Extractive Metallurgy Notes 2017 PDF
Document Details
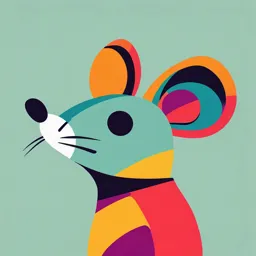
Uploaded by ImmenseWillow
2017
Tags
Summary
This document is a chapter on extractive metallurgy, from a 2017 course. It provides an overview of the topic, highlighting the three main branches: pyrometallurgy, hydrometallurgy, and electrometallurgy, along with detailed descriptions of each. It covers the basic principles and processes involved in extractive metallurgy, with a focus on pyrometallurgy and the different types of fuels used in the process.
Full Transcript
CHAPTER ONE OVERVIEW OF EXTRACTIVE METALLURGY 1.1 Introduction to Extractive Metallurgy Extractive metallurgy deals with metallurgical processes that target the chemical make-up of the ore or concentrate in order to liberate, recover and extract the...
CHAPTER ONE OVERVIEW OF EXTRACTIVE METALLURGY 1.1 Introduction to Extractive Metallurgy Extractive metallurgy deals with metallurgical processes that target the chemical make-up of the ore or concentrate in order to liberate, recover and extract the mineral of interest. Thus, comminution is carried out to reduce the ore to sizes fine enough to liberate the mineral of interest after which concentration is conducted to upgrade the ore. However, some of the minerals, due to the fineness in size, do not respond favourably to the physical methods of concentration. There are even some minerals, which are not liberated at all by any of the mechanical methods of mineral processing. Hence, extractive metallurgical processes are employed to fully liberate, recover and extract the mineral of interest. In most cases of metal recovery, the marketable product is always yielded after extractive metallurgy. Extractive metallurgy has three main branches; pyrometallurgy, hydrometallurgy and electrometallurgy. This course therefore looks at the basic principles of extractive metallurgy for mining students. The fundamentals of extractive metallurgy of gold are also considered since gold is the mineral mostly exploited in Ghana. 1.2 Branches in Extractive Metallurgy 1.2.1 Pyrometallurgy Pyrometallurgy is a branch of extractive metallurgy which deals with the extraction of metals from their ores by thermal methods. Thus it employs heat in the treatment of minerals and metallurgical ores and concentrates to bring about physical and chemical transformations in the materials to enable recovery of valuable metals. Pyrometallurgical treatment may produce saleable products such as pure metals, or intermediate compounds or alloys, suitable as feed for further processing. Pyrometallurgical processes are generally grouped into one or more of the following categories: Drying, Calcining, Roasting, Smelting and Refining. 1 Most of the processes require energy input to sustain the temperature at which the process takes place. The energy is usually provided in the form of combustion of carbonaceous fuel or from electrical heat. Carbonaceous fuels are the main sources of energy in pyrometallurgy and the furnaces that are used are lined with refractories. 1.2.2 Hydrometallurgy Hydrometallurgy is a branch of extractive metallurgy that extracts metals from ores by aqueous methods. In hydrometallurgy, a condition is developed that helps to extract the mineral of interest leaving the gangue mineral by making use of a suitable extractant (or solvent). It involves three (3) processes or steps namely; Leaching (e.g. Agitation, Heap, Dump and In-situ Leaching) Solution Purification (e.g. Adsorption, Ion exchange, Ion flotation, Solvent extraction and Precipitation) Metal Recovery (e.g. Crystallization, Adsorption, Electrolysis and Precipitation) 1.2.3 Electrometallurgy Electrometallurgy is the branch of electrochemical engineering, which deals with the extraction and refining of metals from aqueous and molten solutions. It uses the medium of electricity and is based on the principle of electrochemistry. 1.3 Course Presentation and Assessment This course will be delivered through lectures with supporting lecture notes and practical work at the laboratory to aid students’ assimilation of the course. Assignments will be given after treating each chapter to test students’ understanding. These assignments should be presented to the lecturer before the commencement of a new chapter. Students will be assessed through: Continuous Assessment 40 % and Final exam 60 % The continuous assessment will comprise, Assignments, class test, announced quizzes and class attendance. 2 1.4 Course Aims and Objectives The main aim of this course is; To prepare students to become competent mining engineers by equipping them with extractive metallurgical processes essential in mineral processing. Course objectives include: To educate students on extractive metallurgy To introduce students to various branches and processes involved in extractive metallurgy To encourage students to make good use of the knowledge acquired during this lectures in their profession as mining engineers 1.5 Expected outcome of the Course At the end of the course, students are expected to: Gain good knowledge of extractive metallurgy Apply the principles learnt during course delivery in practical work both as a student and a professional engineer 3 CHAPTER TWO PYROMETALLURGY 2.1 Introduction Pyrometallurgy consists of the thermal treatment of minerals and metallurgical ores and concentrates to bring about physical and chemical transformations in the materials to enable recovery of valuable metals. Pyrometallurgical treatment may produce saleable products such as pure metals or intermediate compounds or alloys, suitable as feed for further processing. 2.2 Processes in Pyrometallurgy Pyrometallurgical processes are generally grouped into one or more of the following categories: Drying Calcining Roasting Smelting Refining Most of the processes require energy input to sustain the temperature at which the process takes place. The energy is usually provided in the form of combustion of carbonaceous fuel or from electrical heat. 2.3 Fuel Fuel is any material that is burned or altered in order to obtain energy to produce heat that is enough to be applied in industrial operation. Fuel releases its energy either through a chemical reaction such as combustion, or nuclear means, such as nuclear fission or nuclear fusion. An important property of a useful fuel is that its energy can be stored and released only when needed, and that the release is controlled in such a way that the energy can be harnessed to produce work. Examples: Methane, Petrol and Wood. 4 The major sources of energy used in pyrometallurgy are electrical energy and by combustion of carbonaceous fuels. 2.3.1 Electrical energy This is generated mainly from waterfall or waterpower, burning of carbonaceous fuels (e.g. coal, oil,) and by uranium fission in a nuclear reactor. It is mainly generated by public utility companies and made available for purchase by domestic users and industries. 2.3.2 Carbonaceous Fuels This is any substance that may be burned rapidly enough in air during the process of combustion (oxidation) to generate heat capable of being applied in industrial operation. Examples of fossil fuels available for industrial use are coal, crude oil and natural gas, which are primary sources, and their products. Classification of Carbonaceous Fuels Primary fuels They are naturally occurring fuels obtained directly from the surface of the earth, its crust or from the sea e.g. Firewood, Coal and Crude Oil. Coal is a fossil fuel formed in ecosystems where plant remains were preserved by water and mud from oxidization and biodegradation, thus sequestering atmospheric carbon. Coal is a readily combustible black or brownish-black rock. It is a sedimentary rock, but the harder forms, such as anthracite coal, can be regarded as metamorphic rock because of later exposure to elevated temperature and pressure. It is composed primarily of carbon and hydrogen along with small quantities of other elements, notably sulfur. Coal is extracted from the ground by coal mining, either underground mining or open pit mining (surface mining). For coal to be qualified as a good coking coal it must possess the following properties: Volatile matter component should be 22-33 % to make it hard, strong and chemically unreactive coke that can withstand crushing in the furnace. 5 Ash content should be less than 10 % - as ash from coal is transferred to coke in coking. Sulphur content should be less than 2 % since excess sulphur remains with coke and readily associates with some metals present and its subsequent removal is expensive. Available fuel may not possess all these good qualities. However processes can be adapted to produce reasonably acceptable coke from inferior coals. Crude oil is a “fossil fuel” formed naturally from decayed animals and small plants living in ancient seas millions of years ago. As these animals die, they sink to the bottom of the sea where they mix with mud, sand, and clay. This mixture of mud and organic material is rich in hydrogen and carbon, the building blocks of crude oil. More mud and sediments were deposited on the sea floor over the years and over millions of years this layer of organic rich mud becomes buried thousands of feet deep in the earth. The temperature of the earth becomes hotter as you go deeper into the earth and the weight of all the mud and rocks above increase the pressure. This combination of increased temperature and pressure causes the organic material to change into crude oil. As the temperature increases the crude oil can be changed into natural gas. Crude oils vary in colour, from clear to tar-black, and in viscosity, from water to almost solid. It is mostly found in porous rock formations underground (once sea beds) in the upper strata of some areas of the Earth's crust, but can also be found above ground in oil seeps (oil sands) or tar pits (tar sands). Crude oil is the basis for engine oil, diesel oil, kerosene and other petroleum-based products as it is taken through fractional distillation to produce them. Chemical oil should have very low sulphur content, which is reflected by the price. Calorific values for oil are generally about 25 % higher than those of typical coal. Due to its high energy density, easy transportability and relative abundance, it has become the world's most important source of energy since the mid 1950s. Fossil fuels are non- renewable resources because they take millions of years to form, and reserves are being depleted much faster than new ones are being formed. Concern about fossil fuel supplies 6 is one of the causes of regional and global conflicts. The production and use of fossil fuels raise environmental concerns. Secondary fuel This class of fuel is artificially obtained from primary fuels e.g., charcoal, coke and kerosene. Coke is a solid carbonaceous residue derived from low-ash, low-sulfur bituminous coal from which the volatile constituents are driven off by baking in an oven without oxygen at temperatures as high as 1000 °C (1832 °F) so that the fixed carbon and residual ash are fused together. Metallurgical coke is used as a fuel and as a reducing agent in smelting iron ore in a blast furnace. Coke from coal is grey, hard, and porous and has a heating value of 24.8 million Btu/ton (29.6 MJ/kg). Some coke making processes produce valuable by-products that include coal tar, ammonia, light oils, and "coal gas". Petroleum coke is the solid residue obtained in oil refining, which resembles coke but contains too many impurities to be useful in metallurgical applications. Fuels may be sub grouped into solid, liquid and gaseous, examples of which are; Solid fuels – coke, coal, charcoal, firewood Liquid fuels – petrol, diesel, kerosene, crude oil, Gaseous fuels have easy distribution and easy control of composition and calorific value. Natural Gas Fuel - drawn from gas deposits in the earth normally in connection with hydrocarbons e.g., CH4 and Ethane. Artificial Gas Fuel – Oxyacetylene, coke oven gases (methane, H, CO), Blast Furnace gas (CO, CO 2, N), refinery gas (butane, propane), etc. Butane and propane can be liquefied with petroleum to produce bottled gas. Group Assignment 2.1 Discuss the environmental effects of secondary fuel production and suggest ways to avert its adverse impact on the environment. 2.3.3 Combustion This is used to define burning of fuel – oxidation of fuel at such a rate as to cause an appreciable rise in temperature (of several hundreds of degrees) in the substance involved in the process. The combustion of a fuel is a chemical reaction of the fuel or certain 7 constituents of it with oxygen, usually accompanied by a release of energy in the form of heat. The combustible elements are carbon, hydrogen, sulphur or their compounds. Note the following Definitions Flue Gases are the gaseous products produced after combustion. Ash is the non-combustible inorganic matter in solid fuel, which is the solid residue that remains after all the combustible, and volatile materials have been burnt off. It contains silicon, lime, ferric oxides, etc. Complete Combustion is the burning of a fuel in excess air in excess of up to 50 % of the theoretical air. Combustion can be complete or incomplete depending on the amount of oxygen or air available. Ignition Temperature is the temperature at which combustion begins. It depends on a number of factors, for example the ignition temperature of a solid fuel depends on particle size, nature of surface and porosity. That of a liquid fuel depends on the vapour pressure of the liquid and the proportion of vapour to air needed to form an ignitable mixture. With gaseous fuels, ignition temperature varies directly with concentration and decreases with increasing pressure. Moisture Content of a fuel is that matter content of the substance which can be removed by evaporation. Calorific Value is the heat resulting from a unit quantity (mass or volume) of fuel. It has a direct relation with the chemical composition of the fuel; the standard unit for heating values is now joules per kilogram or cubic metre. 2.3.4 Desirable Properties of Fuel a. Easy ignition b. High calorific value c. Suitable and controllable combustion rate characteristics d. Low and controllable impurity content e. Low smoke emission f. Availability g. Cost effectiveness 8 h. Uniformity in property and consistency. 2.4 Agglomeration It is a process by which fine grain materials are converted into coarser lumps. The comminution of ore to produce material of reasonable particle size for subsequent extraction processes inevitably lead to the production of too fine particles being part of the product, which needs to be reformed into bigger particles. These extremely fine particles are not desirable since in roasting, for example, they can block air spurges and also lead to caking and losses of dust-laden minerals. Also in leaching /hydrometallurgy, too much fines may lead to sliming, difficulty in percolation, problems in solid-liquid separation, etc. It is therefore necessary to agglomerate fines or bridge them to produce bigger aggregates. Agglomeration therefore is the process used in re-forming fine particles into larger lumps. There are four main methods. 2.4.1 Briquetting The feed is mixed well with water and usually binding agents. Binders may be inorganic - clay, lime, cement and metal salt, or organic – oil, tar pitch, etc. Before agglomeration, the material should have a certain amount of moisture content depending on the type of material and the binder. When lime or cement is used as a binder the briquette may be hardened at room temperatures (curing). When clay is used as a binder, the briquette is hardened at elevated temperature by firing (e.g. in a kiln). Briquetting may be done without a binder in which case the hardening is done by firing. It may also be done by first heating the substance to the required temperature and then pressing the hot material in the machine. Coal and Zinc ores may be agglomerated by briquetting. 2.4.2 Nodulising It is the conversion of fine grain materials into bigger lumps by heating the material in a rotary kiln to a temperature at which a partial fusion of the material starts. Nodulising is restricted to the agglomeration of metallic fines where there is insignificant amount of low- 9 melting gangue material constituents which tend to fuse into forming irregularly shaped nodules of low permeability. It therefore has limited applications. Low-grade manganese carbonate can be nodulised into high-grade manganese oxide. The kiln is inclined to help easy movement and it has liners or refractories, which helps to lift the material. Hot air Preheat ed air Feed Agglomerated material Figure 2.1 Nodulising 2.4.3 Pelletising Applied to a lot of iron ore concentrate. The fines are mixed thoroughly with a suitable amount of moisture and the binding agent usually clayey material such as bentonite. The mixture is rolled on an inclined drum, disc, etc. causing the ore particles to cling together and roll up into small spheres. Internal pores are reduced by mechanical interlocking which increase the strength of the ball. The ball may be hardened at room temperature (curing) or by heating (firing) between 650 and 13000C. Firing causes recrystallisation of the material and thus formation of stronger bonds. The heating may be done in a kiln / grate or oven firing it at around 1200oC (in duration) to harden. 2.4.4 Sintering This is similar to Nodulising but the material is stationary. This is done at a relatively higher temperature where partial melting and re-crystallisation cause the fine particles to adhere into bigger lumps. The fines may be mixed with some amount of coal, which will cause a self-sustaining burning on the surface. 10 2.5 Drying Drying simply means evaporation of water. It is a dewatering process normally to physical moisture content of zero. It is defined as the removal of mechanically held or physically combined water. Ideally minerals or the ore in mineral processing should be dry since crushing is essentially done dry. This is however not the case in real practical situations. Totally dry minerals are rare. Wet minerals sometimes are not desirable and they pose problems like choking, caking, corrosion especially where the percentage water is high. Moreover in some industries like the cement industries the entire comminution is done dry. Where mineral processing gives the end product for shipping, marketing essentially dry products decreases the weight and transportation cost. Also in the mineral processing labs, the ore is crushed and pulverised under dry conditions thus any solid sample that is received is dried to reduce the moisture content to zero. In the mining and mineral companies important calculations such as throughput, recovery etc, are based on dry mass thus samples are taken across shifts to determine the moisture content so as to get the actual (dry) mass of the ore processed. Drying can be achieved through; Exposing to sun and air. Heating the ore or substance above the normal boiling point of water at atmospheric pressure. Reducing the atmospheric pressure with respect to the vapour pressure of water at the temperature in question. Drying can be performed as a separate process e.g. in drying furnace or kilns or as an auxiliary process e.g. in the roasters wet ore charge is preheated and dried by flue gases before it gets to the actual reaction chamber. In drying a solid by evaporation, no fixed temperature is required except that the temperature of the source must be greater than that of the material. The higher the temperature the more rapid the evaporation; however the temperature must not be high enough to start any chemical reaction such as the roasting of sulphide particles or to cause any fusion of the solid. 2.6 Calcination 11 Calcination, also referred to as calcining is a thermal treatment process applied to ores and other solid materials in order to bring about a thermal decomposition, phase transition, or removal of a volatile fraction. The calcination process normally takes place at temperatures below the melting point of the product materials. It is more endothermic than drying. Calcination is to be distinguished from roasting, in which more complex gas-solid reactions take place between the furnace atmosphere and the solids, as calcination takes place in the absence of air. The process of calcination derives its name from its most common application, the decomposition of calcium carbonate (limestone) to calcium oxide (lime). The product of calcination is usually referred to in general as "calcine," regardless of the actual minerals undergoing thermal treatment. Calcination is carried out as separate process in furnaces or reactors sometimes referred to as kilns of various designs including shaft furnaces, rotary kilns, multiple hearth furnaces, and fluidized bed reactors and/or as an auxiliary process in roasters and smelters. Examples of calcination processes include the following: decomposition of hydrated minerals, as in calcination of bauxite, to remove crystalline water as water vapour; decomposition of carbonate minerals, as in the calcination of limestone to drive off carbon dioxide; decomposition of volatile matter contained in raw petroleum coke; heat treatment to effect phase transformations, as in conversion of anatase to rutile or devitrification of glass materials 2.7 Roasting Involves the heating of a substance or an ore below its fusion temperature in the presence of air to decompose the material or change the physical and chemical properties to make it suitable for subsequent process. It is usually a preparatory treatment of an ore for 12 subsequent leaching or smelting. Roasting in the auriferous industry involves the heating of complex gold ore containing sulphidic minerals mainly pyrite and arsenopyrite and carbonaceous materials in air to produce volatile arsenous oxide (As 2O3,), sulphur dioxide (S02), and carbon dioxide (CO2). The iron is also oxidised into hematite, which contains the gold and is porous enough to be leachable by cyanide. 2.7.1 Types of Roasting Processes Oxidising Roasting This is the replacement of sulphides with oxygen. Oxidising roasting is the most important of all the roasting processes as far as pyrometallurgy is concerned. E.g. 2ZnS + 3O2 → 2ZnO + 2S02 eq 2.1 In sulfide roasting, if the temperature and gas conditions are such that the sulphide feed is completely oxidized, the process is known as “dead roasting”. However if the roasting process is performed with less than the required amount of oxygen to fully oxidize the feed, it is called "partial roasting" because the sulfur is only partially removed. Reducing Roasting This is the heating of an ore or concentrate in contact with a reducing agent so as to convert it into metals or effect reduction in certain compounds. This is not common in modern times. E.g. ZnS + C + O2 → Zn + CO2 + SO2 eq 2.2 Chloridising Roasting This consists of heating the ore or concentrates in contact with chlorine (e.g. NaCl, HCl, Cl2, CaOCl2) to convert into chlorides, which may be leachable by water. E.g. ZnS + O2 + Cl2 → ZnCl2 + SO2 2MgO + C + Cl2 → 2MgCl + CO2 eq 2.3 Sulphating Roasting 13 This is a modification of oxidising roasting where temperature and gas conditions are controlled such that the sulphides in the feed react to form metal sulphates instead of metal oxides. Sulphates are more soluble in water. E.g. ZnS + 2O2 → ZnSO4 eq 2.4 Magnetising roasting This is a modified form of reducing roasting where haematite is reduced under controlled conditions to form magnetite to aid in magnetic separation but this is expensive for commercial purposes. Gravity method is rather used to concentrate Fe2O3 since it is heavy. Eg. 3Fe2 O3 + ½C → 2Fe3O4 + ½ CO2 eq 2.5 Sinter or Blast Roasting Sinter or Blast roast is just a type of oxidizing roast in which the end product is sintered and agglomerated as well. Material may be mixed with coal. 2.7.2 Common Methods of Roasting Hearth Roasting It is carried out in a multi-hearth system in which about 8-12 hearths are enclosed in the large cylindrical shell having diameter (6.1-7.6) and is about 9.14m high (Figure 2.2). Air enters from the bottom and is heated when it get into contact with the roasted material leaving the roaster. The ore enters at the top and drops from hearth to hearth while the sulphide ore particles are roasted as they come into contact with the rising gases. Often the first hearth serves as a dryer. In order to promote oxidation of the material in the roaster, the roaster is equipped with rotating rubble, which is attached to the central shaft. The rabble has arms, which serve to stir the charge as well as plough it towards the opening through which it drops to the next lower hearth. For example, on the first hearth, the rubbles plough through the material to push it towards the centre where there is an opening and hence allowing the materials to 14 fall on the second hearth. The rubbles here plough the material towards the periphery where there are opening allowing the material to fall on the subsequent hearth below. The vertical hearth furnace can roast between 100-200 tons of material per day. Heat can be supplied by fuel but usually the process is autogenous. It is cooled by sending cold air through the central shaft and the arms Figure 2.2 A Multiple Hearth Furnace (Huntington-Heberlein Type) Flash Roasting The concentrate, which is often wet, is dried on the first upper hearth or the second (Figure 2.3). It then falls through a combustion chamber where roasting takes place rapidly. 15 Auxiliary fuel is burnt in the unit to maintain the process temperature in a case where combustion is not autogeneous. After settling on the lower hearth the calcine (product) is collected and discharged from the roaster. Flash roasting requires that the ore or the concentrate be ground to fine size and as a result the dust lost on the roaster is likely to be high. A flash roaster has much greater capacity than hearth roaster of the same size because the materials are roasted rapidly in a flash. Figure 2.3 Flash Oxidation Fluidised Bed Roaster A fluidized bed reactor (FBR) is a type of reactor device that can be used to carry out a variety of multiphase chemical reactions. In this type of reactor, a fluid (gas or liquid) is passed through a granular solid material (usually a catalyst possibly shaped as tiny spheres) at high enough velocities to suspend the solid and cause it to behave as though it were a 16 fluid. This process, known as fluidization, imparts many important advantages to the FBR. As a result, the fluidized bed reactor (figure 2.4) is now used in many industrial applications. Figure 2.4 Fluidized Bed Reactor Blast Roasting In the multi hearth roaster the air circulate by natural draft and is not forced through the charge. In blast roasting (fig. 2.5) the charge to be roasted is held stationary and a current of air is drawn or blasted through it hence the name “blast furnace”. A certain amount of limestone is mixed with the roaster charge. The limestone serves to lower the temperature to a point where fusion from the charge could be avoided. This is achieved as a result of heat absorbed in heating and decomposing the limestone. The charge must be coarse enough to prevent channelling and increase permeability of air through them. The roasted 17 material is removed by means of rotating disc or a horizontally moving spiral. It is 60 meters high and can roast about 10 000 tons of material per day. At present Dwight–Lloyd process which is an improved version has replaced the Blast roasting and it is called Sinter Roasting. Figure 2.5 A Vertical Furnace – the iron blast furnace 2.7.3 Factors to Consider In Ensuring Well Roasted Products Particles should be relatively small to increase rate of reaction (it should however not be over ground). The correct temperature should be ensured and maintained to prevent melting and formation of unwanted product. The charge should have the right composition and good contact with the hot air. 18 2.7.4 Purpose of Roasting To convert sulphides to sulphates (Sulphating) To convert sulphides to oxide (Oxidation) To convert sulphides to chlorine (Chloridising) To convert sulphides to metals (Reduction) All these are done to effect liberation and to make the ore amenable to leaching or other subsequent processes. 2.8 Smelting It is a metallurgical process of melting a rock normally under reducing conditions to reduce some of the metallic oxides and at the same time to produce two main immixable fluids; molten metal and slag. Separation is thus effected in a melt i.e. when the metal is in the molten form. A smelting operation may yield 4 products – molten metal, matte, speiss and slag which separate partially into layers with increasing specific gravity as Slag (silicate S.G. = 3.6) Matte (sulphide S.G.= 5.2) Speiss (arsenide S.G.= 6.0) and the impure metal or bullion. The material to be smelted is charged in the solid state but the product from a smelter is in the liquid state. The only solid material that escapes is the dust carried out in the furnace gases. There are two important types of smelting; reduction and matte smelting. 2.8.1 Reduction Smelting It takes place strictly under reducing conditions or in contact with a reducing agent so as to reduce the mineral of interest into a metal. It results in molten metal and slag. 2.8.2 Matte Smelting This is a method of smelting of sulphide ores which results in molten metallic sulphides and slag. Molten matte is a solution of metallic sulphides with high electrical conductivity, low melting point and high specific gravity. It contains some oxides (impurities) and thus requires recleaning or refining. It is normally an intermediate product in the extraction of polymetallic ores. For example, Fe-Cu-Matte is the intermediate product in the extraction 19 of Cu from chalcopyrite (FeCuS2). It is also the standard treatment of the concentrate of Cu - Ni sulphide. [Cu –Ni – matte (20 – 70 % Cu, 20 – 25 % S, and the rest is Fe)]. Antimony could be treated in this way (only when it becomes necessary). This is carried out in the reveberatory furnace. Shaft or blast furnace can be used but in copper and nickel, the most commonly used one is the reveberatory. Blast is used for lead and iron. Smelting may be conducted in a blast furnace, reverberatory furnace or electric furnace. Reduction smelting is usually carried out in blast furnaces and matte in reverberatory but there are exceptions in both cases and each type may be performed in an electric furnace. The necessary heat for smelting may be supplied from fuel or electricity or the process may be autogeneous. 2.8.3 Fluxes This is normally added to the smelting process in order to give the slag the required properties. A flux is a material, which is added to a furnace charge, and it has the primary duty of reacting with the oxidised portions (impurities) to form a fluid slag. It also lowers the melting point of the slag forming constituents so they can melt easily and float. The slag forming impurities must have a higher affinity for the flux than the metal and thus dissociate from the metal. The composition and amount of flux to be added therefore is determined by the impurities and thus the slag envisaged. Examples of fluxes are lime, magnesia, and silica. Flux can be grouped into acidic, basic and neutral. Acid fluxes are used for basic impurities and vice versa, whilst neutral gives more fluidity. Some of the common fluxes used are: Lime, magnesia iron, steel making Fluorspar steel making Silica copper smelting Borax copper smelting Heamatite/Pyrite Lead smelting For smelting operations, silica is used to flux metal oxides and calcium or iron oxide for fluxing siliceous material. 20 2.8.4 Slags During smelting the bottom layer of the smelted material is the molten metal or matte or speiss. Slag is the substance that forms the top layer after smelting. It is glasslike in nature and thus can be hammered off. It normally contains siliceous or oxide materials (e.g. SiO 2, Al2O3, CaO, FeO and sometimes phosphates, borates, aluminates and sulphates) supposed to be unwanted in most cases but not all, e.g. Antimony, and in the smelting of iron for example, high limestone content in the slag can be used to produce clinker or Portland cement. Generally slags are commonly black, brown or dark green in colour, the colouration is largely due to the presence of oxides of iron. Functions of a Slag Receptacle for gangue and or reduced oxides in primary extraction (remove impurities). Prevents matte and molten metal from oxidation to retain some sulphur for refining. Prevents heat loses from the crude metal. In refining process, the slag serves to absorb all the extracted impurities. In the electric smelting furnace the slag could be used as a heating resistor. Properties of Slag Melting temperature: should have a proper melting point; and should melt at that temperature. Specific Gravity: should have low specific gravity as this aids separation. Example Magnesia slag (S.G = 2.0) and Lead (S.G = 3.6). Low viscosity: some valuable metals suspended in the slag will be able to sink into the molten metal if the slag is fluid i.e. less viscous. Low solubility for metal or matte: molten metal should not be dissolved in the slag. 2.9 Refractories A refractory is a material that retains its strength at high temperatures. It is defined as materials having those chemical and physical properties that made them applicable for 21 structures or as components of systems that are exposed to environments above 1000 0F (538 0C). Refractory materials are used in linings for furnaces, kilns, incinerators and reactors. They are also used to make crucibles. Refractory materials must be chemically and physically stable at high temperatures. Depending on the operating environment, they need to be resistant to thermal shock, be chemically inert, and/or have specific values of thermal conductivity and of the coefficient of thermal expansion. Refractories must be chosen according to the conditions they will face. Some applications require special refractory materials. Zirconia is used when the material must withstand extremely high temperatures. Silicon carbide and carbon are two other refractory materials used in some very severe temperature conditions, but they cannot be used in contact with oxygen, as they will oxidize and burn. Examples of materials used in making refractories include quartzite, sandstone, kaolin, bauxite, forsterite, magnesite, dolomite and graphite. 2.9.1 Factors Affecting the Choice of a Refractory Price Overall cost of purchasing and maintaining a refractory material should be reasonable. The important thing is not the initial cost but the cost per unit weight of the material treated: one refractory material may cost more than another but if it has a longer life it may be cheaper in the long run to install it rather than a refractory with a lower material cost. Melting Point Melting point of refractory must be in excess of the substance being heated so as not to fuse at the operating temperature. Strengths (mechanical & physical) Must be strong enough to maintain its dimension and rigidity and withstand wear due to feeding under elevated temperature. Through abrasion the surface may wear when feeding, 22 air coming out under pressure may also affect it. Feeding and pouring is continuous as the refractory material is still being heated – become soft so it must be strong enough. Chemical Composition Refractories can soften due to some slag or flue gases, which contain a lot of different types of material, which come into contact with the refractory material, enter the pores and soften it. Therefore there should be no such reaction. If a refractory is in contact with a molten slag or has dust laden gases impinging on its surface, it must not react chemically with these substances. Acid refractories will resist the action of acid (siliceous) slag and dust. Basic refractory will withstand the chemical action of basic slag and dust. Thermal conductivity The thermal conductivity must be low to minimize heat losses from furnace walls. That is it should be able to retain the heat within the furnace so as to conserve energy and protect outer shell (steel). Electrical Conductivity This property is only important when smelting in an electric furnaces. Graphite and the metals are the only good electric conductors among the refractories: others are all insulators. Graphite is a very good refractory material and used for electrodes and furnace lining in all high temperature electric furnaces. Metals are not important as refractories in electric furnaces but copper wires for e.g. or bus bars are used to bring the current to the graphite electrodes. These metallic conductors are often water-cooled. Expansion / Thermal Shock Refractory bricks should have a small coefficient of expansion. This is important because the refractory expands if the furnace is brought up to temperature (operation temperature) and provision must be made for this in the furnace design. If a refractory is subjected to repeated heating and cooling (thermal shock) it may spall (break apart). Spalling can be prevented by careful operation to avoid sudden temperature changes. The tendency of a refractory to spall can be minimised if proper methods are used in its manufacture. 23 Porosity Since most refractories are made by firing mixtures of solids, the formation of pores between the solid grains can not be prevented. The porosity should be kept at a minimum, since all the refractory properties (e.g. strength, thermal shock resistance) of the brick improve when the porosity is decreased, except in the case of insulating refractories. Resistance to Chemical Attack Most of the oxide or silicate refractories are already fully oxidized so that they will not be affected by oxygen. However, graphite and silicon carbide will oxidize at high temperature and actually will burn. Chemical attack usually results from the contact of acid and basic refractories, through slag or dust. With acid slag (i.e. slag high in silica) acidic refractories and with basic slag (i.e. high in CaO, MgO) basic refractories should be in contact. The product of the chemical reaction has a very low melting point (forms a eutectic). For the same reason acid and basic refractories should not be laid side by side in the heated portions of the furnace. Bricks may have to withstand abrasive damage or erosion by fast moving, dust-laden gases. Softening Point It is the temperature at which, the refractory is plastically deformed under load. The selection of the refractory for a given service temperature should take the softening point into consideration not the melting point of the refractory. 24 CHAPTER THREE HYDROMETALLURGY 3.1 Introduction Hydrometallurgy is a branch of extractive metallurgy that extracts metals from ores by aqueous methods. In hydrometallurgy, a condition is developed that helps to extract the mineral of interest leaving the gangue mineral by making use of a suitable extractant (or solvent). It involves three (3) processes or steps namely; Leaching (e.g. Agitation, Heap, Dump and In-situ Leaching) 25 Solution Purification (e.g. Adsorption, Ion exchange, Ion flotation, Solvent extraction and Precipitation) Metal Recovery (e.g. Crystallization, Adsorption, Electrolysis and Precipitation) 3.2 Leaching It is the process of selectively dissolving a desired soluble constituent (e.g. gold) from a solid material (e.g. ore, concentrate, calcine, matte) by means of a solvent (lixiviant) to produce a concentrated pregnant solution from which the dissolved metal can be recovered. Ore + Lixiviant Pregnant Solution (Mainly valuable mineral) Leaching Residue (Mainly gangue material) Figure 3.1 Leaching The pregnant solution contains some impurities that have been co-leached and there is the need to get rid of them through solution purification to obtain a pure solution from which the metal can be recovered. 3.2.1 Leaching Reagents or Lixiviants Lixiviants are salts used in mineral dissolution. Examples of these are: Water: for leaching common salt, calcines from sulphide roasting or chloridised roast. In lead sulphide ores water can leach sulphide under high pressure to produce sulphates which act as lixiviant for leaching lead at high temperature. NaCN: To leach Au and Ag in a complex mediated oxidising leaching. NaCO3: To leach Uranium. Acidified ferric sulphate: To leach sulphides especially copper sulphides. NaCl: To leach lead sulphate. 26 Dilute H2SO4: To leach oxidised Cu ores and MnO2. NaOH: To leach bauxite. Ammonia: To leach Cu ores; mostly used when acid consuming gangues are present. Though NH3 has high vapour pressure it leads to losses and forms complex with copper (Cu). 3.2.2 Choice of Lixiviant Depending on the chemistry of the material to be leached in association with the leaching methods various reagents could be used in the leaching of ores. The choice of reagents depends on the following: Chemical and physical nature of the material to be leached. Selectivity; the reagent should dissolve only the mineral of interest and not the gangue. Corrosive nature of the reagent; it should not be so corrosive to attack the tanks and other accessories. Toxicity; it should not be toxic. However, if its vapour pressure is low and toxic effects can be controlled, then it could be acceptable. Stability; the reagent should not break down or polymerise. Cost; it should not be too expensive. It should be cost effective e.g., both cyanide and thiourea can be used to leach gold. Thiourea is not poisonous but its price is about ten times that of CN and its concentration is 2 kg/ton as against 0.5 kg/ton of CN. Regeneration; it should have the ability to go through restoration and re-use. This helps cut down cost and decrease environmental impact. Viscosity; aqueous solutions of the reagent should be able to flow freely. Viscosity modifiers may be used if the slurry does not respond well. Capacity; The reagent should have a high capacity, that is, a small amount should be able to leach large quantities of ore. Salts used in leaching may be that of a weak base and strong acid or a strong base and a weak acid. Such salts hydrolyse in aqueous media. To counterbalance the effects of hydrolysis, pH modifiers are introduced. For example sodium cyanide is the salt of a strong 27 base and weak acid and undergoes hydrolysis to produce HCN, which aside leading to solution losses, is a very toxic gas. Studies show that in moist air or aqueous solution more than 50 % of NaCN exist as HCN gas below the pH of 9.5. To prevent this from taking place, a pH modifier (lime or caustic) is added. NaCN + H2O NaOH + HCN very poisonous Some minerals like gold cannot dissolve in the absence of oxygen or other oxidising agents. For such minerals hydrogen peroxide or oxygen (may be from the air) should be introduced during leaching. 3.2.3 Factors Affecting Leaching Rate The following factors have been found to influence the rate of leaching: Pulp density: Rate of leaching increase with decreasing pulp density. Particle size: Generally the finer the particle size, the higher the surface area exposed for contact and thus the faster the leaching rate. Coarse particle take longer time to leach. Howbeit, over-grinding must be avoided since it produces large amount of fines that complicate solid liquid separation; gangue material is also activated when ground too fine. Over-grinding also increases grinding cost and thus milling cost. Temperature: Generally, leaching rate increases with increase in temperature. It is a linear increase, anyway since leaching reaction is diffusion controlled. Excessive temperatures should however be avoided since it may lead to co-leaching of gangue minerals. Pressure: Leaching could be carried out under atmospheric or higher pressures. Under atmospheric conditions it is termed open leaching, otherwise it is pressure leaching. Higher pressures increase rate of leaching. 28 Concentration: Rate of leaching increases with increasing concentration of lixiviant at constant feed rate. However, very high concentrations lead to co-leaching of gangue materials. This causes purification problems. Agitation: The primary aim of agitation is to keep the particles in suspension and expose them to the solvent as much as possible. It also increases the diffusion rate by decreasing the interfacial boundary layer. 3.2.4 Leaching Technology The leaching process can be a continuous or a batch process. For Batch Leaching, the material is allowed enough time to leach completely before it is discharged for separation. It is relatively expensive and is normally used in the lab for test work, e.g., bottle rolling. For Continuous Process, leaching is done in series where an ore in one tank is leached to a certain extent before it enters another and by the time it gets to the last tank the mineral is considered to have been leached to a level where separation can be done. It is relatively cheaper and used for large quantities of high-grade ore. In practice ores are leached in two ways; choice of leaching method is closely linked with the grade of ore and the rate of dissolution of mineral values in the lixiviant. Agitation leaching This deals with high grade ores and is used where finely divided ore is mixed with water and agitated in a suitable vessel. The agitation is done by mechanical impellers or pneumatic means these help to suspend the mineral particles thus exposing them to the influence of the lixiviant. The leaching time here is shorter; it can be 16-36 hours. Non-agitation leaching It deals with low-grade ores. These systems are fixed bed reactors (heap, vat, dump, in- situ), where lixiviant is made to leach the ore by percolation. It is important that the ore is permeable to the lixiviant, and the technology cheap for the process to be viable. 3.2.5 Types of Non-Agitation Leaching 29 In-situ leaching, Solution mining or leaching in place Pregnant. Lixivian solution t Impervious roc Ore body Figure 3.2 (porous) Leaching in Place The grade of the ore is too low to justify mining and transportation expenses. It is thus simply shuttered and left in place. Lixiviant is pumped into the ground to leach the ore, whilst pregnant solution is pumped out. It is an important requirement that the underlying rock be of impervious nature. Leaching time may be in years. Dump Leaching The grade here is too low to justify crushing expenses, though higher than in-situ leaching. The ore is mined transported and dumped (without crushing) on a suitable site (impervious pad); lixiviant is sprinkled to leach the ore. This may take months to years. Heap Leaching The grade of the ore is too low to justify grinding expenses. The ore is mined, crushed to suitable size and heaped on an impervious terrain sloped at an angle of about 7 0 to ensure flow under gravity. Lixiviant is sprinkled on the heap to percolate through the ore and leach metal values. The pregnant solution is collected at the base for subsequent purification and metal recovery. The solution collected may be circulated several times to increase the 30 grade or get the required grade for purification and/or recovery. The ore must be porous to ensure proper dissolution and extraction of mineral values. Figure 3.3 Heap Leaching The fines produced should be screened off in non-agglomeration leaching or agglomerated (agglomeration heap leach). This is done to ensure proper percolation through the heap, avoid retention and channelling of lixiviant and offset filtration of too much fines in pregnant solution. Once agglomerated, curing time of up to about 72 hours is allowed. It can take up to about 40 days. Heaps can be several meters in length and about 50 m high. Heap leaching is cheaper – there is no filtration, needs less attention – few people can man it, it has higher retention time due to several cycles, low recovery – 70-80 % about, high losses, retention of values in voids due to high capillary action. Leach Pad Design Firm ground to withstand the weight of material. Removal of top soil and vegetation (kept for re-vegetation) Grading, filling and compacting of the ground. Inclination lengthwise and sideways. Uniform strength of the entire area – avoid points of stress concentration and tearing of layer. The prepared floor is laid with impermeable layer, asphalt, concrete, compacted clay or HDPE. In the case of HDPE – joints must be welded. The pad may be double to reduce seepage during perforation. Example Asphalt Drain 31 Clay The pad is normally divided into cells each of which has independent drainage systems so it can be diverted to the appropriate pond depending on concentration. There is a pond system built below the heap and is also lined with HDPE. It serves as the collection point for solutions to and from the heaps (pregnant solution pond – to recovery unit, barren solution pond which receives tails from the recovery unit, intermediate pond – for reconditioning and re-spraying, overflow pond – excess solution from other ponds). The intermediate ponds are supposed to receive pregnant solution with low gold values and also barren solution, for conditioning and sprinkling. One intermediate pond should have a higher concentration of cyanide after reconditioning. The higher concentrations are used in spraying fresh heaps while the lower one is for old heaps. Types of Leach Pads Reusable pad – Exhausted ore is unloaded for disposal and fresh ore heaped on pad. Valley-Leach Method – The ore is heaped behind a retaining wall built in a valley. Expanding pad – Exhausted heap flattened and fresh ore added, or abandoned and new pads prepared for fresh ore. Vat Leaching This is similar to heap leaching, but in this case the crushed ore is submerged in large tanks filled with lixiviant. The ore must be porous and uniformly sized. Fines and slimes must also be checked. Figure 3.4 Vat Leaching Tank 32 Advantages of Vat Leaching To reduce leaching time, increase gold extraction and gold content of the pregnant solution. Smaller plant site per ton of ore Closer metallurgical control Lower capital and operating cost 3.2.6 Agitation Leaching This is used for High-grade ore Fast leaching ore (can take 24 hrs) Pre-treated ore Slimy ore The high-grade ore is mined, crushed, ground finely to liberate the leachable ore. The pulp is conditioned and pumped into a suitable vessel for leaching under agitation. This type is referred to as open leaching with 100oC as the maximum attainable temperature as a result of inter-reaction with atmospheric pressure. Agitation is done to suspend mineral particles and expose them to the influence of the lixiviant. The type practiced in the mine is the continuous leaching where the ore move from one tank to another as leaching goes on. Figure 3.5 Agitation Leaching of Gold Ore 3.2.7 Pressure Leaching 33 This is a special case of agitation leaching used normally for ores like sulphide minerals (asenopyrite, pyrite, etc) that require high temperatures and pressures. Pressure leaching is done in enclosed vessels, which can retain heat and built-up pressure. Example is the autoclave – steel vessels with very thick refractory walls to withstand high pressure and temperature. Though the reaction is faster than open leach, it is relatively expensive due to the need to set up equipment to take care of too high temperature and pressure. The grade of the ore does necessarily have to be high enough to pay for the expenses to make profit. Autoclaves are lined with anti-corrosion materials like Pb since H2SO4 acid is produced and heat resistant material like titanium all of which make the process expensive. Figure 3.6 Pressure Leaching of Sulphidic Gold Ore 3.3 Solution Purification / Metal Recovery After leaching, the pregnant solution containing about 2 – 15 ppm and 1ppm from agitation and non agitation leaching respectively is purified to get rid of unwanted substances that were co-leached. The extent of purification will depend on the level of impurities present in the solution. The pregnant solution can also be purified (concentrated further) to increase the grade. 3.3.1 Adsorption This is the concentration or purification of ions or molecules of mineral of interest in a liquid phase onto a substance in a solid phase. The solid phase is called adsorbent and the liquid phase is called the adsorbate. Being a surface phenomenon, the rate of adsorption 34 depends on the surface area of the adsorbent (solid; e.g. activated carbon, Biomass and inorganic materials like clay, zeolite etc). There are three types of adsorption: Exchange adsorption – This is as a result of electric attraction between adsorbate (liquid or component) and adsorbent due to difference in charges. The higher the charge, the greater the attraction. Physical adsorption – This is due to weak forces or Vander Waal forces between molecules. The position of adsorbed molecule is not fixed and it can be multi- layered. It is also reversible (can be desorbed). Chemical adsorption – This is due to much stronger forces like chemical bonds. Adsorbate and adsorbent surfaces share electrons. It forms a monolayer and is irreversible. 3.3.2 Crystallization This is the formation of solids from solution, melt, vapour or a different solid. It may be done in one step or multi step depending on purity. The driving force for crystallisation is super saturation-point of minimum dilution, of the solid to be formed in the solution. Steps involved in crystallization include super saturation, nucleation and crystal growth. Nucleation is the formation of new crystals or crystal nucleus. Homogeneous nucleation occurs in the absence of foreign solid, container wall and at high degree of super saturation. Heterogeneous nucleation occurs with the help of foreign solids or pseudonucleus (false nucleus) and container wall, present at moderate degree of super saturation. These act to adsorb the solute particles forming the nucleus. Crystal growth is the addition of new layers to the already formed crystal. Crystallisation can be induced by evaporation, reduction in temperature, salting, etc. Examples of crystal growth include monolayer, polynuclear layer thus imperfections in the crystal surface and aggregative growth. In solution, crystallization may be induced by evaporation or cooling depending on the salt’s behaviour in water at various temperature or solubility reduction solvents may be used. Crystallisation from melts or molten substances is done by cooling normally in a process called solidification. Fractional crystallization is used to separate several solutes 35 present in the same solution. Nucleation inhibitors are used in case two substances have the same level of super saturation. Seeds of desired solute may also be introduced to induce nucleation. 3.3.3 Solvent Extraction This is a process of solution purification, concentration or upgrading involving a phase transfer from an aqueous phase to an organic phase. Mn+ can then be transferred from the organic phase to an aqueous phase through elution. It involves the extraction of a component of a solid by contacting with another liquid/solution (mainly organic extractant), which should be selective for the ion of interest. For example DIBK in the gold industry is used for the extraction of gold from aqueous pregnant solution. The mother solution must not mix with the extractant. Organic Extractant M n+ Concentrated Organic + Solution Mn+ Pregnant solution Barren of Mn+ Figure 3.7 Solvent Extraction 3.3.4 Ion Exchange 36 This is a purification method used to concentrate or remove metal ion from solution by an ion exchange resin. The resin is a highly insoluble polymeric and tightly cross-linked structure containing fixed polar ionic groupings as an integral part of the polymer structure. The ionic groupings has a diffusible component which goes into solution and exchange for the metal value when the resin ionise in solution. Another application can be found in water softening. When zeolite made up of sodium aluminate silicate is introduced into water, calcium or magnesium ion present in the water is exchanged with sodium ions in the zeolite (resin). At the end of the process, the calcium or magnesium ions will attach themselves on the resin and be removed later. This way the water is softened. 3.3.5 Ion Flotation It is the use of anionic or cationic surfactant to react with metal ions in solution to form an insoluble metal compound or soap that can be levitated to the surface by blowing air through the substance. The collector is added in stoichiometric amount in relation to the metal content in the solution. Iron flotation is a new tool, which is finding a wider application in environmental management in the treatment of effluent and wastewater to remove waste. 3.3.6 Cementation This is the process of precipitating a metal ion in solution by the use of another metal. The precipitated metal cements on the foreign metal. This reaction is electrochemical and works on the principle that metals with more positive electro-potential or oxidation will pass into solution and cement a metal ion with a less positive potential. For example finely divided copper is displaced by an iron scrap and cadmium can also be displaced by zinc as shown; Cu 2 + + Fe → Cu o + Fe2 + OHC Fe → Fe2 + + 2e RHC Cu 2 + + 2e → Cu o Cd ++ + Zn ° →Cd ° + Zn ++ 37 CHAPTER FOUR ELECTROMETALLURGY 4.1 Introduction Electrometallurgy is the branch of electrochemical engineering, which deals with the extraction and refining of metals from aqueous and molten solutions. It uses the medium of electricity and is based on the principle of electrochemistry. 4.2 Electrochemistry This is the study of the interconversion of electrical and chemical energy. This conversion takes place in an electrochemical cell that may be A Voltaic cell, in which spontaneous reaction generates electrical energy. Electrolytic cell, in which electrical energy is used to bring about a non- spontaneous reaction. All of the reactions considered in this chapter are of the oxidation – reduction type which can be split into two half-reactions. In one half-reaction, referred to as reduction, electrons are consumed. In the other called oxidation, electrons are produced. There can be no net charge in the number of electrons. The number of electrons consumed in reduction must be exactly equal to the number produced in the oxidation half-reaction. In electrochemical cell, these two half-reactions occur at two different electrodes, which most often consist of metal plates or wires. Reduction occurs at the cathode; a typical half-reaction might be Cathode: Cu 2+ (aq) + 2e − → Cu ( s ) 38 Oxidation takes place at the anode, where a species such as zinc metal produces electrons: Anode: Zn( s ) → Zn 2+ (aq) + 2e − It is always true in an electrochemical cell that, anions move to the anode; cations move to the cathode. One of the most important characteristics of a cell is its voltage, which is a measure of reactions spontaneity. Cell voltages depend on the nature of the half-reactions occurring at the electrodes and on the concentrations, it is possible to calculate the standard free energy change and equilibrium constant of the reaction involved. The principles discussed in this chapter have a host of practical applications. Whenever you start your car, turn on your cell phone, or use a remote control for your television or other devices, you are making use of a voltaic cell. Many of our most important elements, including hydrogen and chlorine are made in electrolytic cells. 4.3 Electrolytic Cells In an electrolytic cell, a non-spontaneous redox reaction is made to occur by pumping electrical energy into the system. A generalized diagram for such a cell is shown in figure 4.1. The storage battery at the left provides a source of direct electric current. From the terminals of the battery, two wires lead to the electrolytic cell. This consists of two electrodes, A and C, dipping into an electrolyte containing ions M+ and X-. An electrolyte is a substance which in solution or fused state, dissociates into positive and negative ions that is it is ionised. The solution, as a result of the dissociation has the ability to conduct an electric current. e- e- Anode Cathode C A M+ X- 39 Storage battery Figure 4.1 Electrolytic Cell The battery acts as an electron pump, pushing electrons into the cathode, C, and removing them from the anode, A. To maintain electrical neutrality, some process within the cell must consume electrons at C and liberate them at A. This process is an oxidation-reduction reaction; when carried out in an electrolytic cell, it is called electrolysis. At the cathode, an ion or molecule undergoes reduction by accepting electrons. At the anode, electrons are produced by the oxidation of an ion or molecule. 4.3.1 Quantitative Relationships There is a simple relationship between the amount of electricity passed through an electrolytic cell and the amount of substances produced by oxidation or reduction at the electrodes. From the balanced half-equations; Ag + (aq) + e − → Ag ( s ) Cu 2+ (aq) + 2e − → Cu ( s ) Au 3+ (aq) + 3e − → Au ( s ) You can deduce that 1 mol of e- 1 mol of Ag (107.9 g of Ag) 2 mol of e- 1 mol of Cu (63.55 g of Cu) 3 mol of e- 1 mol of Au (197.0 g of Au) Relations of this type, obtained from balanced half-equations, can be used in many practical calculations involving electrolytic cells. You will also need to become familiar with certain electrical units, including those of; Quantity of electrical charge: The common unit here is coulomb, C. The coulomb is related to the charge carried by a mole of electrons through the Faraday constant: 1 mol electrons = 9.648 × 104 C Rate of current flow: Here the common unit is ampere, A. When a current of one ampere passes through an electrical circuit, one coulomb passes a given point in the circuit in one second. That is, 1 A = 1 C/s 40 Amount of electrical energy: This can be expressed in joules, J. When a charge of one coulomb moves through a potential difference of one volt (V) it acquires energy of one joule: 1 J = 1 C V Table 4.1 Electrical units Quantity Unit Defining Relation Conversion Factors Charge coulomb (C) 1 C = 1 A s = 1 JN 1 mol e- = 9.648 × 104C Current ampere (A) 1 A = 1 C/s Potential volt (V) 1 V = 1 J/C Power watt (W) 1 W = 1 J/s Energy joule (J) 1 J = 1 V C 1 kWh = 3.600 ×106J Assignment 4.1 Chromium metal can be electroplated from an aqueous solution of potassium dichromate. The reduction half-reaction is; Cr2O72-(aq) + 14H+ (aq) + 12e- 2Cr(s) + 7H2O A current of 6.00 A and a voltage of 4.5 V are used in the electroplating. (a) How many grams of chromium can be plated if the current is run for 48 minutes? (b) How long will it take to completely convert 215 mL of 1.25 M K2Cr2O7 to elemental chromium? (c) How many kilowatt hours of electrical energy are required to plate 1.00 g of chromium? In working assignment 4.1, we have in effect assumed that the electrolyses were 100% efficient in converting electrical energy into chemical energy. In practice, this is almost never the case. Some electrical energy is wasted in side reactions at the electrodes and in 41 the form of heat. This means that the actual yield of products is less than the theoretical yield. 4.3.2 Electro-Winning It is the electrolytic extraction of metal from an electrolyte containing metal ions by the passage of current (Figure 4.1). The metal may be electrowon either from aqueous solution or fused salts and the electrodes may be active or inert. Metals like aluminium, sodium, potassium and magnesium are very electropositive and are electrowon from fused salts. It is primarily the reduction of metal to zero oxidation state. Ions in solution or fused state are deposited as metals on the cathode. Figure 4.2 Electro-Winning Cell 4.3.3 Electro-Refining It is the process of refining an impure metal by electrolytic methods. The impure metal is made the anode of the cell while a pure strip of metal is made the cathode (Figure 4.3). The impure material dissolves and the pure ions migrate to, and deposit on the pure strip. The electrolyte should be of high electrical conductivity and constant concentration. 42 Figure 4.3 Electro-Winning Cell During the electro refining of metal, the potential is adjusted to cause the dissolution of the metal of interest alone. Thus metal impurities with higher reduction potentials would not go into solution but rather fall to the bottom of the container as anode mud or sludge. Those having a lower reduction potential will dissolve and move to the cathode along with the metal of interest but the potential at the cathode is set so as not to favour the deposition of these metals. Thus only the pure metal of interest will be deposited. 4.3.4 Electro-Plating It is the process of depositing a sound metallic coating with the required characteristics onto a conducting surface by means of electrolysis (Figure 4.4). It is carried out by immersing the item to be plated in solution of the metal to be plated on it and passing a direct current between the part and another electrode to produce a thin smooth sound metallic deposit. The article to be electroplated should thoroughly be cleansed of grease and dirt by dipping it in acid and alkaline cleaning solutions. The character of the deposit depends on many factors including temperature, current density, time and composition of bath. Others are type, nature and surface preparation of substrate. Electroplating can be used to improve the nature of metallic and non-metallic materials. It may also be used to improve the abrasion resistance of steel balls, restore worn out parts and to provide protection against corrosion. 43 Figure 4.4 Electro-plating Cell CHAPTER FIVE EXTRACTIVE METALLURGY OF GOLD 5.1 Introduction Gold is a soft, dense, bright yellow metallic element. It is one of the transitional elements of the periodic table with atomic number of 79. Gold is found in nature in quartz veins and secondary alluvial deposits as a free metal or in a combined state. It is widely distributed although it is rare, being 75th in order of abundance of the elements in the crust of the Earth. It is almost always associated with varying amounts of silver; the naturally occurring gold-silver alloy is called electrum. Gold occurs, in chemical combination with tellurium, in the minerals calaverite and sylvanite along with silver, and in the mineral nagyagite along with lead, antimony, and sulphur. It occurs with mercury as gold amalgam. It is 44 generally present to a small extent in iron pyrites. Galena, the lead sulphide ore that usually contains silver, sometimes also contains appreciable amounts of gold. It is one of the softest metals and is a good conductor of heat and electricity. Gold is extremely inactive; it is unaffected by air, heat, moisture, and most solvents. It will, however, dissolve in aqueous mixtures containing various halogens such as chlorides, bromides, or some iodides. It will also dissolve in some oxidizing mixtures, such as cyanide ion with oxygen, and in aqua regia. The chlorides and cyanides are important compounds of gold. Gold melts at about 1064 °C, boils at about 2808 °C, and has a relative density of 19.3 with atomic weight is 196.967. The properties of gold that are exploited in its recovery are: Solubility in acidic and alkaline solutions in relatively high oxidising environments Metallic yellow colour High specific gravity Ability to form an amalgam with mercury The extractive metallurgy of gold focuses on the various types of gold ores and the respective beneficiation processes used for winning the gold metal. 5.2 Classification of Gold Ores in Ghana Based on metallurgical processes, gold ores may be broadly classified as alluvial ores, free milling ores and refractory ores. The technique employed in extracting gold in Ghana and the associated environmental problems vary from mine to mine depending on the type of ore being treated. Gold particles are naturally liberated in alluvial ores and thus can be recovered by gravity concentration without undergoing comminution. Alluvial gold deposits occur in streams, riverbeds and terraces. These deposits are derived from primary hard rock deposits that have undergone several cycles of erosion and deposition. Alluvial ores are abundant in Ghana and can be found in and along the banks of Ankobra, Offin, Tano, Pra and Birimian River systems. 45 Free milling ores are those from which about 95 % of the gold can be recovered by simple direct cyanidation when the ore is milled to 80 % passing 75 m. Gold ores in the Tarkwaian system of Ghana can be referred to as free milling ores. The minerals associated with gold are basically oxides. Some of the major oxide minerals include silica, hematite, magnetite and illmenite. The ores are termed refractory when a significant proportion of the gold cannot be recovered by conventional gravity concentration or direct cyanidation. In Ghana refractory ores are found in many areas including Obenemasi, Obuasi, Bogoso, and Prestea. 5.3 Gold Beneficiation Processes The various beneficiation processes used in extracting gold from the various ore types found in Ghana are discussed in the following sections. 5.3.1 Alluvial Ore In Alluvial ore, gold exists as discrete particles, usually, not associated with the gangue. It is naturally liberated and thus concentrated by gravity method. Alluvial ores require no comminution because the gold is already liberated. Recovery involves a pre-treatment step in which the ore is washed and screened. The through screen product which usually contains the gold is then subjected to gravity separation. Depending on the gold particle size range, a centrifugal concentrator or a sluice board may be used. The gravity concentrate may be smelted directly after cleaning on a secondary concentrator or after amalgamation and retorting to get the bullion. It may also be leached by intensive cyanidation. Figure 5.1 shows the sample flow-sheet for the treatment of alluvial gold ores. 5.3.2 Free Milling Ore In free milling gold ore, gold is found in quartz vein mainly along sheared zones and can be liberated by comminution (see Figure 5.2). Gold in the milled material can be leached 46 by cyanidation and the appropriate methods used to recover gold from the ensuing pregnant solution. 5.3.3 Complex Gold Ore In complex gold ore, part of the gold is found in quartz and the remaining associated with sulphide minerals and carbonaceous materials. The gold size in the quartz is similar to that in the free milling and thus recovered as such. The size of gold in the sulphide (notably arsenopyrite and pyrite) and the carbonaceous material however are usually sub- microscopic and disseminated in such a way that gold cannot be fully liberated by any mechanical techniques. For this reason, pre-treatment process is very necessary to decompose the sulphide and the carbonaceous material thereby releasing the gold occluded in them (This is shown in Figure 5.3). Once liberated, gold can be leached by cyanidation. ROM ORE ORE BOX WATER SCRUBBER/TROMMEL COARSE MATERIAL FINES GRAVITY CONCENTRATION (10) TAILS ROUGHER CONCENTRATE GRAVITY CONCENTRATION (20) AMALGAMATION* RETORTING* SMELTING GOLD BULLION *Those steps can be skipped 47 Figure 5.1 Simple Flow Sheet for Processing Alluvial Gold Ores ROM ORE COMMINUTION CIRCUIT LEACHING TAILINGS DISSOLVED GOLD ACTIVATED CARBON ADSORPTION ACID WASHING/RINSING CAUSTIC CYANIDE ELUTION CARBON 48 ELUATE REGENERATION ELECTROWINNING CALCINATION OF CATHODE PRODUCT SMELTING GOLD BULLION Figure 5.2 Simple Flow Sheet for Oxide Ore Processing ROM ORE COMMINUTION CIRCUIT FLOTATION FLOTATION TAILINGS FLOTATION CONCENTRATE OXIDATION LEACHING TAILINGS DISSOLVED GOLD ACTIVATED CARBON ADSORPTION ACID WASHING/RINSING CAUSTIC CYANIDE ELUTION CARBON 49 ELUATE REGENERATION ELECTROWINNING CALCINATION OF CATHODE PRODUCT SMELTING GOLD BULLION Figure 5.3 Simple Flow Sheet for Sulphide Ore Processing 5.4 Pre-treatment Options The two major causes of refractoriness in gold ores are ultra-fine gold particles in the matrix of sulfides and the presence of carbonaceous matter. Carbonaceous materials act as a preg-robber during cyanidation. Such ores are pre-treated before gold leaching. Pre- treatment processes targets and decomposes selected associated minerals and opens up their molecular structure so that leaching reagents can make contact with and dissolve the gold. It also eliminates, passivates or drastically reduces the presence of the gangue minerals, which cause refractoriness. These pre-treatment methods are broadly classified into pyrometallurgical and hydrometallurgical oxidation processes. The main pyrometallurgical technique is roasting while hydrometallurgical processes include pressure, chlorine and bacterial oxidation. 5.4.1 Pressure Leaching Pressure leaching involves the dissolution of sulphide ore under high temperature, between 160 and 350 oC, and high pressure in an enclosed vessel, example of which is an autoclave. High temperatures (above 100 oC) in water can be achieved under increased pressure. 50 During the process, sulphides are leached out of the ore into solution and the gold is concentrated in the sub-microscopic state in the residue. This process is relatively expensive and is not practiced in Ghana on large scale as is shown in equations 5.1, 5.2, 5.3 and 5.4 repectively. 4 FeAsS + 11O2 + 2 H 2O → 4 HAsO2 + FeSO4 (5.1) 2 FeS2 + 7O2 + 2 H 2O → 2 FeSO4 + 2 H 2 SO4 (5.2) In the process, iron (II) and arsenic (III) oxidize respectively to the ferric and arsenate states. 4 FeSO4 + 2 H 2 SO4 + 2O2 → 2 Fe2 ( SO4 )3 + 2 H 2O (5.3) 2 HAsO2 + 2O2 + 2 H 2O → 2 H 3 AsO4 (5.4) 5.4.2 Roasting It is the thermal decomposition of sulphide and carbonaceous material at temperatures in excess of 600 C. This results in the oxidation of arsenic, sulphidic sulphur and carbonaceous materials to volatile arsenous oxide, sulphur dioxide and carbon dioxide respectively (which until about 1998 were emitted into the atmosphere), while iron is transformed into iron oxide (hematite), which contains the gold. As 2O3 inhibits plant growth, among others, while sulphur dioxide increases the acidity of soils causing direct damage to vegetation root systems and adversely affecting plant nutrition. It is thus imperative to address these concerns in order to save the environment may undergo chemical reactions as shown in equations 5.5 and 5.6 and 5.7 repectively. 4FeS2 + 11O2 → 2Fe2O3 + 8SO2 (5.5) 2FeAsS + 5O2 → Fe2O3 + As2O3 + 2SO2 (5.6) C + O2 → CO2 (5.7) 5.4.3 Biological Oxidation Consequently the use of biological oxidation (BIOX) system has been considered in recent times. Bacterial oxidation makes use of chemolithotrophic or ‘rock eating’ bacteria, which 51 oxidize sulfides and release the gold particles for leaching by other lixiviants. Bacterial oxidation of gold ores is differentiated from bacterial leaching in that the bacteria used do not dissolve the gold. Several bacteria are known to oxidize sulfides but the ones commonly used in biomining are Thiobacillus thiooxidans, Thiobacillus ferrooxidans and Leptospirillum ferrooxidans. These sulfur and iron oxidizing bacteria are acidophilic mesophiles and work within the pH range of 1.2-1.8 and temperature range of 35 and 45 o C. Bio-oxidation of gold ores may take place in Continuously Stirred Tank Reactors (CSTR) or in heaps and dumps but the use of stirred tank bioreactors is more prominent. Figure 5.4 shows a typical flow sheet for Genmin’s bio-oxidation pre-treatment process for refractory gold concentrates. Cooling water Concentrate Biooxidation reactors Limestone Nutrients Neutralization sludge Stock tank Wash water CCD Air thickeners pH modification, cyanidation/adsorption Fig. 5.4 Typical Flow Sheet for Genmin’s Bio-oxidation Plant Feed to bioreactors is mainly flotation concentrate and the processing time is between 3 and 5 days if high sulfide oxidation levels are expected. The oxidation reactions of pyrite and arsenopyrite are exothermic and consume a large amount of oxygen and bioreactors 52 provide efficient and rapid oxygen uptake as well as effective heat removal. The heat generated may be harmful to the bacteria and CSTRs are equipped with heat exchangers to help keep the temperature within acceptable limits. Dissolved oxygen levels are kept above 2 ppm. During bio-oxidation, supplements such as nitrogen and phosphorus are added in the form of fertilizer grade ammonium sulfate and potassium phosphate or phosphoric acid. Some of the reactions are chemical and can take place in the absence of bacteria while others do not proceed at an appreciable rate without microbial intervention. Pyrite and arsenopyrite may undergo chemical reactions as shown in equations 5.8 and 5.9. 2FeS2 + 7O2 + 2H2O 2FeSO4 + 2H2SO4 5.8 4FeAsS + 11O2 + 6H2O 4H3AsO3 + 4FeSO4 5.9 The oxidation states of Fe (II) and As (III) may be increased to Fe (III) and arsenic (V) by the action of bacteria as given in equations 6.0 to 6.3. These reactions proceed under mild conditions in the absence of bacteria. 4FeS2 + 15O2 + 2H2O 2Fe2(SO4)3 + 2H2SO4 6.0 2FeAsS + 7O2 + H2SO4 + 2H2O 2H3AsO4 + Fe2(SO4)3 6.1 4FeSO4 + O2 + 2H2SO4 2Fe2(SO4)3 + 2H2O 6.2 2H3AsO3 + O2 2H3AsO4 6.3 Advantages of bio-oxidation over alternative pre-treatment processes include the following: Lower capital and operating costs Greater gold recovery Shorter plant construction time Less laborious environmental requirements Arsenic stabilized simple operation, requiring less skilled labour Safer and healthier plants 5.5 Leaching of Gold All the technologies used in treating non-alluvial gold ores thus involve the use of alkaline cyanide solution, in agitation circuits and fixed bed reactors. The low grade gold ore as it 53 is mined consists of minute quantities of the precious metal Au disseminated within large quantities of gangue material. The aim of treatment therefore is to free the gold grains physically by comminution and use chemical processes to extract it. After reducing the ore to a size fine enough to liberate the leachable mineral, Au is transferred chemically from the solid state to the liquid state by cyanidation. There is therefore the need for solid/liquid separation, the filtrate of which is the pregnant liqour from which gold can be won, for example, by Zn precipitation/ cementation or electrowinning. Filtration process however is capital intensive. Furthermore, as a result of depletion of high grade gold ores most of the ores being treated now are low grade (below 2 ppm). This exaggerates the filtration cost setting it around 1.7-2.7 times that of carbon recovery systems. For this reason activated carbon recovery systems have gained very wide acceptance in the gold industry especially over the last 3 decades or so. Au ions found in cyanidation solution are always in the form of complexes. Some of the well-known complexing reagents are cyanide, chloride, iodide, thiosulphate, thiocyanate and thiourea. Cyanide is currently the most widely used lixiviant in the gold industry and the process of leaching gold with cyanide is called cyanidation. The basic leaching reaction of gold in sodium cyanide solution in the presence of air (oxygen) is: 4Au + 8NaCN + O2 + 2H2O → 4Na[Au(CN)2] + 4NaOH 5.7 gold + sodium + oxygen + water → gold in + sodium in ore cyanide solution hydroxide solution From the equation, the reaction is carried out in basic medium between 10.5 and 11 so as to prevent the formation and evolution of hydrogen cyanide gas. More than half the sodium cyanide hydrolyses to HCN below pH of 9.5 in moist air or aqueous environment. Apart from a reduction in the quantity of cyanide available for leaching, the gas evolved is also highly toxic. The pH can be raised using lime, caustic soda or cement. Lime is usually used because it is cheaper and also because it is a viscosity modifier 54 Refractory ores may also contain cyanide-consuming compounds, which are known as cyanicides. These are substances, which cause secondary cyanide- and oxygen-consuming reactions leading to wastage and reduced gold dissolution rate. They include minerals of copper, zinc and partially oxidized arsenides and sulphides. Cyanide should therefore be added in excess of the stoichiometric quantities during leaching. 5.5.1 Gold Dissolution Rate Gold dissolution rate, like other leaching reactions, is affected by the following: Size of Gold Particles: Fine gold particles dissolve faster than coarse gold particles. Oxygen: The dissolved oxygen (D.O.) is a requirement in gold dissolution. Temperature: Gold dissolution rate increases with increasing temp up to about 85 oC. Water: Recycled water from the plant may affect gold dissolution if it contains high concentrations of common salt (sodium chloride) and magnesium sulphate. Coatings and Gold Compounds: Gold occluded by sulphides or coated by Flotation reagents dissolve very slowly. Residence time: The higher the residence time the better the dissolution of gold. Pulp density: High pulp density reduces the quantity of lixiviant and oxygen per unit volume available for leaching. Low densities may result in increased gold dissolution but plant throughput is affected adversely. 5.5.2 Heap Leaching of Gold Ores The two main leaching systems used in gold recovery are heap leaching and agitation leaching. In heap leaching, the ROM ore is crushed to a suitable size and agglomerated if necessary with lime or Portland cement and water. In Ghana, the most suitable binder is Portland cement which serves also as a pH modifier. The particle sizes to which the ore should be crushed and the quantity of cement per tonne of ore could be established by column leach tests. The agglomerated ore may be transported to the impervious pads by stackers or dump trucks. It is then allowed to ‘cure’ for about 72 hrs before irrigation begins. Sodium cyanide solution is sprinkled onto the heaped ore for gold to be leached as it percolates. It may take about seven (7) days for solution to percolate through the heap. Since oxygen is needed for the leaching of gold the dissolved oxygen (D.O.) level should 55 be monitored closely. The solution is enriched with oxygen when it is sprayed into the atmosphere before falling on the heap. The pipes through which the leaching reagent is sprinkled should be placed such that all parts of the heap would receive the solution. There is also a pond system built below the heaps to receive, for example, the pregnant solution from the heap and barren solution after gold recovery. Heap leaching of gold may be influenced by such factors as D.O. levels, nature of ore, percolation, particle size, pH and cyanide concentration. Apart from nature of the ore, all the others are control parameters. Supervisors and operators should therefore make sure that set values are adhered to. 5.5.3 Agitation Leaching of Gold Ores Contrary to heap leaching the run-of mine ore is crushed and ground to liberate the leachable mineral in the case of agitation leaching. It is then pumped into the leached tanks and conditioned to the required pulp density, pH and cyanide concentration. The tanks are equipped with agitators, which serves to stir the pulp exposing the mineral values to the influence of the lixiviant and thus enhancing the leaching rate. 5.6 Gold Recovery from Pregnant Solution After leaching, it may be necessary to separate the solution from the solids before the solution is treated for gold. However, some methods allow gold recovery from slurries. Solid-liquid separation if necessary may be done by thickening followed by filtration and also by washing in counter current decantation thickeners (CCDT). Dissolved gold, can be recovered from pregnant solutions by one or a combination of the following processes: Zinc Cementation Activated Carbon Adsorption Ion exchange/solvent extraction Electrowinning 56 5.6.1 Activated Carbon Adsorption There are two processes by which carbon can be used to win gold from slurry; Carbon In Pulp (CIP) and Carbon In Leach (CIL). In CIP, activated carbon is introduced into a leached pulp to adsorb the Au. It is used for low grade ores and the main purpose is to offset filtration. In CIL, activated carbon is administered at the beginning of cyanidation so that leaching and adsorption take place simultaneously. This process is mainly used in treating ores with a substantial portion of carbonaceous materials which tends to pregrob leached Au during leaching. Because of the association between the carbonaceous material and the ore the carbonaceous materials pass through the comminution stage and are reduced to the size of other minerals making removal selectively after leaching difficult. They are therefore lost to tailings after adsorbing the gold. The natural carbons (carbonaceous material) however need a certain lag of time to start adsorbing due to film coatings. On the contrary activated carbon starts adsorbing immediately it is introduced. As activated carbon is added to the carbonaceous ore both compete for the gold in solution. Now since activated carbon is introduced in greater quantity than the carbonaceous material present in the ore, is more active and has higher adsorption power, it will adsorb more of the gold. Furthermore because of its larger particle size it can be removed selectively after loading. Figure 5.5 Sequence of Carbon and Pulp Transfer 57 All these processes are effective for low grade ores because only the dissolved gold is adsorbed leaving the gangue material as inert. Thus there is no need for filtration. Loaded carbon is removed selectively onto a vibratory screen, washed and then sent to the stripping plant for elution. There is another process called Carbon In Column (CIC) where usually, heap leach liquor is passed through a packed carbon column and gold is loaded onto the carbon. Characteristics of Activated Carbon The wide use of activated carbon for gold recovery processes today is based on the following characteristics of carbon: 1. The ability to handle slimy and carbonaceous ores. 2. The ability to recover Au efficiently irrespective of incoming precious metal concentration. 3. The ability to achieve high Au loading (up to 99.9 %) rapidly from systems containing low levels of Au (below 1 ppm). 4. The ability to release Au rapidly when immersed in a hot concentrated CN solution, producing an eluate suitable for use in zinc precipitation or electrowinning circuits. 5. Its physical strength to resist mechanical breakdown, particularly in loading tanks, where fine carbon loaded with Au may be moved through screens. 6. Its uniform particle size and shape which facilitates screening from the pulp (even in the presence of wood chips). The carbon recovery systems however send more cyanide to the tailings pond than the counter current decantation (CCD) systems. This presents a great threat to wild life and therefore needs an efficient remedy. Factors Affecting Gold Adsorption by Carbon: The amount of gold adsorbed by a given quantity of activated carbon depends on the following (to be discussed in class): Time 58 Gold Concentration Carbon Activity Temperature Agitation Particle Size of Carbon Pulp Density Carbon Fouling It is the reduction in the activity of carbon due to the adsorp