FHP LEC3 - Muscle Physiology - Mujica 2024 PDF
Document Details
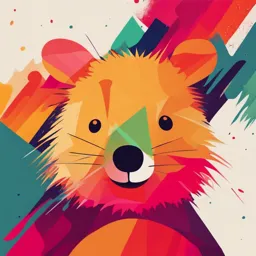
Uploaded by UnquestionableKremlin
Rutgers University
2024
Patricio E. Mujica
Tags
Summary
This document, "FHP LEC3 - Muscle Physiology - Mujica 2024", is a lecture on muscle physiology focusing on skeletal, cardiac, and smooth muscle. It covers excitation-contraction coupling, sarcomere function, and muscle mechanics. The document was written by Patricio Mujica.
Full Transcript
Physiology of Skeletal, Cardiac, and Smooth Muscle Patricio E. Mujica, Ph.D. Department of Pharmacology, Physiology and Neuroscience MSB H655 [email protected] Slideshow originally developed by Krista N. Blackwell, Ph.D. By the end of this lecture you should be able to: Identify the macros...
Physiology of Skeletal, Cardiac, and Smooth Muscle Patricio E. Mujica, Ph.D. Department of Pharmacology, Physiology and Neuroscience MSB H655 [email protected] Slideshow originally developed by Krista N. Blackwell, Ph.D. By the end of this lecture you should be able to: Identify the macroscopic and microscopy anatomy/ultrastructure of skeletal muscle fibers, cardiac muscle and smooth muscle. Compare and contrast the events of excitation-contraction coupling (including relevant receptors and channels) for skeletal, smooth and cardiac muscle Compare the characteristics of fast- and slow-twitch, oxidative and glycolytic fibers. Describe the mechanisms for temporal and spatial summation of skeletal muscle Diagram and describe how sarcomere contracts with optimal force if it is at optimal length (length-tension relationship) and identify the differences of this relationship when comparing skeletal and cardiac muscle Diagram the force-velocity relation in skeletal and cardiac muscle Describe how and why tetanus is prevented in cardiac muscle Three Types of Muscle Tissue Skeletal muscle is the main muscle form in the body Skeletal Muscles make up ~30-45% of the total body weight are under voluntary control are mostly attached to bone, at origin and insertion – results in a basal level of stretch always placed on the muscles Functions include: – Production of skeletal movement – Maintaining posture and body position – Protect internal organs (abdominal & pelvic organs) – Generate heat Skeletal muscle organization Skeletal muscle has three connective tissue layers: the epimysium, perimysium and endomysium Each skeletal muscle is enclosed by the epimysium Within each skeletal muscle there are bundles of muscle fibers known as fascicles Fascicles are enclosed by the perimysium – Each sheath is surrounded elastic fibers, nerves and blood vessels that are also necessary for muscle function The innermost connective tissue layer, the endomysium, encloses the skeletal muscle cells or myofibrils Skeletal muscle organization The sarcolemma is the cell membrane of a muscle fiber Transverse tubules (T-tubules) are deep invaginations of the sarcolemma that allow rapid conduction of action potentials throughout the muscle The sarcoplasmic reticulum (SR) is a modified endoplasmic reticulum that serves as a major store for calcium inside the cell The terminal cisternae are enlarged regions of the SR that are closely apposed to the ttubules Triads are two terminal cisterna and one t-tubule, which serve as the site of initiation of muscle contraction Sarcomeres are the contractile units of skeletal and cardiac muscle A-band: contains thick (myosin) and thin filaments (actin) I-band: thin filaments only (actin & tropomyosin) Each I-band is dissected by Z-line which contain titin, nebulin, desmin & actinin which anchor the thin filaments M-line: center of the sarcomere; region where thick and thin filaments do not overlap H-zone: contains only thick (myosin) filaments Sarcomeres shorten during muscle contraction With muscle contraction, the I band and the H band shorten, while the A band remains unchanged Thin filaments consist of actin chains intertwined with troponin and tropomyosin proteins Actin is a globular protein (G-actin) arranged as a chain of repeating units, forming two strands of an alpha helix – Each G-actin molecule has a myosinbinding site Tropomyosin wraps around actin filaments and covers the myosinbinding sites Troponin (Tn) is made up of 3 subunits: – Tn-T is attached to tropomyosin – Tn-I inhibits myosin binding site on actin – Tn-C has Ca2+ binding sites (4 Ca2+ bind to 1 Tn-C) Nebulin and titin serve as supporting proteins for the actin strands Thick filaments consist of myosin molecules that form a protein chain with a head and a tail Myosin molecules are composed of intertwining protein chains that form a long stiff tail, an elastic hinge region and two heads – Each myosin head has a heavy chain (HC) and a light chain (LC) – The heavy chain contains an ATP binding site necessary for muscle contraction (myosin ATPase) and a binding site for actin – The hinge region is the elastic component that allows the head to swivel and move (power stroke) Troponin and tropomyosin are critical for calcium binding and muscle contraction Major Steps in Excitation-Contraction Coupling Excitation-contraction coupling is the process in which an action potential causes calcium concentration to increase in the cytosol, leading to contraction of the muscle. Steps: 1. Excitation: the action potential generation at the neuromuscular junction 2. Coupling occurs when the action potential propagates to the triads resulting in the release of calcium from the sarcoplasmic reticulum 3. Contraction occurs when calcium binds to the myofibrils and the muscle fiber shortens (powerstroke occurs) 4. Relaxation occurs when calcium is released from the myofibrils and returned to the SR Neuromuscular Junction Sequence of Events at the Neuromuscular junction for Skeletal Muscle Contraction The neuromuscular junction is the synapse between a motor neuron and a muscle fiber 1. Action potentials are propagated down the somatic motor neuron 2. The presynaptic terminal becomes depolarized and voltage-gated calcium channels open, increasing calcium permeability 3. Calcium ions entering the presynaptic terminals stimulate the fusion of ACh-containing vesicles with plasma membrane 4. (a) ACh is released into the synaptic cleft and diffuses to the postsynaptic membrane Sequence of Events at the Neuromuscular junction for Skeletal Muscle Contraction 4. (b) The motor-end plate contains nicotinic receptors that bind ACh 5. The nicotinic receptors open allowing Na and K ions flux across the motor end plate causing depolarization and the development of an end plate potential (EPP) 6. The EPP spreads across the muscle fiber, resulting in depolarization and the firing of an action potential in skeletal muscle which spreads along the t-tubules 7. The EPP is terminated when ACh is degraded by Acetylcholinesterase (AChE) in the synaptic cleft Neuromuscular Transmission can be altered by toxins and drugs Excitation is the generation of the action potential at the neuromuscular junction The triads are the site of coupling electrical activity and calcium release for muscle contraction Triads are sites of close apposition of T-tubular and sarcoplasmic reticulum (SR) membranes – They convert the electrical signal into a chemical (release of Ca2+ from the SR) signal When the t-tubules are depolarized dihydropyridine receptors (L-type calcium channels) cause a conformational change in the ryanodine receptor (calcium release channels) – The ryanodine receptors open allowing calcium to diffuse from the SR into the cytosol – Calcium is released into the cytosol and available to bind to troponin C to initiate muscle contraction Coupling occurs when the action potential propagates to the triads resulting in the release of calcium from the sarcoplasmic reticulum Contraction occurs when calcium binds to the myofibrils and the muscle fiber shortens Power Stroke in Skeletal Muscle and Rigor The release of ADP stabilizes the myosin-actin interaction The cycle stops here when [ATP] ↓ In the continuous presence of ATP and Ca2+, ATP binds to a now exposed site on the myosin HC. At the end of the power stroke myosin releases ADP, exposing the ATP-binding site in the myosin HC Myosin heads rotate, move the attached actin, and shorten the muscle fiber, causing the power stroke. ATP binding destabilizes the myosin-actin interaction; myosin releases actin and returns to the “cocked” position. At rest, myosin heads are bound to adenosine diphosphate and are said to be in a “cocked” position in relation to the thin filament Ca2+ binding to the troponin—tropomyosin complex induces a conformational change in the thin filament that allows for myosin heads to cross-bridge with thin filament actin. Note: Although the cycle starts with the rigor state, relaxed muscle fibers remain mostly in step 2 (cocked position). The rigor state is brief in in living muscle Relaxation occurs when calcium is released from the myofibrils and returned to the SR Skeletal Muscle Mechanics A skeletal muscle twitch occurs with one cycle of excitation-contraction coupling (ECC) A muscle twitch results from one stimulus or contraction–relaxation cycle – results in 20-30% of the maximum tension possible – muscle completely relaxes between each twitch Duration of Key Steps in Skeletal Muscle ECC AP propagation along sarcolemma (5-10 ms) AP propagation along T- tubules (~0.7 ms) Peak rate of Ca2+ release from (SR ~ 5 ms) Peak muscle tension (~25 ms) Types of Contraction: Isometric and Isotonic Isometric contractions do not shorten the muscle fiber Isotonic Contractions occur with Muscle Movement During an isotonic contraction the tension that is developed stays the same, but the muscle shortens and is able to lift the load Isotonic contraction = tension development followed by shortening There are two types of isotonic contractions: – – Concentric Contraction: tension develops while the muscle shortens Eccentric Contraction: tension develops while the muscle lengthens The length-tension describes how muscles develop the necessary force for contraction The length-tension relationship is the relationship between the length of the fiber and the force that the fiber produces Fiber length refers to the length of an isolated fiber and the degree of overlap between the actin and myosin filaments in the sarcomeres Skeletal muscle always operates at optimal resting length, so it always produces the maximum force or tension that can be produced in the muscle Length-Tension relationship and Force Generation Force generation over time Change in length over time (optimal length in center: 8 units) Passive Force: force applied to the muscle before stimulation (minimal in skeletal muscle, as optimal length is approached) Active Force: force that results when the muscle is stimulated Total Force: the combination of active and passive tension At very short or very long lengths, active force development declines Passive tension rises significantly when excessive stretch is placed on the fiber Force (Load)-Velocity Relationship The force-velocity relationship describes the velocity of shortening as a product of changes in afterload The velocity of shortening reflects the speed of crossbridge cycling As the afterload increases, the velocity of shortening decreases (due to the higher resistance to shortening) Temporal (wave) summation can lead to the development of tetanus Temporal recruitment occurs with the firing of an increased number of action potentials Twitches are added up (summation) to produce more force – – – – Multiple stimuli (i.e. 2nd, 3rd, 4th…) are summed Sequentially fired before the muscle completely relaxes During summation, the second contraction starts at the beginning of relaxation of the first contraction Results in a greater contractile force Tetanus occurs when many stimuli are given very close together, resulting in continuous contractions and the development of maximum tension (force) Multiple motor units work together to allow spatial summation in skeletal muscles Spatial summation is the increased number of motor neurons firing action potentials – Results in the contraction of more motor units – Within one motor unit all fibers contract simultaneously. Recruitment of Motor Units: Size Principle 1. Type I motor units are recruited first. Small motor neuron has small cell body and axon diameter, low threshold. Innervate small fibers, with smallest force or twitch tension and slowest contraction. 2. Type IIa MUs are recruited next. Medium motor neuron innervates medium fibers with larger forces and faster contraction times. 3. Type IIb MUs are recruited last. Large motor neuron innervates largest fibers with fast twitch, largest force and fastest contraction. Energy Sources for Muscle Contraction Three Types of Skeletal Muscle Fibers There are three main types of Skeletal muscle fibers: Slow oxidative (Type I, SO) muscle fibers are adapted for slow contractions over long periods without fatigue have many mitochondria, many surrounding capillaries, and much myoglobin, These features that make the tissue appear dark or red in color. Fast glycolytic (Type II b, FG) fibers are specialized for rapid, short-term contraction have few mitochondria or capillaries depending largely on anaerobic metabolism of glucose derived from stored glycogen White in appearance Rapid contractions lead to rapid fatigue as lactic acid produced by glycolysis accumulates. Fast oxidative-glycolytic (Type II a, FOG) fibers have physiological and histological features intermediate between those of the other two types Pink (light red) in appearance Skeletal Muscle Fiber Types Physiology of Cardiac Muscle The heart is a hollow muscular pump that pumps the blood throughout the vasculature Atrial Myocytes Ventricular Myocytes Ventricular Muscle Cells: Striated muscle cells, usually with two nuclei. Cells branch Sarcomere structure is similar to skeletal muscle fibers. Cells are rich in mitochondria (1/3 of volume), have less developed SR. Intercalated disks have gap junctions that electrically couple cardiac myocytes Copyright ©2010 Pearson Education, Inc. or its affiliates. All Rights Reserved. www.trincoll.edu/.../Jason_Final-Muscle-Med.jpg Cells are connected end-to-end by gap junctions. Cells form functional syncytium (all behave as one). Pacemaker cells initiate the action potentials necessary for cardiac muscle contraction Contractile Cell Action Potential Contractile cells make up 95-99% of the of the myocardial cells of the heart Pacemaker (Autorhythmic) Cell Action Potential Pacemaker cells make up 1-5% of the myocardial cells and are responsible for initiating heart action potentials Major Steps in Excitation-Contraction Coupling (Cardiac Muscle) Excitation-contraction coupling is the process in which an action potential leads to an increased calcium concentration in the cytosol and contraction of the muscle 1. Excitation: the action potential generation by cardiac pacemaker cells 2. Coupling occurs when the action potential propagates to the dyads resulting in the release of calcium from the sarcoplasmic reticulum 3. Contraction occurs when calcium binds to the myofibrils and the muscle fiber shortens (powerstroke occurs) 4. Relaxation occurs when calcium is released from the myofibrils and returned to the SR Excitation-contraction coupling of the heart is similar to skeletal muscle The length of the contractile cell action potential prevents tetanus of cardiac muscle Cardiac muscle does not contract in an all-or-nothing manner Skeletal muscle Resting sarcomere length – optimal length (~ 2.2 μm) Contracts in an all-or-nothing manner Cardiac muscle Myocytes have higher passive tension (due to the blood filling the ventricles and the stretching of the muscle) Resting sarcomere length is less than optimal (~1.8 μm) Can have graded contractions because the heart can increase the contractile force it develops under changing conditions (up to the optimal length) Active and Passive Tension Curves Fig. from Jewell, in "The Physiological Basis of Starling's Law of the Heart" CIBA Fdn Symp. 24, 1974, as modified by Katz, in "Physiology of the Heart," Raven Press, 1992. Cardiac muscle has a larger resting (passive) tension compared to skeletal muscle blood entering the heart stretches cardiac muscle. the heart operates at sarcomere lengths lower than the optimal so that it can modulate the force of contraction needed to eject the blood. Physiology of Smooth Muscle Smooth muscle function is important to the maintenance of homeostasis in many body systems Many different locations in the body – It is located in the vascular system (blood vessel walls), gastrointestinal tract (esophagus, stomach, etc.), urinary system (bladder), reproductive system (uterus) and the eye Varying contractile patterns – In some organs of the body, smooth muscle can undergo phasic contractions (cyclical) or tonic (continuous) contractions Two functionally different types – Single-unit smooth muscle – Multi-unit smooth muscle Involuntary muscle – Requires neural or hormonal input for contraction to occur There are two types of smooth muscle Smooth Muscle Organization Cells are much smaller than skeletal muscle fibers (~ 5 µm wide, ~ 100 µm long) Cells are not striated, have a smooth appearance Have less developed SR, compared to skeletal and cardiac muscle There are multiple small membrane invaginations – caveolae, but no T-tubules Smooth muscle organization Smooth muscle contains less contractile proteins than skeletal muscle… but the actin/myosin ratio is ~10-15:1 (actin filaments are much longer) Thin filaments: actin is stabilized by dense bodies (no troponin complex) Thick filaments: myosin Intermediate filaments: support cytoskeletal function (desmin and vimentin) Dense bodies: cytoplasmic and membrane-associated proteins (contain α-actinin, desmin and vimentin) – serve as anchors for actin – analog of Z-lines Smooth muscle organization During contraction the filaments slide together making cell rounder Contraction can be coupled to changes in membrane potentials or ligand binding Generation of an action potential is not always necessary for contraction – if AP occurs there is almost always contraction (electromechanical coupling) Slow wave potentials – cyclic depolarizations and repolarizations – can be sub-threshold or reach threshold and fire action potentials Some smooth muscle have pacemaker cells – can spontaneously fire action potentials, independent of nervous system control Contraction can be coupled to changes in membrane potentials or ligand binding Smooth muscle contraction can occur without substantial changes in membrane potential (pharmacomechanical coupling). Autonomic nervous system neurotransmitters (i.e. epinephrine, norepinephrine, acetylcholine) GPCR activation (via Gq-IP3 and GscAMP pathways) Paracrine substances (NO, Histamine) Smooth muscle requires calmodulin to bind calcium to initiate muscle contraction In smooth muscle, calcium can come into the cytoplasm from the extracellular compartment (CICR) or from the SR (IP3-GPCR) Unique to smooth muscle is the calcium-calmodulin (CaM) binding that is required – Four Ca2+ ions bind to calmodulin – Ca2+-CaM binds to activate myosin light chain kinase (MLCK) – MLCK phosphorylates the regulatory light chains on myosin (requires 1 ATP/Ser), – Increases ATPase activity of myosin – Takes time! Fast cross-bridge cycling similar to striated muscle – Requires 1 ATP per each cycle. Myosin light chain phosphatase plays a role in smooth muscle relaxation Myosin light chain phosphatase (MLCP) decreases the Myosin ATPase activity – results in a reduction of muscle tension Myosin phosphatase activity does not dictate complete relaxation of smooth muscle Smooth muscle has the ability to prolong muscle contraction without using large amounts of ATP “Latch state” allows prolonged smooth muscle contraction Because smooth muscle may need to maintain force for long periods, there is a unique latch state that allows the muscle to maintain tension without extensive energy utilization The latch state – allows smooth muscle to maintain tone (force) with minimal expenditure of ATP – occurs because crossbridges dissociate slowly (despite MLCK dephosphorylation and Myosin light chain phosphatase activity), reducing energy expenditure – can be maintained with ~30% cross-bridges phosphorylated – is important in muscles which require long periods of tension development (which would theoretically use large amounts of energy) Smooth Muscle Mechanics Force Velocity Relationship-Smooth Muscle Slower contraction, slower relaxation (phosphorylation takes time, slow crossbridge dissociation—seconds-scale) Maximal velocity directly depends on cross-bridge phosphorylation. Length-Tension Relationship-Smooth Muscle Passive tension curve is shifted to the left compared to two other muscle types (larger amount of connective tissue—resistance!) Smooth muscle cells can shorten/lengthen more than skeletal and cardiac cells. Comparisons of Skeletal, Smooth and Cardiac Muscle