might disrupt cell morphology and toughens cell structures so that they do not change during the staining procedure. Heat fixation is commonly used to fix bacteria, where gentle heat is applied to a film of cells (a smear) on a slide. Heat fixation of a specimen starts with adding a drop of cells suspended in liquid and spreading it on a slide. After air drying the liquid, a whitish layer smear should appear on the slide, which is then passes through the flame. The heat coagulates the organisms' proteins, causing the bacteria to adhere to the slide. Staining techniques Simple staining In order to observe bacterial cell size, shape, and arrangement, a simple staining method can be applied in which a single dye is used. A heat-fixed smear is simply covered with a stain for a short time, excess stain is washed off with water, and the slide is blotted dry. Basic dyes such as crystal violet, methylene blue and carbolfuchsin are commonly used in simple staining. Differential staining A staining procedure that distinguishes organisms based on their staining properties is referred to as differential staining. The staining produced may be used to differentiate bacteria into two groups. For example, Gram staining divides most bacteria into two groups: Gram-positive and Gram-negative. Acid-fast staining is another important differential staining procedure used to identify the pathogenic bacterium Mycobacterium tuberculosis. The staining method divides bacteria into two groups: acid-fast and non-acid-fast. Many differential staining procedures are applied to detect the presence of a specific structure, such as a flagellum, endospore, or capsule (also known as structural staining). Gram stain The Gram stain is the most widely used staining procedure in bacteriology. It is called a differential stain because it differentiates between Gram-positive and Gram-negative bacteria. Bacteria that stain purple with the Gram staining procedure are termed Gram-positive and those that stain reddish pink are said to be Gram-negative. The terms positive and negative have nothing to do with electrical charge, but simply designate two distinct morphological groups of bacteria. Gram-positive and Gram-negative bacteria stain differently because of fundamental differences in the structure of their cell walls (Figure 2 A & B). The bacterial cell wall serves to give the organism its distinctive shape as well as to prevent osmotic lysis. The main difference between Gram-positive and Gram- negative bacteria is the thickness of the cell wall peptidoglycan (Figure 2 A & B). Figure 2. A. Gram-positive cell wall. B. Gram-negative cell wall. The Gram staining procedure involves four basic steps: 1. Primary stain - crystal violet for one minute. All bacteria take up this dye and appear purple. Rinse off the excess dye with water. 2. Mordant - Gram's iodine is added to interact for one minute with the crystal violet. Excess iodine is rinsed off with water. 3. Decolorization - 95% ethanol is briefly (10-20 seconds) applied to the smear followed by a water rinse. 4. Counterstain - Safranin is added for 45 seconds to dye any decolorized cells. It does not change the color of the cells that retain the crystal violet. During the staining procedure, the crystal violet and iodine combine to form a larger molecule that precipitates out within the cell of Gram-positive bacteria. The alcohol/acetone mixture then causes dehydration of the multilayered peptidoglycan, thus decreasing the pores between the molecules and causing the cell wall to trap the crystal violet-iodine complex within the cell. In the case of Gram-negative bacteria, the alcohol/acetone mixture, being a lipid solvent, dissolves the outer membrane of the cell wall and may also damage the cytoplasmic membrane to which the peptidoglycan is attached. The single thin layer of peptidoglycan is unable to retain the crystal violet-iodine complex and the cell is therefore decolorized. There are several factors that could result in a Gram-positive organism staining Gram-negatively: 1. The method and techniques used. Overheating during heat fixation, over decolorization with alcohol, and even too much washing with water between steps may result in Gram-positive bacteria losing the crystal violet-iodine complex. 2. The age of the culture. Cultures more than 24 hours old may lose their ability to retain the crystal violet-iodine complex. 3. The organism itself. Some Gram-positive bacteria are more able to retain the crystal violet- iodine complex than others. Therefore, one must use very precise techniques in Gram staining and interpret the results with discretion. Lab activities All activities to be done individually A. Preparation of bacterial smears Agar medium culture 1. Using the dropper bottle of distilled water found near your staining rack, place 1/2 of typical sized drop of water on a clean slide by touching the dropper to the slide (Figure 3). 2. Aseptically take a small inoculum of the culture from the agar surface using a sterile loop and just touch the drop of water 2 - 3 times until it just turns cloudy. 3. Burn the remaining bacteria off of the loop. If too much culture is added to the water, you will not see stained individual bacteria. 4. After the loop cools, spread the suspension over the entire slide to form a thin film. 5. Allow this thin suspension to completely air dry. 6. To heat-fix the bacteria to the slide, pass about one inch of the slide (film-side up) through the flame of the Bunsen burner as many times as it takes until the slide just becomes uncomfortable to hold. Broth medium culture 1. Aseptically place 2 or 3 loopfuls of the culture on a clean slide (Figure 3). Do not use water. 2. Using the loop, spread the suspension over the entire slide to form a thin film. 3. Allow this thin suspension to completely air dry. 4. Pass the slide (film-side up) through the flame of the Bunsen burner 3 or 4 times to heat-fix. Figure 3. Preparation of a bacterial smear from an agar culture or a broth culture. B. Simple staining 1. Place the heat-fixed slide on a staining tray and cover the entire film with safranin. Stain for one minute. 2. Pick up the slide by one end and hold it at an angle over the staining tray. Using the wash bottle on the bench top, gently wash off the excess safranin from the slide. Also, wash off any stain that gets on the bottom of the slide. 3. Use a blotting paper to blot the slide dry. Observe using oil immersion microscopy. 4. Draw the cells and record the cells’ shape and arrangement. C. Gram Stain 1. Prepare a smear of your bacteria on a clean glass slide as described in section (A). 2. Stain with crystal violet for one minute (Figure 4). Gently wash with water. Shake off the excess water but do not blot dry between steps. 3. Stain with Gram's iodine solution for one minute and gently wash with water. 4. Decolorize by adding 95% ethanol for 20 seconds. Wash immediately with water. 5. Stain with safranin for 45 seconds and wash with water. 6. Blot dry and observe using a light microscope reaching to oil immersion lens (review the focusing steps from Lab 1). 7. Draw the cells and record the cell shape, arrangement, color and Gram reaction i.e. state whether the cells are Gram-positive or Gram-negative Figure 4. Gram staining procedure. Lab Discussion Simple Stain: 1. Describe the procedure for heat fixation. 2. Define the following: acidic dye, basic dye, direct stain. 3. State the chemical and physical principles of direct staining. Gram Stain 1. State why the Gram stain is said to be a differential stain. 2. Describe the differences between a Gram-positive and a Gram-negative cell wall. 3. Describe a theory as to why Gram-positive bacteria retain the crystal violet-iodine complex while Gram-negative bacteria become decolorized. 4. Describe three conditions that may result in a Gram-positive organism staining Gram-negatively. Practical (5) The Protists In this lab session you will learn: 1. The microbial diversity in the Protists group (concentrating on microalgae and protozoa) 2. The various morphological and distinctive features used in their identification. Introduction The protitsts are a diverse collection of eukaryotic organisms including algae and protozoa classified under the domain eukarya. They lack highly organized tissue found in animals and plants. Algae includes photosynthetic organisms which possess a cell wall, they may be microscopic i.e., microalgae or can be seen by the naked eye i.e., macroalgae. Whereas, protozoa refer to unicellular eukaryotes that obtain their nutrients through chemoheterotrophy i.e., use organic source of nutrients. A genus may differ morphologically when it is in the vegetative stage i.e., actively growing cells or in the reproductive stage. They are commonly found free living inhabiting aquatic environments and may also be living in terrestrial habitats. These organisms were once classified under the Kingdom Protista; however, some scientists still use classification term. The protists are now classified based on morphological, biochemical and phylogenetic analysis. The latest classification scheme divides the protists into the four supergroups; Excavata, Amoebozoa, Stramenopiles, Alveolates, and Rhizaria (SAR), and Archaeplastida (Figure 1). Figure 1. The supergroups of the protists. Microalgae Microalgae include a diverse group of photosynthetic, heterotrophic organisms which have an extraordinary potential for cultivation as energy crops. The organisms can exist as unicellular, colonial or filamentous form. They are ubiquitously distributed throughout the biosphere and grow under the widest possible variety of conditions. They live in moist habitats, black earth, in the desert sands and in all the conditions in between. In this lab we will study the following representatives of micro-algae: Domain: Eukarya Kingdom: Protista Supergroup: SAR 1. Diatoms Diatoms are unicellular group of microalgae (Figure 2 A and B). They possess chlorophyll, thus are able to photosynthesize and the majority of diatoms fix carbon dioxide. The organisms have a distinctive, two-piece cell wall of silica called frustule. Different genera of the group vary in shape and ornamentation, and they are of two forms, pennate and centric (Figure 1 A and B). Figure 2. A. Pennate diatom. B. Centric diatom. Domain: Eukarya Kingdom: Protista Supergroup: Archaeplastida 2. Chlamydomonas sp. The vegetative form of Chlamydomonos spp. are unicellular, spherical or oval in shape with a large chloroplast (Figure 3). They possess two flagella of equal length. The organism reproduces asexually though the production of flagellated motile spores known as zoospores by mitotic division. During sexual reproduction, two cells act as gametes fuse and enters a resting phase before meiosis occurs. Figure 3. A. Chlamydomonas sp. B. Microscopic image of Chlamydomonas sp. image. Domain: Eukarya Kingdom: Protista Supergroup: Archaeplastida 3. Volvox sp. Volvox sp. is a motile green algal colony composed of 500-50,000 Chlamydomonas- like cells arranged along the periphery of a hollow mucilaginous sphere connected by cytoplasmic strands (Figure 4A). The spaces between the cells are filled with water and mucilage. During asexual reproduction, daughter colonies are formed with in a mother colony (Figure 4B). Eventually colony ruptures releasing the daughter colonies which mature into mother colonies. Sexual reproduction, involves mating between female and male gametes (reproductive cells) leading to the formation zygospores (resting cells arising from mating between gametes) in a mother colony (Figure 4C). Figure 4. A. Vegetative state of Volvox sp. B. Asexual state of volvox sp. C. Sexual state of volvox sp. Domain: Eukarya Kingdom: Protista Supergroup: Archaeplastida 4. Spirogyra sp. A filamentous algae of cylindrical cells containing a large central vacuole, with the nucleus suspend by cytoplasmic threads (Figure 5A). The distinctive feature of the organism is the spiral band-like chloroplasts. Asexual reproduction in Spirogyra sp. takes place by means of fragmentation of the filaments (Figure 5B). Sexual reproduction occurs by conjugation of gametes of different mating type produced in gametangia (organs or cells in which gametes are produced). Conjugation is of two types; lateral and scalariform. Lateral conjugation takes place between two adjacent cells of the same filament connected by a conjugation tube (Figure 5C). Whereas scalariform conjugation occurs between two filaments (Figure 5D). After conjugation, the gametangia will be empty and zygospore is formed within a zygosporangia (structure in which zygospores are produced). Figure 5. A. Vegetative state of Spirogyra sp. B. Asexual state of Spirogyra sp. C. Lateral conjugation of Spirogyra sp. D. Scalariform conjugation of Spirogyra sp. Domain: Eukarya Kingdom: Protista Supergroup: Archaeplastida 5. Vaucheria sp. Photosynthetic, multinucleate tubular branches lacking cross walls except in association with reproductive organs or an injury (Figure 6A). Vaucheria sp. Reproduces asexually by the production of zoospores or non-motile aplanospores (Figure 6B). These spores will germinate under favourable conditions to generate the vegetative state of the organism. Sexual reproduction is through the generation of an antheridium (male sex organ) and an oogonium (female sex organ). The antheridium is a short lateral branch with a curved apex which produces motile antherozoids. The antherozoids fertilizes the egg in the oogonium forming an oospore (zygote) (Figure 6C). Figure 6. A. Vegetative state of Vaucheria sp. B. Asexual state of Vaucheria sp., zoospores and aplanospores C. Sexual reproduction of Vaucheria sp. Domain: Eukarya Kingdom: Protista Supergroup: Excavata 6. Euglena sp. Unicellular flagellated organisms that have plant and animal characteristics (Figure 7). Most Euglena spp. have chloroplasts and can make their own food by photosynthesis. However, they are not completely autotrophic, euglena spp. can also absorb food from their environment. The organism is unique in that it is both heterotrophic (must consume food) and autotrophic (can make its own food). The possess an eyespot called a stigma which helps in positing the organisms to light. The contractile vacuole is responsible for osmoregulation. Euglena spp. usually lives in quiet ponds or puddles or fresh water of organic rich brackish water. Figure 7. Euglena sp. Protozoa Protozoa are single-celled eukaryotes that commonly show characteristics associated with animals, most notably motility and heterotrophy. Most protozoans are too small to be seen with the naked eye of size 0.01-0.05 mm. Organisms up to 0.5 mm in size are fairly common and can easily be seen under the microscope. Protozoa have traditionally been grouped on the basis of locomotion. Motility in protozoa is by means of pseudopodia (false feet), flagella or cilia. In this lab we will study the following representatives of Protozoa: Domain: Eukarya Kingdom: Protista Supergroup: Amoeboza 1. Amoeba sp. A genus of protozoa that moves by means of temporary projections called pseudopodia, and is well-known as a representative unicellular organism (Figure 8). The organism has a food vacuole containing several enzymes for the digestion of food, in addition to a contractile vacuole. The cytoplasm is divided into a gelatinous outer ectoplasm, and an inner fluid endoplasm. Figure 8. Amoeba sp. Domain: Eukarya Kingdom: Protista Supergroup: Excavata 2. Trypanosoma sp. The genus Trypanosoma contains a large number of parasitic species which infect wild and domesticated animals and humans in Africa (Figure 9). Certain species are transmitted to humans by the blood-sucking tsetse fly and causes the African sleeping sickness disease. The organism is undulating in shape with a flagellum. Figure 9. Trypanosoma sp. Domain: Eukarya Kingdom: Protista Supergroup: SAR 3. Paramecium sp. A unicellular organism about 0.02 inches long, that employs short hair-like projections known as cilia for motility (Figure 10). A disinvite feature of the organism is the presence of a large macronucleus for non-reproductive cell function and a smaller micronucleus contains genetic material passed from one generation to the other. When Paramecium sp. encounters a negative stimulus, it is capable of rotating up to 360 degrees as an avoidance behavior to find an escape route. Figure 10. Paramecium sp. Domain: Eukarya Kingdom: Protista Supergroup: SAR 4. Plasmodium sp. Unicellular non-motile, obligate parasites of humans, animals and insects (Figure 11). Certain species of the genus infect red blood cells through transmittance by mosquitoes which cause the disease malaria. Figure 11. Plasmodium sp. Practical (6) The kingdom Fungi In this lab session you will learn: 1. Microscopic examination of Yeast using Wet mount. 2. Cultural characteristics of Yeasts on agar plate 3. Isolation of Pathogenic Yeasts Candida albicans from the oral cavity 4. Microscopic examination of molds using wet mount 5. Cultural characteristics of Rhizopus & Aspergillus on agar plates 6. Microscopic examination of commercially prepared slides 7. Cultivation of molds on agar culture media INTRODUCTION Fungi are eukaryotic organisms and include the yeasts, molds, and fleshy fungi. Yeasts are microscopic, unicellular fungi; molds are multinucleated, filamentous fungi (such as mildews, rusts, and common household molds); the fleshy fungi include mushrooms. All fungi are chemoheterotrophs, requiring organic compounds for both an energy and carbon source, which obtain nutrients by absorbing them from their environment. Most live off of decaying organic material and are termed saprophytes. Some are parasitic, getting their nutrients from living plants or animals. The study of fungi is termed mycology. In general, fungi are beneficial to humans. They are involved in the decay of dead plants and animals (resulting in the recycling of nutrients in nature), the manufacturing of various industrial and food products, the production of many common antibiotics, and may be eaten themselves for food. Some fungi, however, damage wood and fabrics, spoil foods, and cause a variety of plant and animal diseases, including human infections. Yeasts Yeasts are unicellular, oval or spherical fungi which increase in number asexually by a process termed budding. A bud forms on the outer surface of a parent cell, the nucleus divides with one nucleus entering the forming bud, and cell wall material is laid down between the parent cell and the bud. Usually the bud breaks away to become a new daughter cell but sometimes, as in the case of the yeast Candida, the buds remain attached. 1 Because of their unicellular and microscopic nature, yeast colonies appear similar to bacterial colonies on solid media. Yeasts are facultative anaerobes and can therefore obtain energy by both aerobic respiration and anaerobic fermentation. The vast majority of yeasts are non-pathogenic and some are of great value in industrial fermentations. For example, Saccharomyces species are used for both baking and brewing. The yeast Candida is normal flora of the gastrointestinal tract and is also frequently found on the skin and on the mucous membranes of the mouth. Today we will use Saboraud Dextrose agar (SDA) to grow yeasts. SDA is an agar with a higher sugar concentration and a lower pH, both of which inhibits bacterial growth but promote fungal growth. Molds Molds are multinucleated, filamentous fungi composed of hyphae. A hypha is a branching, tubular structure from 2-10 μm in diameter which is divided into eukaryotic cell-like units by crosswalls called septa in some molds. The total mass of hyphae is termed a mycelium. The portion of the mycelium that anchors the mold and absorbs nutrients is called the vegetative mycelium; the portion that produces asexual reproductive spores is termed the aerial mycelium. Molds can be identified based on the following criteria: Reproductive structure (sexual or asexual spores), appearance of colony (colour & texture), and organization of hyphae (septate or coenocytes) Aspergillus is an example of molds that produce conidiospores. Although usually nonpathogenic, it may become opportunistic in the respiratory tract of a compromised host 2 and, in certain foods, can produce mycotoxins. The conidiophore terminates in a ball-like structure called a vesicle. Rhizopus is an example of a mold that produces sporangiospores. Although usually nonpathogenic, it sometimes causes opportunistic wound and respiratory infections in the compromised host. At the end of its sporangiophore is dome-shaped end called a columella that extends into a sac-like structure called a sporangium. Its sporangiospores, typically brown or black, are produced within the sporangium. Anchoring structures called rhizoids are also produced on the vegetative hyphae. 3 Rhizopus can also reproduce sexually. During sexual reproduction, hyphal tips of (+) and (-) mating type join together and their nuclei fuse to form a sexual spore called a zygospore. This gives rise to a new sporangium producing sporangiospores having DNA that is a recombination of the two parent strain's DNA. Industrially, Aspergillus is used to ferment soybeans into soy sauce and as a decomposer in the environment. Rhizopus sp. is another example of molds, which causes the bread mold Today we will use potato dextrose agar (PDA) to grow molds, because of its complex carbohydrate content and low pH which inhibits bacterial growth, but not fungal growth. Mushrooms Mushrooms are the visible parts of certain fungal growths that live underground, where they feed on dead and decaying matter. When these fungi are ready to reproduce they send up mushrooms, fruiting bodies that carry spores for reproduction. Spores form on the gills of a mushroom underneath its cap. Within a few days they shoot out and scatter, and the fruiting body dies. Some of the billions of spores released will develop into new fungi. Autumn is the best time to spot mushrooms and other fungal fruiting bodies. They may be safe to eat or highly poisonous. 4 5 Lab activities All activities to be done in groups A. Microscopic examination of Yeast using Wet mount 1. From an agar plate: Place a small drop of methylene blue in the center of a slide. Transfer a small portion of a single colony to the drop with a loop and suspend (be certain to allow the loop to cool before picking up specimen). 2. Lower a clean cover slip over the drop as though it were hinged at one side. 3. First focus with the 4x objective on the edge of the coverslip. It is easier to find and focus on than the nearly transparent suspension. 4. Find a bubble in the liquid suspension, and adjust the fine focus on the edge of the bubble. 5. Switch to the 10x objective, repeat the careful focusing. 6. Switch to the 40x objective, repeat the careful focusing. You should be able to distinguish bacteria at this power (magnification = 400x). 7. Partially rotate nosepiece so that 40x and 100x objectives be on both sides of the specimen. Place a small drop of oil on the slide in the center of the lighted area. 8. Rotate turret so that the 100x oil immersion objective touches the oil and clicks into place. 9. Focus only with fine focus. 10. Note the shape and the appearance of budding cells. B. Cultural characteristics of Yeasts on agar plate (prepared plates) 1. Using the terms in the provided Figure, describe the growth characteristics of the provided agar plates. 2. Use a microscope to magnify the colony. C. Isolation of Pathogenic Yeasts Candida albicans from the oral cavity 1. Using a sterile cotton swab, obtain a sample from your tongue. 2. Aseptically, perform a single streak on an SDA plate. 3. Incubate at 370C for 24-48 hours. 6 D. Microscopic examination of molds using wet mount 1. Place two drops of lactophenol cotton blue on a clean microscopic slide. 2. Using sterile needles remove a bit of the fungal growth (Aspergillus & Rhizopus) and place on the stain. 3. Carefully lower the cover slip. 4. Examine your wet mount using low and high magnification powers. E. Cultural characteristics of Rhizopus & Asperegillus on agar plates 1. Using a stereo microscope, examine the provided PDA plates. 2. Determine the characteristic growth features: color and texture. F. Microscopic examination of commercially prepared slides 1. Examine the provided commercially prepared slides showing the different types of fungi. 2. Make fully labeled drawings of these slides. G. Cultivation of molds on agar culture media 3. With the aid of a sterile cork borer, cut an agar disc in the provided cultures of Rhizopus & Aspergillus. 4. Aseptically, pick up the disc with a needle and place it inverted onto the surface of a PDA plate. 5. Incubate at 25°C for 7 days. 6. Observe the growth on the PDA plates. 7 Practical (7) Viruses (Bacteriophages) In this lab session you will learn: 1. The characteristics of viruses. 2. The structure of the coliphageT4. 3. The lytic and lysogenic life cycle of the coliphageT4. 4. Qualitative tests for the detection of bacteriophages (Broth test, spot test and streak test) 5. Quantification of bacteriophages. Introduction Viruses are simply a genome surrounded by a protein coat. They are infectious agents with both living and non-living characteristics. 1. Living characteristics of viruses a. They reproduce at a fantastic rate, but only in living host cells. b. They can mutate. 2. Non-living characteristics of viruses a. They are acellular, that is, they contain no cytoplasm or cellular organelles. b. They do not carry out metabolic reactions on their own and must replicate using the host cell's metabolic machinery. c. Viruses do not grow and divide. Instead, new viral components are synthesized and assembled within the infected host cell. d. With few exceptions, they possess DNA or RNA but never both. Viruses are usually much smaller than bacteria. Most are submicroscopic, ranging in size from 10-250 nanometers. Structurally, they are much simpler than bacteria. Every virus contains a genome of single-stranded or double-stranded DNA or RNA that functions as its genetic material. This is surrounded by a protein shell, called a capsid or core, composed of protein subunits called capsomeres. Many viruses consist of no more than nucleic acid and a capsid, in which case they are referred to as nucleocapsid or naked viruses. Most animal viruses have an envelope surrounding the nucleocapsid and are called enveloped viruses. The envelope usually comes from the host cell's membranes by a process called budding, although the virus does incorporate glycoprotein of its own into the envelope. Bacteriophages Bacteriophages are viruses that infect only bacteria. In addition to the nucleocapsid or head, some have a rather complex tail structure used in adsorption to the cell wall of the host bacterium (Figure 1). Figure 1. The structure of the T4 coliphage Viruses are obligate intracellular parasites that must live and develop inside of living cells. Viruses have the ability to bind specifically to their target host cell, and to enter the host cell or to inject their genome into the host cell. Once the viral genetic material is in the host cell it takes over the metabolism of the host cell and converts it to the process of manufacturing new viral entities (virions). When complete virions are produced within the host cell they leave the cell in a variety of ways. Some viruses cause the host cell to burst or lyse, thereby instantly releasing all the enclosed virions. Other viruses leave their host cells by a complex process of budding off the host's cytoplasmic membrane. In these cases the mature virus usually takes some of the host's cytoplasmic membrane with it when it leaves. Even though the host cells of budding viruses do not burst, they eventually die. Since viruses lack organelles and are totally dependent on the host cell's metabolic machinery for replication, they cannot be grown in synthetic media. In the laboratory, animal viruses are grown in animals, in embryonated eggs, or in cell culture. (In cell culture, the host animal cells are grown in synthetic medium and then infected with viruses.) Plant viruses are grown in plants or in plant cell culture. Bacteriophages are grown in susceptible bacteria. The lytic cycle of bacteriophages Today we will be working with bacteriophages since they are the easiest viruses to study in the lab. Most bacteriophages, such as coliphage T4 that we are using today, replicate by the lytic life cycle and are called lytic bacteriophages. The lytic life cycle of coliphage T4 consists of the following steps: 1. Adsorption Attachment sites on the bacteriophage tail adsorb to receptor sites on the cell wall of a susceptible host bacterium. 2. Penetration A bacteriophage enzyme "drills" a hole in the bacterial cell wall and the bacteriophage injects its genome into the bacterium. This begins the eclipse period, the period in which no intact bacteriophages are seen within the bacterium. 3. Replication Enzymes coded by the bacteriophage genome shut down the bacterium's macromolecular (protein, RNA, DNA) synthesis. The bacteriophage genome replicates and the bacterium's metabolic machinery is used to synthesize bacteriophage enzymes and bacteriophage structural components. 4. Maturation The bacteriophage parts assemble around the genome. 5. Release A bacteriophage-coded lysozyme breaks down the bacterial peptidoglycan causing osmotic lysis of the bacterium and release of the intact bacteriophages. 6. Reinfection From 50-200 bacteriophages may be produced per infected bacterium and they now infect surrounding bacteria. Figure 2. The lytic cycle and lysogenic cycles of a bacteriophage. The lysogenic cycle of bacteriophages Some bacteriophages replicate by the lysogenic life cycle and are called temperate bacteriophages. When a temperate bacteriophage infects a bacterium, it can either (1) replicate by the lytic life cycle or cause lysis of the host bacterium, or (2) it can incorporate its DNA into the bacterium's DNA and assume a noninfectious state. In the latter case, the cycle begins by the bacteriophage adsorbing to the host bacterium and injecting its genome, as in the lytic cycle. However, the bacteriophage does not shut down the host bacterium. Instead, the bacteriophage DNA inserts or integrates into the host bacterium's DNA. At this stage, the virus is called a prophage. Expression of the bacteriophage genes controlling bacteriophage replication is repressed by a repressor protein and the bacteriophage DNA replicates as a part of the bacterial nucleoid. However, in approximately one in every million to one in every billion bacteria containing a prophage, spontaneous induction occurs. The bacteriophage genes are activated and bacteriophages are produced as in the lytic life cycle. Quantification test for the enumeration of phage particles Phage number can be determined based on their infectivity of bacteria. Serial dilution of the phage sample are plated out on uniform growth of bacteria on the surface of an agar plate known as a bacterial lawn. Each phage that adsorbs to a bacterium will reproduce and cause lysis of that bacterium. The released phages then infect neighboring bacteria, causing their lysis. Eventually a visible self-limiting area of lysis, a plaque, is observed on the plate. Figure 3. Quantitative test for the enumeration of phages. Qualitative Tests to determine the presence of phages Qualitative tests are performed to demonstrate the presence of the phage only. In this lab, you will perform the following qualitative tests: broth test, spot test and streak plate test. Tryptic soy medium, a general-purpose non-selective medium, will be used for these tests. Lab activities A. Broth Test 1. Take three 5 ml TSB tubes and label them according to the table below. Tube label Contents in ml E. coli Phage Test 0.5 ml 0.1 ml E. coli control 0.5 ml 0 Phage control 0 0.1 ml 2. Add the appropriate amount of E. coli and/or phage into each of your labelled test tubes using a pipette. 3. Incubate the tubes at 37 o C for 24 hr. 4. Record your results as turbid or clear in your work sheet. B. Spot Test 1. Take three TSA agar plates and label them as spot test, E.coli control, and phage control. 2. Dip a sterile cotton swab in the E. coli broth culture and swipe it over the surface of TSA labelled E.coli control and spot test without leaving space. 3. Spot a loop full of the phage in the center of spot test plate and the phage control plate. 4. Allow the phage to absorb into the medium and incubate at 37 o C right side up. 5. Plaque formation (circular zone of clearing) indicates a sample is positive for phages. If the spot contains an intact lawn of bacteria indistinguishable from the background lawn of bacteria, this indicates a negative result. Record results. C. Streak plate Test 1. Label three TSA plates as: test plate, E. coli control, and phage control. 2. Using a sterile inoculating loop, streak 4 parallel lines of your E. coli culture on test plate and E. coli control plate. 3. Rotate your plates, and streak 4 parallel lines of your phage so that they are at right angles with the E. coli. 4. Streak 4 parallel lines of your phage on the phage control plate. 5. Incubate the 3 TSA plates, right sides up at 37°C for 24 hrs. 6. Record your results. Lab Discussion 1. Define the following: bacteriophage, prophage, and plaque. 2. Describe the structure of the bacteriophage coliphage T4 and the function of its components. 3. Describe the lytic life cycle of bacteriophages. 4. Compare between qualitative tests and quantitative tests. Practical (8) Microbial Ubiquity In this lab session you will learn: 1. The ubiquity of microorganisms 2. Microbial isolation techniques from different environments 3. Quantification of microorganisms in an environmental sample Introduction The term microbe includes a wide range of organisms, from single cell prokaryotes (bacteria and archaea) to single and multi-celled eukaryotes (fungi, algae and protozoa). The term even includes non-living viruses. Microorganisms are found throughout the environment: in the air and water; on the surface of any object such as clothes, walls, furniture; in soil and dust; and on and in our own bodies (skin and mucous membranes). Microbes are even found in extreme, seemingly inhospitable environments: living and thriving microbes are common in hot springs with temperatures in excess of 95°C, in Antarctic seawater below 0°C, and in acid mine waters whose pH is below 4. Ordinarily these ubiquitous microorganisms are of no threat to healthy humans, and quite a few are actually beneficial. Normal flora, on and in our body, act as an initial defense against invading pathogens. Normal flora compete with foreign microorganisms for nutrients and space, thereby preventing them from flourishing. In the absence of the normal flora (e.g., due to prolonged antibiotic treatment), opportunistic microorganisms may become established, increasing the risk of developing disease. In the environment, many organisms in soil play important geochemical roles in processing vital elements such as phosphorus and nitrogen, making them available to other living things. Nonetheless, there are organisms in our environment that are harmful: soil also harbors the spores of the tetanus and botulism bacteria. In order to demonstrate the ubiquity and diversity of microbes in the environment, samples from soil, water, immediate areas of the environment and/or from your body will be obtained and cultured. 1 Possible samples: Human body Environment Throat Bench top (before and after disinfection) Skin Water (tap, sea, stagnant) Between toes soil Ear Air (exposed plate) Lab activities A. Qualitative isolation of soil microorganisms 1. Label a tube of sterile nutrient broth "Sand". Add 1 gram of sand soil. 2. Repeat the above step with garden soil. 3. Label a third sterile nutrient broth as "Control". 4. Incubate the broth tubes as 300 C for 48 hrs. 5. Keep the tubes in the refrigerator until the next lab session. B. Quantitative isolation of soil microorganisms 1. Add 10 grams of garden soil to 90 ml sterile water. Label as "Original sample". 2. Shake vigorously for 20 minutes. Leave it to stand. 3. Set up four test tubes each containing 9 ml of sterile water. Label them 10 -1, 10-2, 10-3, and 10-4. 4. Serially dilute down the series of the four tubes. i.e. transfer 1 ml of the original sample into the 10-1 tube, mix, one ml of 10-1into the 10-2 , mix. 5. Aseptically, transfer 0.1 ml from each dilution and spread on 2 nutrient agar (NA) plates, 2 potato dextrose agar (PDA) plates, and 2 PDA plates with anti fungal agent. 6. Incubate NA plates at 300 C for 48 hrs. 7. Incubate PDA and PDA with anti fungal agent plates at 30 0 C for 7 days. 8. Keep the plates in the refrigerator until the next lab session. C. Isolation of air microorganisms 1. Label one NA plate and one PDA plate. 2. Expose your plates for 30 min. in the tested area. 3. Close the plates and incubate at 300 C for 48 hrs. 4. Keep the plates in the refrigerator until the next lab session. D. Isolation of water microorganisms 1. Label three NA plates with the appropriate water sample (tap water, sea water, and stagnant water). 2. Aseptically, transfer 0.1 ml of the tap water onto the plate labelled as "tap water". Using a glass spreader spread the sample over the entire surface of the plate. 3. Repeat the above step with the other water samples. 4. Incubate at 300 C for 48 hrs. 5. Keep the plates in the refrigerator until the next lab session. 2 E. Isolation of microorganisms from human skin 1. Label one TSA plate as "Lips" 2. Press your lips gently on the agar surface. 3. Label another TSA plate as "Fingers" 4. Place your fingers gently on the agar surface. 5. Label a third TSA plate as "Ear". 6. Remove the sterile cotton swab from the package and immerse it in the sterile water. Wring out the excess water on the inside of the test tube and rub the swab over your ear. 7. Gently, so as not to mess up the agar surface, rub the swab over the entire surface of the plate without going back over areas you have already swabbed. 8. Incubate at 370 C for 48 hrs. 9. Keep the plates in the refrigerator until the next lab session. F. Isolation of microorganisms from human body orifices 1. Label a TSA plate as "tongue". 2. Press your tongue gently on the agar surface. 3. Label a TSA plate as "Cough". 4. Vigorously cough onto your open TSA plate. Close the plate. 5. Incubate at 370 C for 48 hrs. 6. Keep the plates in the refrigerator until the next lab session. 3 Practical (9) Biochemical Tests In this Lab session, you will learn: 1. The use of biochemical tests to differentiates microorganisms 2. The scientific basis of biochemical tests 3. Tests to detect; starch utilization, fermentation of carbohydrates, INTRODUCTION In the previous labs we examined bacteria microscopically. Staining provides valuable information as to bacterial morphology and gram reaction. Beyond that, however, microscopic observation gives little additional information as to the genus and species of a particular bacterium. To identify bacteria, we must rely heavily on biochemical testing. The types of biochemical reactions each organism undergoes act as a "thumbprint" for its identification. This is based on the following chain of logic: Each different species of bacterium has a different molecule of DNA (i.e., DNA with a unique series of nucleotide bases). Since DNA codes for protein synthesis, then different species of bacteria must, by way of their unique DNA, be able to synthesize different protein enzymes. Enzymes catalyze all the various chemical reactions of which the organism is capable. This in turn means that different species of bacteria must carry out different and unique sets of biochemical reactions. In general, we can classify enzymes as being either exoenzymes or endoenzymes. Exoenzymes are secreted by bacteria into the surrounding environment in order to break down larger nutrient molecules so they may enter the bacterium. Once inside the organism, some of the nutrients are further broken down to yield energy for driving various cellular functions, while others are used to form building blocks for the synthesis of cellular components. These later reactions are catalyzed by endoenzymes located within the bacterium. (A) STARCH HYDROLYSIS Starch is a polysaccharide that appears as a branched polymer of the simple sugar glucose. This means that starch is really a series of glucose molecules hooked together to form a long chain. Additional glucose molecules then branch off of this chain as shown below. GLU | ( ---GLU-GLU-GLU-GLU-GLU-GLU-GLU--- )n Some bacteria are capable of using starch as a source of carbohydrate but in order to do this they must first hydrolyze or break down the starch so it may enter the cell. The bacterium secretes an exoenzyme that hydrolyzes the starch by breaking the bonds between the glucose molecules. This enzyme is called a diastase. ( ---GLU GLU GLU GLU GLU GLU GLU--- )n The glucose can then enter the bacterium and be used for metabolism. When iodine is added to starch, the iodine-starch complex that forms gives a characteristic dark brown or deep purple color reaction. If the starch has been hydrolyzed into glucose molecules by the diastase exoenzyme, it no longer gives this reaction. (B) FERMENTATION OF CARBOHYDRATES Carbohydrates are complex chemical substrates that serve as energy sources when broken down by bacteria and other cells. They are composed of carbon, hydrogen, and oxygen (with hydrogen and oxygen being in the same ratio as water; [CH2O]) and are usually classed as either sugars or starches. Fermentation of Carbohydrates by Bacteria Facultative anaerobic and anaerobic bacteria are capable of fermentation, an anaerobic process during which carbohydrates are broken down for energy production. A wide variety of carbohydrates can be fermented by different bacteria in order to obtain energy and the types of carbohydrates which are fermented by a specific organism can serve as a diagnostic tool for the identification of that organism. We can detect whether a specific carbohydrate has been fermented by looking for common end products of fermentation. When carbohydrates are fermented as a result of bacterial enzymes, the following fermentation end products may be produced: 1. Acid end products, or 2. Acid and gas end products. In order to test for these fermentation products, you inoculate and incubate tubes of media containing a single carbohydrate (such as lactose or maltose), a pH indicator (such as phenol red) and a Durham tube (a small inverted tube to detect gas production). If the particular carbohydrate is fermented by the bacterium, acid end products will be produced which lowers the pH, causing the pH indicator to change color (phenol red turns yellow). If gas is produced along with the acid, it collects in the Durham tube as a gas bubble. If the carbohydrate is not fermented, no acid or gas will be produced and the phenol red will remain red. Fermentation of Carbohydrates by Yeasts The enzymes in yeast break down glucose to give two products: ethanol (alcohol) and carbon dioxide. Fermentation processes have long been utilized in baking bread, making beer and wine, and producing cheese, yogurt, soy sauce, and many other foodstuffs. Baker's yeast is cultivated from the strain Saccharomyces cerevisiae because of its superior fermentation abilities. With respect to their metabolism baker's yeasts are facultative anaerobe. They can ferment or respire depending upon environmental conditions. In the presence of oxygen respiration takes place, without oxygen present, fermentation occurs. During anaerobic conditions high levels of NADH develop, leaving a shortage of NAD+. Low levels of NAD+ slow the rate of glycolysis. Fermentation restores NAD+ levels while producing alcohol and CO2. During aerobic respiration glucose is broken down into water and carbon dioxide. C6H12O6 + O2 6H2O + 6CO2 Under ideal conditions most eukaryotic cells produce 36 ATP molecules from one molecule of glucose. During fermentation, baker's yeast breaks glucose into ethyl alcohol and carbon dioxide. C6H12O6 2C2H5OH + 2CO2 This process only yields 2 ATP per glucose molecule. (C) CATALASE ACTIVITY Catalase is the name of an enzyme found in most bacteria which initiates the breakdown of hydrogen peroxide (H2O2) into water and free oxygen: 2H2O2 ------ catalase---------> 2H2O + O2 During the normal process of aerobic respiration, hydrogen ions (H+)