Exercise Physiology Notes PDF
Document Details
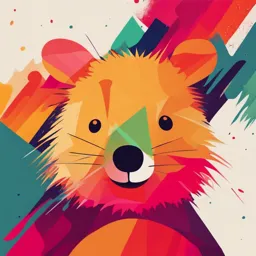
Uploaded by UnequivocalTruth
Tags
Summary
These notes provide an overview of exercise physiology, covering topics like skeletal muscle function, cardiovascular system, respiratory mechanisms, energy metabolism, and thermoregulation. The document outlines various aspects of exercise and its effects on the body, including the influence of factors like age, sex, and climate. It also discusses theoretical elements and practical applications.
Full Transcript
**EXERCISE PHYSIOLOGY\ ** +-----------------------+-----------------------+-----------------------+ | **Unit** | **Topic** | **Page** | +=======================+=======================+=======================+ | **I** | **Skeletal Muscles |...
**EXERCISE PHYSIOLOGY\ ** +-----------------------+-----------------------+-----------------------+ | **Unit** | **Topic** | **Page** | +=======================+=======================+=======================+ | **I** | **Skeletal Muscles | | | | and Exercise** | | +-----------------------+-----------------------+-----------------------+ | | Macro & Micro | | | | Structure of the | | | | Skeletal Muscle | | +-----------------------+-----------------------+-----------------------+ | | Chemical Composition | | +-----------------------+-----------------------+-----------------------+ | | Sliding Filament | | | | theory of Muscular | | | | Contraction | | | | (Mechanisms of | | | | Muscular Contraction) | | +-----------------------+-----------------------+-----------------------+ | | Types of Muscle fibre | | +-----------------------+-----------------------+-----------------------+ | | Muscle Tone among | | | | Athletic Groups | | +-----------------------+-----------------------+-----------------------+ | | Chemistry of Muscular | | | | Contraction | | +-----------------------+-----------------------+-----------------------+ | | Heat Production in | | | | the Muscle | | +-----------------------+-----------------------+-----------------------+ | | Effect of exercises | | | | and training on the | | | | muscular system | | +-----------------------+-----------------------+-----------------------+ | | Muscular fatigue | | +-----------------------+-----------------------+-----------------------+ | | Influence of age and | | | | sex on muscle | | | | function | | +-----------------------+-----------------------+-----------------------+ | **II** | **Cardiovascular | | | | System and Exercise** | | +-----------------------+-----------------------+-----------------------+ | | Heart Valves and | | | | Direction of the | | | | Blood Flow | | +-----------------------+-----------------------+-----------------------+ | | Conduction System of | | | | the Heart | | +-----------------------+-----------------------+-----------------------+ | | Blood Supply to the | | | | Heart | | +-----------------------+-----------------------+-----------------------+ | | Functional Capacity | | | | at Rest and During | | | | Physical Activity | | +-----------------------+-----------------------+-----------------------+ | | Cardiac Cycle Stroke | | | | Volume | | +-----------------------+-----------------------+-----------------------+ | | Cardiac Output, Heart | | | | Rate & Blood Pressure | | +-----------------------+-----------------------+-----------------------+ | | Factors Affecting | | | | Heart Rate | | +-----------------------+-----------------------+-----------------------+ | | Cardiac Hypertrophy | | +-----------------------+-----------------------+-----------------------+ | | Effect of exercises | | | | and training on the | | | | Cardio vascular | | | | system | | +-----------------------+-----------------------+-----------------------+ | **III** | **Respiratory System | | | | and Exercise** | | +-----------------------+-----------------------+-----------------------+ | | Structure of the | | | | pulmonary system, | | | | Mechanics of | | | | Breathing | | +-----------------------+-----------------------+-----------------------+ | | Respiratory Muscles, | | | | Minute Ventilation, | | | | ventilation at Rest | | | | and During Exercise | | +-----------------------+-----------------------+-----------------------+ | | Diffusion of Gases, | | | | Exchange of Gases in | | | | the Lungs | | +-----------------------+-----------------------+-----------------------+ | | Mechanism of Gaseous | | | | Exchange in the Lungs | | | | and in the Tissues | | +-----------------------+-----------------------+-----------------------+ | | Control of | | | | Ventilation, | | | | Ventilation and the | | | | Anaerobic Threshold. | | +-----------------------+-----------------------+-----------------------+ | | Oxygen Debt - Lung | | | | Volumes and | | | | Capacities | | +-----------------------+-----------------------+-----------------------+ | | Effect of exercises | | | | and training on the | | | | respiratory system | | +-----------------------+-----------------------+-----------------------+ | **IV** | **Energy Metabolism | | | | and Transfer** | | +-----------------------+-----------------------+-----------------------+ | | Metabolism; ATP - PC | | | | or Phosphagen System | | +-----------------------+-----------------------+-----------------------+ | | Anaerobic Metabolism | | +-----------------------+-----------------------+-----------------------+ | | Aerobic Metabolism | | +-----------------------+-----------------------+-----------------------+ | | Aerobic and Anaerobic | | | | Systems during Rest | | | | and Exercise | | +-----------------------+-----------------------+-----------------------+ | | Short Duration High | | | | Intensity Exercises | | +-----------------------+-----------------------+-----------------------+ | | High Intensity | | | | Exercise Lasting | | | | Several Minutes, Long | | | | Duration Exercises. | | +-----------------------+-----------------------+-----------------------+ | **V** | High Intensity | | | | Exercise Lasting | | | | Several Minutes, Long | | | | Duration Exercises. | | +-----------------------+-----------------------+-----------------------+ | | Thermal balance | | | | during Cold, Heat and | | | | Humidity, | | | | Thermoregulation | | +-----------------------+-----------------------+-----------------------+ | | Sports performance in | | | | hot climate, Cold | | | | Climate, high | | | | altitude | | +-----------------------+-----------------------+-----------------------+ | | Overview of Doping | | | | and its hazards | | +-----------------------+-----------------------+-----------------------+ | | Stimulants and sports | | | | performance Influence | | | | of: | | | | | | | | Amphetamine, Anabolic | | | | steroids, | | | | Androstenedione, Beta | | | | Blocker, Choline, | | | | Creatine - Human | | | | growth hormone on | | | | sports performance. | | | | Narcotic, Stimulants: | | | | Amphetamines, | | | | Caffeine, Ephedrine, | | | | Sympathomimetic | | | | amines - Stimulants | | | | and sports | | | | performance. | | +-----------------------+-----------------------+-----------------------+ **Unit 1** **Skeletal Muscles and Exercise** Macro & Micro Structure of the Skeletal Muscle, Chemical Composition - Sliding Filament theory of Muscular Contraction (Mechanisms of Muscular Contraction) - Types of Muscle fibre - Muscle Tone among Athletic Groups - Chemistry of Muscular Contraction - Heat Production in the Muscle - Effect of exercises and training on the muscular system -- Muscular fatigue -- Influence of age and sex on muscle function **Unit 2** **Cardiovascular System and Exercise** Heart Valves and Direction of the Blood Flow - Conduction System of the Heart - Blood Supply to the Heart - Functional Capacity at Rest and During Physical Activity- Cardiac Cycle Stroke Volume - Cardiac Output - Heart Rate- Blood Pressure - Factors Affecting Heart Rate - Cardiac Hypertrophy - Effect of exercises and training on the Cardio vascular system **Unit 3** **Respiratory System and Exercise** Structure of the pulmonary system, Mechanics of Breathing - Respiratory Muscles - Minute Ventilation, ventilation at Rest and During Exercise - Diffusion of Gases - Exchange of Gases in the Lungs - Mechanism of Gaseous Exchange in the Lungs and in the Tissues - Control of Ventilation - Ventilation and the Anaerobic Threshold. Oxygen Debt - Lung Volumes and Capacities - Effect of exercises and training on the respiratory system **Unit 4** **Energy Metabolism and Transfer** Metabolism - ATP - PC or Phosphagen System - Anaerobic Metabolism - Aerobic Metabolism - Aerobic and Anaerobic Systems during Rest and Exercise - Short Duration High Intensity Exercises - High Intensity Exercise Lasting Several Minutes, Long Duration Exercises. **Unit 5** **Environmental conditions and sports performance, Doping and ergogenic aids** Thermal balance during Cold, Heat and Humidity -- Thermoregulation - Sports performance in hot climate, Cold Climate, high altitude - Overview of Doping and its hazards - Stimulants and sports performance Influence of: Amphetamine, Anabolic steroids, Androstenedione, Beta Blocker, Choline, Creatine - Human growth hormone on sports performance. Narcotic, Stimulants: Amphetamines, Caffeine, Ephedrine, Sympathomimetic amines - Stimulants and sports performance. **Unit 1** **SKELETAL MUSCLE AND EXERCISE** **Introduction** **Exercise physiology is a scientific discipline that focuses on understanding the physiological and biochemical processes that occur in the body during physical activity and exercise. It explores how the body responds, adapts, and improves through exercise, and it plays a crucial role in enhancing athletic performance, promoting health, and preventing and managing various medical conditions.** **The Importance of Exercise Physiology** **Understanding Human Performance: Exercise physiology provides insights into how different systems of the body---such as the cardiovascular, respiratory, muscular, and nervous systems---work together during physical activity. This knowledge is essential for athletes, coaches, and trainers to optimize performance and design effective training programs.** **Health and Wellness: Regular physical activity is essential for maintaining good health and preventing chronic diseases such as obesity, diabetes, cardiovascular diseases, and certain cancers. Exercise physiology helps in understanding the mechanisms behind these benefits, enabling healthcare professionals to prescribe exercise as medicine.** **Rehabilitation and Therapy: For individuals recovering from injuries or surgeries, or those managing chronic conditions, exercise physiology offers evidence-based approaches to rehabilitation. Tailored exercise programs can enhance recovery, improve function, and reduce the risk of further complications.** **Key Concepts in Exercise Physiology** **Energy Systems: The body relies on three primary energy systems to fuel physical activity: the phosphagen system (for short, intense activities), glycolysis (for moderate-duration activities), and oxidative phosphorylation (for long-duration activities). Understanding how these systems work and how they are trained is fundamental to improving performance and endurance.** **Muscle Physiology: Muscles are the primary movers in the body, and their function is influenced by factors such as fiber type composition, neural activation, and metabolic processes. Exercise physiology examines how muscles generate force, adapt to training, and fatigue during exercise.** **Cardiovascular and Respiratory Responses: During exercise, the heart, blood vessels, and lungs work together to deliver oxygen and nutrients to tissues and remove waste products. Exercise physiology studies these responses to understand how they can be enhanced through training and how they contribute to overall performance and health.** **Hormonal Regulation: Hormones play a crucial role in regulating various physiological processes during exercise, including energy metabolism, muscle growth, and recovery. Understanding hormonal responses to exercise helps in designing effective training and nutrition strategies.** **Environmental Factors: Exercise physiology also considers how environmental factors such as temperature, altitude, and humidity affect performance and safety. This knowledge is vital for preparing athletes for competition in different conditions and for ensuring safe exercise practices for all individuals.** **Applications of Exercise Physiology** **Athletic Training and Performance: Exercise physiologists work with athletes to design training programs that enhance strength, endurance, speed, and overall performance. They use scientific principles to optimize training loads, recovery, and nutrition.** **Public Health and Fitness: Exercise physiology informs public health initiatives and fitness programs aimed at increasing physical activity levels in the population. This includes developing guidelines for different age groups and fitness levels.** **Clinical Exercise Physiology: In a clinical setting, exercise physiologists collaborate with healthcare providers to prescribe and monitor exercise for patients with chronic diseases, helping to manage symptoms and improve quality of life.** **Research and Innovation: Ongoing research in exercise physiology leads to new discoveries about the human body and its capabilities. This research drives innovation in sports science, rehabilitation, and health promotion.** **Exercise physiology is a dynamic and interdisciplinary field that bridges the gap between science and practical application. By understanding how the body responds to exercise, we can unlock the potential for improved performance, better health, and enhanced quality of life for individuals of all ages and abilities. Whether you are an athlete, a healthcare professional, or someone interested in fitness, the principles of exercise physiology provide a foundation for making informed decisions about physical activity and well-being.** **Types of Muscles in the Human Body** **The human body contains three distinct types of muscles, each with specialized functions and characteristics: skeletal muscle, cardiac muscle, and smooth muscle. These muscle types are essential for various bodily functions, including movement, circulation, and digestion.** **Skeletal Muscle** **These are the muscles that you can control consciously. They are attached to bones by tendons and allow you to move your body. Skeletal muscles are striated, meaning they have a banded appearance.** **Structure and Appearance:** - **Striated: Skeletal muscle fibres have a striated appearance due to the organized arrangement of myofilaments (actin and myosin) within the sarcomeres.** - **Multinucleated: Each skeletal muscle fibre contains multiple nuclei, which are located at the periphery of the cell.** **Control:** - **Voluntary: Skeletal muscles are under conscious control, meaning they are activated by signals from the nervous system that are intentionally generated.** **Function:** - **Movement: Skeletal muscles are attached to bones by tendons and facilitate movement by contracting and pulling on the bones.** - **Posture: These muscles maintain posture and stabilize joints, preventing unwanted movements.** - **Heat Production: During contractions, skeletal muscles produce heat, which helps regulate body temperature.** **Adaptability:** - **Hypertrophy: With resistance training, skeletal muscles increase in size and strength.** - **Endurance: With aerobic training, skeletal muscles improve their endurance capabilities.** **Cardiac Muscle** **This is the muscle that makes up the heart. It is also striated, but it is involuntary, meaning you don\'t have to think about it to make it work. Cardiac muscle contracts rhythmically to pump blood throughout the body.** **Structure and Appearance:** - **Striated: Like skeletal muscle, cardiac muscle has a striated appearance due ~t=o~ the organization of sarcomeres.** - **Branched: Cardiac muscle fibers are branched, allowing them to form a network.** - **Single Nucleus: Most cardiac muscle cells have a single, centrally located nucleus.** **Control:** - **Involuntary: Cardiac muscle is not under conscious control. Its activity is regulated by the autonomic nervous system and intrinsic pacemaker cells within the heart.** **Function:** - **Pumping Blood: Cardiac muscle forms the myocardium (heart muscle) and is responsible for the rhythmic contractions that pump blood throughout the body.** - **Endurance: Cardiac muscle is highly resistant to fatigue due to a high density of mitochondria and a rich blood supply.** **Unique Features:** - **Intercalated Discs: Cardiac muscle cells are connected by intercalated discs, which contain gap junctions and desmosomes. These structures facilitate synchronized contractions and provide mechanical stability.** **Smooth Muscle** **This type of muscle is found in the walls of organs such as the stomach, intestines, and blood vessels. Smooth muscle is not striated and is involuntary. It helps to move food through the digestive system and constrict or dilate blood vessels.** **Structure and Appearance:** - **Non-Striated: Smooth muscle lacks the striations seen in skeletal and cardiac muscle due to the irregular arrangement of actin and myosin filaments.** - **Spindle-Shaped: Smooth muscle cells are spindle-shaped, with a single, centrally located nucleus.** **Control:** - **Involuntary: Smooth muscle activity is not under conscious control and is regulated by the autonomic nervous system, hormones, and local factors.** **Function:** - **Movement of Substances: Smooth muscle is found in the walls of hollow organs (e.g., intestines, blood vessels, and bladder) and is responsible for moving substances through these organs by rhythmic contractions (peristalsis).** - **Regulation of Blood Flow: Smooth muscle in blood vessel walls helps regulate blood pressure and flow by contracting and relaxing.** **Adaptability:** - **Plasticity: Smooth muscle can undergo hypertrophy and hyperplasia (increase in cell number) in response to chronic changes in workload.** **\ ** **Comparison of Muscle Types** **Feature** **Skeletal Muscle** **Cardiac Muscle** **Smooth Muscle** -------------- ------------------------------------ --------------------------------------- ---------------------------------------------------------- Appearance Striated, multinucleated fibres Striated, branched, single nucleus Non-striated, spindle-shaped, single nucleus Control Voluntary Involuntary Involuntary Location Attached to bones Heart Walls of hollow organs (e.g., intestines, blood vessels) Function Movement, posture, heat production Pumping blood Movement of substances, regulation of blood flow Adaptability Hypertrophy, endurance High endurance, intrinsic rhythmicity Plasticity, hypertrophy, hyperplasia **The three types of muscle---skeletal, cardiac, and smooth---each play unique and vital roles in the body. Understanding their structure, control mechanisms, and functions is essential for comprehending how the body operates and adapts to various demands. Whether facilitating movement, pumping blood, or regulating organ function, these muscle types are integral to maintaining health and homeostasis.** **SKELETAL MUSCLE AND EXERCISE** **Skeletal muscles are one of the most fascinating and essential components of the human body. These muscles, also known as voluntary muscles, are responsible for enabling movement, supporting our posture, and maintaining body temperature. Understanding the structure and function of skeletal muscles is crucial for anyone studying anatomy, physiology, or physical education. In this teaching content, we will explore the characteristics, organization, and role of skeletal muscles in greater detail to provide a comprehensive understanding of this fundamental aspect of the musculoskeletal system.** **[MACRO & MICRO ANATOMY OF SKELETAL MUSCLES]** - **Skeletal muscles are sheathed by a tough layer of connective tissue called the epimysium. It also protects muscles from friction against other muscles and bones. Within the epimysium are multiple bundles called fascicles, each of which contains 10 to 100 or more muscle fibers collectively sheathed by a perimysium. A single muscle e.g. biceps contains approx. 1000 muscle fibres. A muscle fibre is enclosed by the plasma membrane called sarcolemma and the cytoplasm inside it is called sarcoplasm.** - **These fibres run the whole length of the muscle** - **Muscle fibres are joined together at the tendons** **The thread like muscle fibres are the individual muscle cells (myocytes), and each cell is encased within its own endomysium of collagen fibers. Thus, the overall muscle consists of fibers (cells) that are bundled into fascicles, which are themselves grouped together to form muscles. At each level of bundling, a collagenous membrane surrounds the bundle, and these membranes support muscle function both by resisting passive stretching of the tissue and by distributing forces applied to the muscle.** **Important points --** **1) Structural and functional unit of muscle -- Muscle cell** **2) Structural and functional unit of muscle contraction or contractile unit of muscles--Sarcomere** **3) The basic functional unit of myofibril is the sarcomere.** **4) Smallest functional unit of muscle -- sarcomere.** Portion of a myofibril, showing sarcomere structure: **S** = Sarcomere, unit of muscle function **A** = A-band, region of myosin **I** = I-band, region of actin **H** = H-zone, region of just myosin **Z** = Z-line, sarcomere boundary **M** = M-line, sarcomere center **This same bundles-within-bundles structure is replicated within the muscle cells. Within the cells of the muscle are myofibrils, which themselves are bundles of protein filaments. The term \"myofibril\" should not be confused with \"myofiber\", which is a simply another name for a muscle cell. Myofibrils are complex strands of several kinds of protein filaments organized together into repeating units called Sarcomeres (distance between two Z lines). The striated appearance of both skeletal and cardiac muscle results from the regular pattern of sarcomeres within their cells.** **The filaments in a sarcomere are composed of actin and myosin. That means each myofibril in turn contains many fine protein filaments called myofilaments called actin and myosin.** **Physiology of Muscles** **The three types of muscle (skeletal, cardiac and smooth) have significant differences. However, all three use the movement of actin against myosin to create contraction. In skeletal muscle, contraction is stimulated by electrical impulses transmitted by the nerves, the motoneurons (motor nerves) in particular. Cardiac and smooth muscle contractions are stimulated by internal pacemaker cells which regularly contract, and propagate contractions to other muscle cells they are in contact with. All skeletal muscle and many smooth muscle contractions are facilitated by the neurotransmitter acetylcholine.** **[INTRODUCTION OF SLIDING FILAMENT THEEORY]** **The sliding filament theory was first introduced by Andrew Huxley, A. Hodgkin, Albert Szent Gyorgyi in 1953-54.** **The first muscle protein discovered was Myosin by German scientist willy Kuhne in 1864. In 1939 a Russian husband and wife discovered that Myosin had an enzymatic property ATPase that can breakdown ATP to release energy.** **Albert Szent Gyorgyi --: A Hungarian physiologist demonstrated in 1942 that ATP was the source of energy for muscle contraction.** **What is sliding filament theory?** **The sliding filament theory is the explanation for how muscles contract to produce force. The actin and myosin filaments within the sarcomeres of muscle fibres bind to create cross-bridges and slide past one another, creating a contraction. The sliding filament theory explains how these cross-bridges are formed and the subsequent contraction of muscle.** **In order for a skeletal muscle contraction to occur;** **1. There must be a neural stimulus** **2. There must be calcium in the muscle cells** **3. ATP must be available for energy** **SARCOMERES** are the smallest functional unit of a muscle fiber. They are composed of precisely organized proteins referred to as thick filaments (Myosin) and thin filaments (Actin). - **Acetylcholine** is a chemical messenger, a neurotransmitter, released by nerve cells. It controls the **contraction** of all **skeletal** or voluntary **muscles**. It also affects the **contraction** of smooth and cardiac muscle. - Calcium is a chemical element with symbol Ca and atomic number 20. - An **ion** is an atom of a chemical element that has an unequal number of electrons compared to protons. Remember that electrons are negative charges, and protons are positive charges. **[INTERIOR STRUCTURE OF NEUROMUSCULAR JUNCTION]**  **STEP - I -: RESTING MUSCLE** - Sarcolemma is an outer layer of muscle fiber which is called T-tubules play a very significant role in this process. - The outer layer of sarcomere is electro positive and from inner side electro negatively known as polarized. - This potential is known as resting potential. - Active sites of actin are blocked. **STEP -- II -: Muscle Activation (action potential in sarcomere)** - In second step nerve impulse has started and make neuromuscular junction. - Axon going to release to neurotransmitter. - As soon as neurotransmitter comes and binds to the sarcolemma, it gets depolarized. And Ca+ released from sarcoplasmic reticulum. - Depolarized means negative charge would be the outer side and inner would side positive. - Membrane permeability has changed. **STEP -- III -: Muscle contraction (troponin binds with ca+ )** - Cytoplasm has Ca+ released. - Troponin has a very high affinity towards Ca+. - Troponin will bind with Ca+ and the active site of Actin gets free. - Now the Myosin head can bind with active site of Actin (Tropomyosin). **STEP -- IV -: Recharging** - ATP is re-synthesized (re-manufactured) allowing actin and myosin to maintain their strong binding state. - When the ATP is then broken down the Myosin head can again attach to an Actin binding site further along the Actin filament and repeat the 'power stroke'. - This repeated pulling of the Actin over the myosin is often known as the ratchet mechanism. **STEP -- V -: Relaxation** Relaxation occurs when stimulation of the nerve stops. Calcium is then pumped back into the sarcoplasmic reticulum breaking the link between actin and myosin. Actin and myosin return to their unbound state causing the muscle to relax. Alternatively relaxation will also occur when ATP is no longer available. **SLIDING FILAMENT THEORY: OVERVIEW** Here is what happens in detail. The process of a muscle contracting can be divided into 5 sections: 1. A nerve **impulse arrives** at the neuromuscular junction, which causes a release of a chemical called **Acetylcholine**. The presence of Acetylcholine causes the **depolarisation** of the motor endplate which travels throughout the muscle by the transverse tubules, causing **Calcium** (Ca+) to be released from the sarcoplasmic reticulum. 2. In the presence of high concentrations of Ca+, the **Ca+ binds to Troponin**, changing its shape and so moving **Tropomyosin** from the **active site of the Actin**. The Myosin filaments can now attach to the Actin, forming a **cross-bridge.** 3. The breakdown of **ATP releases energy** which enables the Myosin to **pull the Actin** filaments inwards and so **shortening** the muscle. This occurs along the entire length of every myofibril in the muscle cell. 4. The Myosin detaches from the Actin and the cross-bridge is broken when an ATP molecule binds to the Myosin head. When the ATP is then broken down the Myosin head can again attach to an Actin binding site further along the Actin filament and repeat the **'power stroke'**. This repeated pulling of the Actin over the myosin is often known as the **ratchet mechanism**. 5. This process of muscular contraction can last for as long as there are adequate **ATP and Ca+ stores**. Once the impulse stops the Ca+ is pumped back to the Sarcoplasmic Reticulum and the Actin returns to its resting position causing the muscle to **lengthen and relax**. **Chemical Composition** Approximately **75% of skeletal muscle is water, 20% is protein and the remaining 3% is made of inorganic salts and other substances including.high energy phosphates, urea, lactic acid, etc.** The minerals calcium, magnesium and phosphorous, various enzymes and pigments, ions of sodium, potassium, and chloride, and amino acids, fats, and carbohydrates are also present in the muscle cell. **The most abundant muscular proteins are myosin, actin, and tropomyosin**. About 700 mg of the conjugated protein myoglobin is incorporated into 100 grams of muscle tissue. **MUSCLE FIBRES TYPES** **Mainly there are two types of muscle fibres --** **1) Slow twitch -- Type I --Red muscle, large quantity of reddish molecule called myoglobin, where oxygen is stored. It is also called oxidative muscle. In this ATP is used up rather slowly.** **2) Fast twitch-- Type II- Plentyful glycogen is present, sometimes called as glycolytic muscle. It has less or no myoglobin, therefore stores less oxygen. ATP used up quickly but cant regenerate quicly coz of lack of oxygen. It becomes quickly fatigues with the build up of lactic acid. where type II is further divided into two types -- type IIaand Type IIx, where Type IIx was considered as the fastest muscle fibre in human history but now Type IIa.** **Note -- Fast fibre contracts more rapidly because of ATPase which promote breakdown of ATP ( high VO2max). Slow fibres have low ATPase.** ---------------------------- -- -- low specific tension. Strained , black in colour ---------------------------- -- -- **MUSCLE TONE --** **Tonic Contraction, or muscle tone, is characterized of the muscles of normal individuals, when they are awake. It is particularly important for important maintain posture.** **Muscle with less tone than normal is described as Flaccid Muscle and those with more than normal tone are called spastic.** **Abnormal Muscle Contraction -- Cramps, convulsions, fibrillation.** **Muscle Diseases -- Strain, myalgia, sprain, myositis, Fibro myositis, Cramp, Cramp, Contusions, Poliomyelitis, muscular dystrophy, Myasthenia graves, Hernia.** **Muscle Fiber Types** The human skeletal muscle is made up of a mixture of slow (type I) and Fast (type II) twitch groups of muscle fibers and are classified according to their contractile and metabolic characteristics. The percentage of slow to fast twitch fibers present in specific muscles are considered to be constant (predetermined genetically), ranging from 13 to 60 percent for slow, and 40 to 85 percent for fast twitch muscle fibers. The percentage of muscle fiber composition plays a very crucial role in determining the performance capacities of world class athletes. For example, world class sprinters have a very high percentage of fast twitch muscle fibers (about 74 percent), while world class distance runners have a very high level of slow twitch muscle fibers (about 75 percent) in their leg muscles. Fast-twitch muscle fibers or type II muscle fibers have a faster rate of electrochemical transmission of action potentials and a higher activity level of myosin ATP enzyme. The type II fibers have a faster rate of release of calcium from the sarcoplasmic reticulum, which helps them to generate energy rapidly for quick, forceful contractions. The fast twitch fiber's intrinsic speed of contraction and tension development is two to three times faster than the slow-twitch fibers. The fast twitch fibers rely largely on a well developed, short term glycolytic system for energy transfer. They have been labeled as fast glycolytic (FG) fibers to signify their fast glycogenolytic capabilities. Fast twitch fibers are generally activated in short-term sprint activities as well as in other forceful muscular contractions that depend almost entirely on anaerobic metabolism for energy. The metabolic and contractile capacities of these fibers are suited for short distances sprint activities and also in the change of pace sports, such as Basketball, Soccer, Tennis, Field hockey, etc. These sports at times require rapid energy that only the anaerobic metabolic pathways supply. The fast twitch muscle fibers are predominantly found in biceps brachii, deltoid, gastrocnemius, and latissimus dorsi. Slow twitch fibers generate energy for ATP resynthesis predominantly by means of the relatively long term system of aerobic energy transfer. They are distinguished by a low activity level of myosin ATPase, a slow speed of contraction, and a glycolytic capacity less well developed compared to that of the fast twitch muscle fibers. The slow twitch fibers contain a number of mitochondria's which are relatively large in size. It is this concentration of mitochondria combined with high levels of myoglobin that give the slow- twitch fibers their characteristic red pigmentation. Accompanying this enhanced metabolic activity is a high concentration of mitochondrial enzymes required to sustain the aerobic metabolism for a long duration. Thus slow twitch fibers resists early muscular fatigue and are well suited for prolonged aerobic exercise. The slow twitch muscle fibers are predominantly found in soleus, semitendinous, vastus lateralis, rectus -abdominis and rectus femoris muscles. **Muscle Tone** Muscle tone is a continual, partial contraction. At anyone movement a small number of the total fibers in the muscle contraction, producing a firmness of the muscle rather than a recoganisable contraction and movement. Different groups of muscle scattered throughout the muscle contract in relays. The muscle tone is characteristic of the muscles of normal individuals when they are awake and is particularly important in maintaining posture. **Staircase Phenomenon or Trepper** Increasingly stronger twitch contractions occur in the muscle in response to maximal stimuli of the muscle, which are repeated at a rate of once or twice a second. In other words, the muscle contracts more forcefully after it has contracted a few times compared to the contraction force generated by it initially. The staircase phenomenon occurs due to the rise in temperature of the active muscles and also due to the accumulation of the metabolic products. Hence, after a few stimuli, the muscle responds to successive stimuli with maximal contraction. **Chemistry of Muscular Contraction** The actin and myosin are the two muscular protein filaments that are responsible for the muscular contraction. They form the contractile elements of the muscle. The muscular contraction takes place when the actin filaments slide over the myosin filaments. The process of liberation of muscular energy ie. Adenosine Triphosphate (ATP), takes place as follows: 1. When ATP is broken down by the enzyme ATPase, adenosine diphosphate and a phosphate radical is formed. The phosphate bond is broken to release energy for muscular contraction. **A T P \-\-\--\> A D P + P + Energy** Above reactions occur in the muscles and without oxygen (Anaerobic Glycolysis). About one fifth of the lactic acid produced from glycogen is oxidized to CO2 and H2O. The remaining lactic acid is converted to glycogen and stored in liver, muscles and other tissues. **Heat Production in the Muscle** Contraction of muscle causes production of heat to increase beyond the resting level. This increase in heat in the muscles takes place as follows; **Activation Heat** The activation heat is produced when the calcium dissociates from its bound state in the sarcoplasmic reticulum. This takes place before the actual tension development of the muscle. **Shortening Heat** During isotonic contraction heat is generated when the contraction of the muscle takes place. This is due to formation of the bond between the myosin and actin filaments and heat is continued to be produced during the sliding of the actin along the myosin filament as the cross bridges attach with the actin filaments. An additional maintenance heat is also produced due to the break down of ATP. **Relaxation Heat** In Isotonic contraction, relaxation heat takes place during relaxation, when weight is lowered by the muscles. At this point, since there is weight as the contractile filaments are relaxing there is a need for the supply of ATP and its break down. Hence heat is produced. If the muscle relaxes without weight, no heat is produced. **Delayed Heat** The muscle liberates delayed recovery heat once the contraction and relaxation of the muscles are completed. Under aerobic conditions, when oxygen is available for muscular contraction, the oxidative delayed heat is liberated. **MUSCLE SENSE** Muscle sense (proprioception) is the brain's ability to know where our muscles are and what they are doing, without our having to consciously look at them. Within muscles are receptors called stretch receptors (proprioceptors or muscle spindles). The general function of all sensory receptors is to detect changes. The function of stretch receptors is to detect changes in the length of a muscle as it is stretched. The sensory impulses generated by these receptors are interpreted by the brain as a mental "picture" of where the muscle is. Learning a skill such as typing or playing the guitar involves very precise movements of the fingers, and beginners will often watch their fingers to be sure they are moving properly. With practice, however, the movements simply "feel" right, which means that the brain has formed a very good mental picture of the task. Muscle sense again becomes unconscious, and the experienced typist or guitarist need not watch every movement. All sensation is a function of brain activity, and muscle sense is no exception. The impulses for muscle sense are integrated in the parietal lobes of the cerebrum (conscious muscle sense) and in the cerebellum (unconscious muscle sense) to be used to promote coordination **CARDIOVASCULAR SYSTEM AND EXERCISE** The cardiovascular system consists of the heart, which is a muscular pumping device, and a closed system of vessels called as arteries, veins and capillaries. The blood contained in the circulatory system is pumped by the heart around a closed circuit of vessels as it passes repeatedly through different systems of the human body. **Structure of the heart --** - Heart is situated in the [thoracic cavity] between the lungs towards left side. - The weight about **280 - 340gm in males and in female it is 230-280 gm, and new born baby weighs 20gms.** - **Heart** is a shape of **Length** of **12 cm, width 9cm, depth 6cm**. - **2/3** of the Heart mass is found on the left side of the body and the other **1/3** on the right. - The heart is located in the thoracic cavity between the lungs. This area is called the **mediastinum.**  Heart is situated in the thoracic cavity between the lungs towards left side. Heart has four chambers -- [right auricle, right ventricle, left auricle, left ventricle]. Upper auricles are separated by a partition known as intra-auricular septum, similarly ventricles are divided by intra-ventricular septum. Cardiac muscles are called **Myocardium** which are striated and involuntary. Heart has three layers called **Endocardium (inner layer), myocardium, and pericardium**. Myocardium contains only type I fiber. Cardiac muscle contraction occurs by **"calcium release".** **.**  **Coronary artery** supplies the blood to the heart. Right coronary supplies to the right side divided into two branches -- the marginal artery and posterior inter-ventricular artery. The left coronary artery divided into two -- circumflex artery and anterior descending artery. **Valves** -- There are four valves in the heart. They are formed by the endocardium. They allow the blood to pass only in one direction. They are -- Right atrio-ventricular valve (tricuspid valve), left atrioventricular valve (Bicuspid valve or mitral valve), Pulmonary semilunar valve, and aortic semilunar valve. **Heart Murmur** -- A heart murmur is a condition in which abnormal heart sounds are detected with the stethoscope. **Blood Circulation** -- 1) Systemic circulation, 2) Pulmonary circulation  **CARDIAC CONDUCTION SYSTEM** Cardiac muscle has its own ability to generate its own electrical signal called **spontaneous rhythmicity**, which allows it to contract without any external stimulation; it imitates its own pulse with neural control. This signal creates an electrical current that can be seen on a graph called an Electrocardiogram (EKG or ECG). Cardiac muscle has its own ability to generate its own electrical signal called **spontaneous rhythmicity**, which allows it to contract without any external stimulation; it imitates its own pulse with neural control. This signal creates an electrical current that can be seen on a graph called an Electrocardiogram (EKG or ECG). There are three main components to an ECG: the **P wave**, which represents the depolarization of the atria; the **QRS complex**, which represents the depolarization of the ventricles; and the **T wave**, which represents the repolarization of the ventricles. During each heartbeat, a healthy heart has an orderly progression of depolarization that starts with **pacemaker cells** in **the sinoatrial node**, spreads throughout the **atrium**, and passes through the **atrioventricular node** down into the **bundle of His** and into the **Purkinje fibers**, spreading down and to the left throughout the **ventricles**. This orderly pattern of depolarization gives rise to the characteristic ECG tracing. **There are four main components --** 1. **S.A Node** -- The impulse for normal heart contractions is initiated in the sinoatrial node(S.A Node), group of specialized cardiac muscle fiber located in the upper posterior wall the right atrium.This tissue generate the impulse, typically at a frequency of aboust 60-80 bpm. Also known as heart's pacemaker and rhythm it establishes is called Sinus Rhythm. S.A node causing the atrial muscles to contract.The electrical impulse reaches AV node. 2. **A.V Node** -- Located in the right atrial wall near the center of the heart. It conducts electrical signals from atrium to ventricles. The impulse is delayed by 0.13 sec as it passes through node and enters AV bundle. This delay allows the atria to fully contract before the ventricles do, maximizing ventricular filling. 3. **A.V Bundle** -- it travels through ventricular septum and then sends right and left bundle branches into both ventricles. Then it branched into the terminal branches called Purkinje fibers. 4. **Purkinje fibers** -- They transmit impulses through the ventricles 6 times faster than rest cardiac conduction system.  Arrhythmias are irregular heartbeats; their effects range from harmless to life-threatening. Nearly everyone experiences heart palpitations (becoming aware of an irregular beat) from time to time. These are usually not serious and may be the result of too much caffeine, nicotine, or alcohol. Much more serious is ventricular fibrillation, a very rapid and uncoordinated ventricular beat that is totally ineffective for pumping blood **CARDIAC CYCLE --** it includes all the mechanical and electrical events that occur during 1heart beat. It includes all events occurring between two consecutive heart beats. The cardiac cycle is the sequence of events in one heartbeat. In its simplest form, the cardiac cycle is the simultaneous contraction of the two atria, followed a fraction of a second later by the simultaneous contraction of the two ventricles FORMULA:  [**The** **cardiac** **cycle** **are** **four** **main** **events:**] 1. 2. 3. 4. **Diastole --** Relaxation phase (ventricles relax, chambers filled with blood). **Systole --** contraction phase (Ventricles contract and expels blood into aorta and pulmonary artery. **Atrial Systole** - The Atria contract and push the blood into the ventricle. To facilitate this filling, the AV valves stay open and the semilunar valve stay closed to keep arterial blood from re- entering the Heart. - The contraction phase last for 0.1 sec. - At this time the pressure in the Atria is higher than the pressure in the ventricle. **Atrial Diastole** - It last for about 0.7 sec. - Both Atria relaxes and during which filling of blood into the Atria takes place from large veins (Superior vena cava, Inferior vena cava and pulmonary veins) **Ventricular Systole** - It last for about 0.3 sec. - Both Ventricle contracts to push blood into the Aorta and Pulmonary trunk. - The pressure of the ventricles forces the semilunar valve to open and the AV valves to close. - Pressure in the Right Ventricle is higher than the pulmonary Artery. - Pressure in the Left Ventricle is higher than the Aorta. **Ventricular Diastole** - It last for about 0.5 sec - Both Ventricles relaxes and it begins before Atrial systole during which filling of blood into Ventricles takes place from Atria. **Joint Diastole** - During the relaxation phases all four chambers of the Heart in diastole as blood pours into the Heart from the Veins. - The ventricles fill to about 75% capacity during this phase and will be completely filled only after the Atria enter systole. - It last for about 0.4 sec - This is the point where both Atria and Ventricle relaxes. As the heart rate increases, this time shortens. In systole when the ventricles contract, pressure inside the ventricles rises and makes atrio-ventricular valves closed (opening of pulmonary and aortic valve) making **Sound 1** and closing of pulmonary and aortic valve (atrio-ventricular valves) makes **Sound 2.** **HEART RATE- Rate at which your heart pumps out blood from left ventricle. It is of three types --** 1) Maximum H.R. 2\) Resting H.R 3\) Target H.R **Calculate your maximum heart rate.** The most common way to calculate your maximum heart rate is to subtract your age from 220. If you're 25 years old, your HR~max~ = 220 -25 = 195 beats per minute (bpm). There is some research that suggests this formula oversimplifies the calculation. You can also estimate your max heart rate with the formula HR~max~ = 205.8 -- (0.685 x age). **Cardio-vascular Drift --(**CV~drift~) is the phenomenon where some [cardiovascular] responses begin a time dependent change, or \"drift\" after around 10 minutes of exercise in a warm or neutral environment without an increase in workload. It is characterized by decreases in mean [arterial pressure] and [stroke] [volume] and a parallel increase in [heart rate]. It has been shown that a reduction in stroke volume due to dehydration is almost always due to the increase in internal temperature. +-----------------------------------------------------------------------+ | **Stroke volume** - Ventricular stroke volume (SV) is often thought | | of as the amount of blood (mL) ejected per beat by the left ventricle | | into the aorta (or from the right ventricle into the pulmonary | | artery). *Stroke* *volume* is the difference between end-diastolic | | and end-systolic volumes; The EDV is the filled volume of the | | ventricle prior to contraction and the ESV is the residual volume of | | blood remaining in the ventricle after ejection. In a typical heart, | | the EDV is about 120 mL of blood and the ESV about 50 mL of blood. | | The difference in these two volumes, 70 mL, represents the SV. | | Therefore, any factor that alters either the EDV or the ESV will | | change SV. **SV = EDV -- ESV** | +=======================================================================+ | **CARDIAC OUTPUT (CO** | +-----------------------------------------------------------------------+ | | +-----------------------------------------------------------------------+ | **Cardiac output is the amount of blood pumped by a ventricle in 1 | | minute. A certain level of cardiac output is needed at all times to | | transport oxygen to tissues and to remove waste products. During | | exercise, cardiac output must increase to meet the body's need for | | more oxygen. People e return to exercise after first considering | | resting cardiac output.** | | | | **To calculate cardiac output, we must know the pulse rate and how | | much blood is pumped per beat. Stroke volume is the term for the | | amount of blood pumped by a ventricle per beat; an average resting | | stroke volume is 60 to 80 mL per beat. A simple formula then enables | | us to determine cardiac output:** | | | | **Naturally, cardiac output varies with the size of the person, but | | the average resting cardiac output is 5 to 6 liters per minute. | | Notice that this amount is just about the same as a person's average | | volume of blood. At rest, the heart pumps all of the blood in the | | body within about a minute. Changes are possible, depending on | | circumstances and extent of physical activity.** | | | | **If we now reconsider the athlete, you will be able to see precisely | | why the athlete has a low resting pulse. In our formula, we will use | | an average resting cardiac output (5 liters) and an athlete's pulse | | rate (50):** | | | | **Cardiac output = stroke volume x pulse** | | | | **5000 mL = stroke volume x 50 bpm** | | | | **5000/50 = stroke volume** | | | | **100 mL = stroke volume** | | | | **Notice that the athlete's resting stroke volume is significantly | | higher than the average. The athlete's more efficient heart pumps | | more blood with each beat and so can maintain a normal resting | | cardiac output with fewer beats.** | | | | **Now let us see how the heart responds to exercise. Heart rate | | (pulse) increases during exercise, and so does stroke volume. The | | increase in stroke volume is the result of Starling's law of the | | heart, which states that the more the cardiac muscle fibers are | | stretched, the more forcefully they contract. During exercise, more | | blood returns to the heart; this is called venous return. Increased | | venous return stretches the myocardium of the ventricles, which | | contract more forcefully and pump more blood, thereby increasing | | stroke volume. Therefore, during exercise, our formula might be the | | following:** | | | | **Cardiac output = stroke volume x pulse** | | | | **Cardiac output = 100 mL x 100 bpm** | | | | **Cardiac output = 10,000 mL (10 liters)** | | | | **This exercise cardiac output is twice the resting cardiac output we | | first calculated, which should not be considered unusual. The cardiac | | output of a healthy young person may increase up to four times the | | resting level during strenuous exercise. This difference is the | | cardiac reserve, the extra volume the heart can pump when necessary. | | If resting cardiac output is 5 liters and exercise cardiac output is | | 20 liters, the cardiac reserve is 15 liters. The marathon runner's | | cardiac output may increase six times or more compared to the resting | | level, and cardiac reserve is even greater than for the average young | | person; this is the result of the marathoner's extremely efficient | | heart. Because of Starling's law, it is almost impossible to overwork | | a healthy heart. No matter how much the volume of venous return | | increases, the ventricles simply pump more forcefully and increase | | the stroke volume and cardiac output.** | | | | **Also related to cardiac output, and another measure of the health | | of the heart, is the ejection fraction. This is the percent of the | | blood in a ventricle that is pumped during systole. A ventricle does | | not empty completely when it contracts, but should pump out 60% to | | 70% of the blood within it. A lower percentage would indicate that | | the ventricle is weakening.** | | | | **Ejection Fraction** -- It is the blood pumped out of the left | | ventricles in relation to the amount of blood that was in the | | ventricles before contraction. It is generally in %. | | | | **Ejection fraction = stroke volume ÷ end-diastolic volume** | | | | In a healthy 70-kilogram (150 lb) man, the stroke volume is | | approximately 70 mL, and the left ventricular end-diastolic volume | | (EDV) is approximately 120 mL, giving an estimated ejection fraction | | of 70⁄120, or 0.58 (58%). Healthy individuals typically have ejection | | fractions between 50% and 65%. | | | | **SEVERE CONDITIONS -** | | | | - **Bradycardia** is a slower than normal heart rate. The hearts of | | adults at rest usually beat between 60 and 100 times a minute. If | | you have **bradycardia**, your heart beats fewer than 60 times a | | minute. | | | | - **Tachycardia** is the medical term for a heart rate over 100 | | beats per minute. There are many heart rhythm disorders | | (arrhythmias) that can cause **tachycardia**. | +-----------------------------------------------------------------------+ | | +-----------------------------------------------------------------------+ | | +-----------------------------------------------------------------------+ **Integrated control of blood pressure -** B.P is normally maintained by reflexes from the autonomic nervous system. **Baroreceptors -** Baroreceptors are mechanoreceptors located in the carotid sinus and in the aortic arch. Their function is to sense pressure changes by responding to change in the tension of the arterial wall. **Mechanoreceptors**- A mechanoreceptor, also called mechanoceptor, is a sensory cell that responds to mechanical pressure or distortion. **Chemoreceptors** - A sensory cell or organ responsive to chemical stimuli. Chemoreceptors are sensors that detect changes in CO2, O2, and pH, and have been classified, based upon anatomical location, as either central or peripheral. **Proprioreceptors** -- A sensory receptor which receives stimuli from within the body, especially one that responds to position and movement. Proprioception, also referred to as kinaesthesia, is the sense of self-movement and body position. It is sometimes described as the \"sixth sense\". **Noci-receptors** - A nociceptor (\"pain receptor\") is a sensory neuron that responds to damaging or potentially damaging stimuli by sending "possible threat" signals to the spinal cord and the brain. \[Note -Sympathetic nervous system controls the blood flow and distribution of blood to the heart and from the heart.\] Extrinsic control of the heart is controlled by PNS, SNS, and Endocrine system. **NERVOUS REGULATION OF THE HEART**  EFFECT OF EXERCISE ON DIFFERENT SYSTEMS OF THE BODY --------------------------------------------------- Acute and chronic exercise programmes develops physiological adaptations in different systems of the body, due to the stress placed on the system. Acute exercise refers to short duration exercise, such as a cycle ergometer or a treadmill maximal exercise test. Chronic refers to extended or long term exercise, such a physical training programme of four to six months duration. ----------------------------------------------------------------------------------------------------------------------------------------------------------------------------------------------------------------------------------------------------------------------------------------------------------------------------------------------------------------------------------------- Effect of Exercise on the Circulatory System -------------------------------------------- Acute and chronic exercise causes an augmented supply of oxygenated blood to the working tissues and an increased return of deoxygenated blood to the heart and the lungs. Due to chronic exercise, the following adaptive changes in the circulatory system take place: Stroke Volume ------------- Cardiac Output -------------- Blood Pressure -------------- Coronary Circulation -------------------- Cardiac Muscles --------------- Arterial-Venous Difference (a-v O~2~ dift) ------------------------------------------ Resting Heart Rate ------------------ Heart Rate during Exercise -------------------------- Blood Flow ---------- Availability of Nutrients ------------------------- **UNIT III** **RESPRATORY SYSTEM** **STRUCTURE AND FUNCTIONS OF THE RESPIRATORY SYSTEM** The human respiratory system is a series of organs responsible for taking in oxygen and expelling carbon dioxide. The primary organs of the respiratory system are the lungs, which carry out this exchange of gases as we breathe. The lungs work with the circulatory system to pump oxygen-rich blood to all cells in the body. The tract is divided into an upper and a lower respiratory tract. The upper tract includes the nose, nasal cavities, sinuses, pharynx and the part of the larynx above the vocal folds. The lower tract includes the lower part of the larynx, the trachea, bronchi, bronchioles and the alveoli. Gas exchange in the lungs occurs in millions of small air sacs called alveoli in mammals. Respiration is made up of two phases called inspiration and expiration: You inhale (breathe in) oxygen during inspiration. You exhale (breathe out) carbon dioxide during expiration. Respiration includes the following processes **NOTE -- At rest, a person need 10 L of oxygen while during exercise person need 120- 150 L of oxygen which is better to taken through mouth.** **MECHANICS OF BREATHING**  **INSPIRATION:** Inspiration is the active part of the breathing process, which is initiated by the respiratory control centre in medulla oblongata (Brain stem). Activation of medulla causes a contraction of the diaphragm and intercostal muscles leading to an expansion of thoracic cavity and a decrease in the pleural space pressure. The diaphragm is a dome-shaped structure that separates the thoracic and abdominal cavities and is the most important muscle of inspiration. When it contracts, it moves downward and because it is attached to the lower ribs it also rotates the ribs toward the horizontal plane, and thereby further expands the chest cavity. In normal quite breathing the diaphragm moves downward about 1 cm but on forced inspiration/expiration total movement could be up to 10 cm. When it is paralysed it moves to the opposite direction (upwards) with inspiration, paradoxical movement. The external intercostal muscles connect adjacent ribs. When they contract the ribs are pulled upward and forward causing further increase in the volume of the thoracic cavity. As a result fresh air flows along the branching airways into the alveoli until the alveolar pressure equals to the pressure at the airway opening. **EXPIRATION:** Expiration is a passive event due to elastic recoil of the lungs. However, when a great deal of air has to be removed quickly, as in exercise, or when the airways narrow excessively during expiration, as in asthma, the internal intercostal muscles and the anterior abdominal muscles contract and accelerate expiration by raising pleural pressure. **COUPLING OF THE LUNGS AND THE CHEST WALL:** The lungs are not directly attached to the chest wall but they change their volume and shape according to the changes in shape and volume of the thoracic cavity. Pleura covering the surfaces of the lungs (visceral) or the thoracic cavity (parietal) together with a thin (20 µm) layer of liquid between them create a liquid coupling. **PRESSURE-VOLUME RELATIONSHIPS:** In the pulmonary physiology absolute pressure means atmospheric pressure (760 mm Hg at sea levels). The pressures and the pressure differences of the respiratory system are expressed as relative pressures to the atmospheric pressure. When it is said that alveolar pressure is zero, it means that alveolar pressure = atmospheric pressure. **REGULATION AND CONTROL OF BREATHING:** In order to maintain normal levels of partial oxygen and carbon dioxide pressure both the depth and rate of breathing are precisely regulated. Basic elements of the respiratory control system are \(1) strategically placed sensors (2) central controller (3) respiratory muscles. **CENTRAL CONTROLLER:** Breathing is mainly controlled at the level of brainstem. The normal automatic and periodic nature of breathing is triggered and controlled by the respiratory centres located in the pons and medulla. These centres are not located in a special nucleus or a group of nuclei but they are rather poor defined collection of neurones. **1. Medullary respiratory centre:** - Dorsal medullary respiratory neurones - Ventral medullary respiratory neurones **2.Apneustic Centre** **3.Pneumotaxic centre** **RESPIRATORY MUSCLES:** Diaphragm, intercostal muscles and the other accessory respiratory muscles work in co-ordination for normal breathing under central controller. There is evidence suggesting that in premature new-born babies this co-ordination is not mature enough and this could be responsible for the sudden infant death syndrome. **SENSORS:** - **Mechanoreceptors** - **Chemoreceptors** **VENTILATION (How does the inspired air get into the alveoli?)** **AIRWAYS AND AIRFLOW:** Inhaled air passes through the conducting airways and eventually reaches the respiratory epithelium of the lungs. The conducting airways consist of a series of branching tubes which become narrower, shorter and more numerous as they penetrate deeper into the lung. The trachea divides into right and left main bronchi, which in turn divide into lobar, then segmental bronchi. This process continues down to the terminal bronchioles, which are the smallest airways without alveoli. Since the conducting airways have no alveoli they do not take part in gas exchange but constitute the anatomical dead space. Its volume is about 150 ml but it varies because airways are not rigid; during inspiration, respiratory tubes are lengthened and dilated, especially in deep breathing. Since the airways serve as a barrier as well, harmful foreign material including most micro-organisms can not easily enter the lower respiratory passages. The very first barrier starts at the vestibules of the nose, which contain hairs, and healthy, sticky mucus intercepting air-borne particles. Caught particles are then ejected by ciliated epithelium, which covers the entire upper respiratory tract. Various factors can interfere with ciliary activity: for example nicotine and tar in tobacco smoking. Coughing occurs in response to chemical or mechanical irritation of nerve endings in the upper respiratory tract. The larynx and the bifurcation of the trachea are the most sensitive regions and any particles of foreign matter lodged in these regions are removed when a cough sends a rapid blast of air sweeping out the respiratory tree.  The alveolated region of the lung includes respiratory bronchioles (divided from terminal bronchioles and have only occasional alveoli on their walls) and alveolar ducts (completely lined with alveoli). This zone is called respiratory zone and the gas exchange occurs here. The distance from the terminal bronchiole to the distal alveous is only a few mm, but the respiratory zone makes up most of the lung, its volume being about 2.5 to 3 L. Blood is brought to the other side of the blood-gas barrier from the right heart by pulmonary arteries, which also form a series of branching tubes leading to the pulmonary capillaries and back to the pulmonary veins. The capillaries lie in the walls of the alveoli and form a dense network that the blood continuously runs in the alveolar wall. At resting not all the capillaries are open but when the pressure rises (e.g. exercise) recruitment of the close capillaries occurs. The diameter of a capillary segment is about 10 µm, just large enough for a red blood cell. The pulmonary artery receives the whole output of the right heart, but resistance of pulmonary circuit is very low. This enables the high blood flow to the circuit. **LUNG VOLUMES AND PULMONARY FUNCTION TESTS:** Pulmonary function can be examined by the **spirometry technique**. Spirometers are the traditional tools of the respiratory physiologists. The subject breathes into a closed system in which air is trapped (bell). As the subject breathes air movement into or out of the mouthpiece causes the bell to rise (inspiration) or fall (expiration). Corresponding movements of an attached pen register the change in volume on a rotating drum recorder. From such a recording we could measure **Tidal volume (TV):** Volume of air inhaled or exhaled with each breath during normal breathing (0.5 L). Inspiratory reserve volume (IRV): Maximal volume of air inhaled at the end of a normal inspiration (3 L) Expiratory reserve volume (ERV): Maximal volume of air exhaled at the end of a tidal volume (1.2 L) **Inspiratory capacity (IC):** Maximal volume of air inhaled after a normal expiration (3.6 L) (TV+IRV) **Functional Residual Capacity (FRC):** The volume of gas that remains in the lung at the end of a passive expiration. (2-2.5 L or 40 % of the maximal lung volume) (ERV+RV). **Residual Volume (RV):** The volume of gas remains in the lung after maximal expiration. (1-1.2 L) Note: FRC and RV can not be measured with an ordinary spirometer (For details see below). **Total Lung Capacity (TLC):** The maximal lung volume that can be achieved voluntarily. (5-6 L) (IRV+ERV+TV+RV) **Vital capacity (VC):** The volume of air moved between TLC and RV. (4-5 L) (IRV+ERV+TV). Multiplying the tidal volume at rest by the number of breaths per minute gives the total minute volume (6 L/min). During exercise the tidal volume and the number of breaths per minute increase to produce a total minute volume as high as 100 to 200 L/min. **Measurements of Functional Residual Capacity (FRC) and Residual Volume (RV):** There are two techniques to study these volumes: Helium Spirometry: In this technique a subject is connected to a spirometer filled with helium which is virtually insoluble in the blood. After some breathes the amount of helium in the lung and the spirometer reach equilibrium. **TOTAL VENTILATION:** The total volume of the gas leaving the lung per unit time. If TV is 500 ml and there are approximately 15 breaths/min the total volume of the gas leaving the lung, total ventilation will be 500 x 15 = 7500 ml/min. It can be measured by having the subject breath through a valve that separates the inspired air from expired air and collecting the expired air. **ALVEOLAR VENTILATION:** The volume of the gas reaching the respiratory zone of the airways. However, not all of the total ventilation volume reaches the alveoli. 150 ml of the TV (500 ml) is left behind in the airways, which does not contain alveoli, therefore does not contribute the diffusion (Anatomic death space). Thus, the volume of gas entering the respiratory zone, alveolar ventilation, is (500-150) x 15 = 5250 ml/min. The measurement of alveolar ventilation is more difficult. One way is to measure the volume of anatomic dead space and calculate the dead space ventilation. This then subtracted from the total ventilation. **Alveolar ventilation = Total ventilation -- Anatomic death space ventilation** **Anatomic dead space ventilation = Anatomic dead space volume x respiration frequency** **ANATOMICAL DEAD SPACE:** Volume of the conducting airways. It is approximately 150 ml but its volume increases with large inspiration and depends on the size and the posture of the subject. Measurement of dead space: Fowler's method: The subject breaths pure oxygen through a valve box and a rapid nitrogen analyser samples and measures the nitrogen concentration in the expired air. After a single inspiration of pure oxygen (100 %) nitrogen concentration in the expired air is increased as the gas in the dead space is washed by pure oxygen. Nitrogen concentration quickly reaches a plateau level (alveolar plateau). The dead space is found by plotting nitrogen concentration against the expired volume. The expired volume up to the vertical line drawn such that area A = area B represents the anatomical dead space volume. **PULMONARY FUNCTION TESTS :** Pulmonary function tests are very useful tests to diagnose several lung diseases. The simplest but one of the most informative tests of lung function is a forced expiration. **TESTS OF VENTILATORY CAPACITY** **Forced Expiratory Volume (FEV):** It is the volume of gas exhaled in one second by a forced expiration following a full inspiration (FEV1). The total volume of the gas exhaled after a full inspiration represents the vital capacity. However, this value could be slightly smaller than the vital capacity measured with a slow (normal speed) expiration. Therefore, this value is called forced vital capacity (FVC). The normal ratio of the FEV1 is 80 % of FVC. **Forced Expiratory Flow (FEF25-75):** This measurement represents the expiratory flow rate over the middle half of the FVC (between 25 -- 75 %). It is obtained by identifying the 25 % and 75 % volume points of FVC, measuring the time between these points and calculating the flow rate. Interpretation of tests of forced expiration: On the basis of the knowledge obtained from these functional tests, lung diseases can be classified as restrictive or obstructive. In restrictive lung diseases (such as pulmonary fibrosis), the vital capacity is reduced to below normal levels. However, the rate at which the vital capacity is forcefully exhaled is normal. In obstructive lung disease (such as asthma, emphysema, bronchitis) the vital capacity is normal because lung tissue is not damage and its compliance is unchanged. In asthma the small airways (bronchioles) constrict, bronchoconstriction increases the resistance to airflow. Although the vital capacity is normal, the increased airway resistance makes expiration more difficult and takes longer time. Obstructive disorders are therefore diagnosed by tests that measure the rate of forced expiration, such as the FEV1 and FEF25-75. A significant decrease in these values suggests an obstructive lung disease. **DIFFUSION.** **How do gasses get across the blood-gas barrier?** **BLOOD-GAS EXCHANGE**: Oxygen and carbon dioxide move between air and blood by simple diffusion: from an area of high to low partial pressure, as simple as water runs downhill. It is a passive process which means requires no energy. Fick's law of diffusion determines the amount of gas moves across the tissue is proportional to the area of the tissue but inversely proportional to its thickness. Because the blood-gas barrier in the lung is extremely thin and has a very large area (50-100 m2 ), it is well suited to its function **How does the lung achieve such a large surface area of blood-gas barrier inside the limited thoracic cavity?** This is achieved by wrapping the pulmonary capillaries around an enormous number of small air sacs, alveoli, and each about 1/3 mm in diameter. There are about 300 million alveoli in the human lung, creating 85 m2 surface area but having a volume of only 4 L. **Calculations of Oxygen and Carbon Dioxide Partial Pressures:** Dalton's Law: Total pressure of a gas mixture (in our case air) is equal to the sum of the pressures that each gas in the mixture would have independently (Partial Pressure of each gas). **P~dry~ atmosphere = PN2 + PO2 + PCO2 = 760 mmHg** **Since oxygen constitutes 21 % of the atmosphere, PO2 = 159 mm Hg.** **nitrogen 78 PN2 = 593 mmHg** Inspired air also contains moisture and its amount may vary with temperature etc. However when the inspired air arrived the alveoli it is normally saturated with water vapour. Because the temperature in the lungs does not change significantly water vapour of the alveolar air could be considered constant (47 mm Hg) **P~wet~ atmosphere = PN2 + PO2 + PCO2 + PH2O = 760 mmHg** PO2 = 0.21 (760-47) = 150 mm Hg (oxygen partial pressure of the inspired air when it arrives alveoli, before the gas exchange). **Why are the measurements of PO2 and PCO2 important?** The measurement of PO2 of arterial blood is particularly important because it provides a good index of lung function. The actual amount of dissolved O2 is a linear function of the PO2: The higher PO2 indicates that more O2 is dissolved. Blood PO2 measurements are not affected by the O2 in red cells (bound with Hb, see below for details). A normal PO2 in the inspired air together with low arterial PO2 means that the gas exchange in the lungs is impaired. In summary, the measurement of PO2 is important for (1) treating patients with pulmonary diseases (2) performing safe surgery (when anaesthesia is used) (3) the care of premature babies with respiratory distress syndrome.  **RESPIRATORY SYSTEM UNDER STRESS** **EXERCISE:** During exercise the rate and the depth of breathing are increased. This increase in ventilation (hyperpnea) matches the simultaneous increase in oxygen consumption and carbon dioxide production that the arterial blood carbon dioxide and oxygen partial pressures and pH do not change dramatically (Please note that hyperpnea is different from hyperventilation. In hyperventilation PCO2 is decreased). The mechanism underlying the exercise-induced changes in ventilation is not clear. **1. Neurogenic mechanisms:** **2. Chemical mechanisms:** Because partial pressures of carbon dioxide and oxygen do not change during exercise it is difficult to explain possible chemical factors. However, the focal changes in these parameters near chemoreceptor area may contribute to the exercise-induced changes in ventilation. **Anaerobic threshold:** The maximum rate of oxygen consumption than can be attained before blood lactic acid levels rise as a result of anaerobic respiration. Lactic acid concentration is increased due to anaerobic limitations in the muscle cells during heavy exercise. \[The cardiovascular system maintains an adequate amount of oxygen to the tissue that mitochondria can carry out oxidative phosphorylation. Oxidative phosphorylation is an oxygen consuming process by which energy derived from substrate oxidation is stored in ATP as a chemical energy. When the oxygen consumption is increased (e.g. heavy exercise) or at low PO2 (e.g. cellular hypoxia) anaerobic glycolysis becomes a major mechanism for cellular ATP formation and glucose is reduced to lactate.\] **ACCLIMATIZATION TO HIGH ALTITUDE:** In the high altitude the human body compensates the low partial oxygen pressure by changing ventilation or affinity of Haemoglobin (Hb) to oxygen or total Hb concentration. **Hypoxic ventilatory response:** Hyperventilation induced by the decreased partial oxygen pressure. This lowers the arterial partial carbon dioxide pressure and causes respiratory alkalosis. The rise in blood pH in turn set the ventilation to a more stable but still slightly higher levels. Hyperventilation increases the tidal volume and reduces the proportion of the anatomical death space in the inspired air. This also improves the oxygenation of the blood. However, in spite of all these adaptation mechanisms, the partial oxygen pressure in the arterial blood can not be increased more than the partial oxygen pressure in the inspired air. As a result partial pressure of oxygen in the arterial blood decreases with increasing altitude. At sea levels arterial blood loses 22% of its oxygen load in tissues: The oxygen saturation of the arterial and venous blood is 97% and 75%, respectively. At high altitude the low oxygen content of red blood cells stimulates 2-DPG production and decreases the affinity of Hb to oxygen, which in turn facilitates the oxygen transport to the tissues. However, at very high altitudes increase in blood pH causes a shift to the left in the oxygen saturation curve and increases the affinity of Hb to oxygen. This second step is indeed beneficial at very high altitude by increasing the oxygenation of the blood in the lungs. Due to low oxygen partial pressure in the arterial blood at high altitude the tissue hypoxia occurs and in response the kidneys secrete erythropoietin hormone. Erythropoietin stimulates the production of red blood cells resulting in polycythemia, which can cause oedema, ventricular hypertrophy and heart failure. **INTERNAL AND EXTERNAL RESPIRATION --** Gas exchange between the air in the lungs and blood, sometimes called **external respiration.** Gas exchange between the blood and the tissues, sometimes called **internal inspiration.** **NOTE - A pulmonologist is a specialist in the diagnosis and treatment of diseases of the lungs.** **Structure of Respiratory System:** ***The respiratory system consists of*** The nose The nasal cavity The pharynx The larynx The trachea Bronchi The lungs Bronchioles Alveoli Diaphragm **NOSE** The interior structures of the external nose have three functions: filtration, smelling, warming the inhaled air. **Pharynx** The pharynx functions as a passageway for air and food both. It is extending 13cm (5in.) from the internal nostrils to the larynx. Air continues through the pharynx. It is extended from Nasopharynx, oropharynx, Laryngopharynx. **Larynx (voice box)** It is also known as the voice box is a short section of the airway that connects the laryngopharynx and the trachea. It keep the food and water away from the air passage and has many other additional purposes. The Larynx contains special structures known as vocal folds, which allow the body to produce the sounds of speech and singing. The superior opening of the larynx is guarded by flap of tissue known as epiglottis. **Trachea** The trachea or windpipe is a tubular passageway for air that is about 12 cm (5 in.) long and 2.5 cm (1 in.) in diameter. The Trachea or windpipe is a 5-inch long tube made of C shaped **hyaline cartilage rings**. **The Lungs** The cavity between Lungs is called Parietal. The functional unit of Lungs is Alveoli. Covering of Lungs is called Pleural (Visceral and Parietal). The space between the right and left Lungs is known as Mediastinum.  **ALVEOLI** Alveoli are tiny, balloon-shaped air sacs. Their job is to move oxygen and carbon dioxide (CO2) molecules into and out of your bloodstream. They are the smallest structures in the respiratory system. They sit at the ends of the branches of your respiratory tree. There are 150 million alveoli in each lung. The walls of the alveoli are very thin. This lets oxygen and CO2 pass easily between the alveoli and capillaries, which are very small blood vessels. At this point, the oxygen molecules move through a single layer of lung cells in the alveolus, then through a single cell layer in a capillary to enter the bloodstream. **GASEOUS EXCHANGE BETWEEN ALVEOLI & CAPPILARIES -** **Partial Pressure Gradients** Partial pressure gradients (differences in partial pressure) allow the **loading of oxygen into the bloodstream** and the **unloading of carbon dioxide out** of the bloodstream. These two processes occur at the same time. The concentration of **Oxygen in the atmosphere is about 21%** and **total pressure is 760 mm Hg**. Atmospheric PO2 = 21% \* 760 = **159.6 mmHg** **Oxygen** has a partial **pressure gradient** of about **60 mmHg** (**100 mmHg in alveolar air and 40 mmHg in deoxygenated blood**) and diffuses rapidly from the alveolar air into the capillary. The process is similar in **carbon dioxide**. The partial pressure gradient for **carbon dioxide** is much smaller compared to oxygen, being only **5 mmHg** (**45 mmHg in deoxygenated blood and 40 mmHg in alveolar air**). **HOW TO MEASURE RESPIRATORY CAPACITY --** ***Maximum Aerobic Capacity (Vo2 Max)*** VO2 Max also called maximal oxygen consumption, maximal oxygen uptake is defined as the volume of oxygen consumed at maximum effort. VO2 reflects cardio- respiratory fitness and endurance. VO2 max is measured in millilitres of oxygen used in one minute per kilogram of body weight (mL/kg/min). **Oxygen deficit** A temporary shortage of oxygen in the body at starting phase of exercise. **Oxygen dept** It is the lacuna of oxygen in human body after termination of exercise. **EPOC (Excess Post-exercise Oxygen Consumption)** It can be described as the amount of oxygen consumed during recovery in excess of that which would have ordinarily been consumed at rest. Some factors that contribute to EPOC include the replenishment of CP and ATP, the conversion of lactate to pyruvate, and the resynthesis of glycogen. **Second wind** Second wind is usually described as a sudden transition from an ill-defined feeling of distress or fatigue during an early portion of prolonged exercise to a more comfortable, less stressful feeling later in the exercise  **STATIC LUNG VOLUME** **Tidal volume**: the volume of air inspired or expired with each normal breath (500ml) **Inspiratory Reserve Volume**: Extra volume of air that can inspired over and beyond the normal tidal volume. (3,000 ml-3300ml). **Expiratory reserve volume**: the amount of air that can still be expired by forced expiration beyond the normal tidal expiration(1,100 ml- 1200ml) **Residual volume**: volume of air remaining in the lung after forced expiration. (1,200 ml) **LUNG CAPACITIES** **Inspiratory capacity**: Amount of the air a person can breathe beginning at the normal expiratory level and distending his lungs to the maximum amount (TV+ IRV) (3500 ml). **Functional residual capacity**: amount of air remaining in the lung at the end of normal expiration. (ERV+RV) (2,300 ml). **Vital capacity**: the amount of air expelled during forced expiration (IRV+ERV + TV) (4,600 ml). **Total lung capacity**: Maximum vol. to which lungs can be expanded with the greatest possible inspiratory efforts. (VC+RV) **IMPORTANT TERMINOLOGIES** **EUPNEA** -- It is a term used to determine normal quiet breathing. **HYPERPNEA** -- It means increased breathing rate. **DYSPNEA** - It refers to difficulty in breathing. **APNEA** -- It refers to temporary cessation of breathing at the end of a normal expiration. **Effect of Exercise on the Respiratory System** The respiratory system does not limit exercise performance, since it is capable of supplying adequate oxygen and in the prompt removal of carbon di oxide. Regular training causes physiological adaptations in the respiratory system. They are as follows: i. **Efficiency of Gaseous Exchange** Due to exercise the efficiency of the complete respiratory system is improved. The respiratory pathways and the area for exchange of gases are constantly used and increased blood flow is present in these areas. As a result there in an increase in the ability of the lungs to enhance the exchange of gases that takes place between the lungs and the blood. This improves the efficiency of the respiratory system. ii. **Ventilation-Perfusion Ratio** At rest, about 4.2 liters of air ventilate the alveoli each minute and the quantity of blood that flows through the pulmonary capillaries is about 5.0 liters. The ratio of alveolar ventilation to pulmonary blood flow is known as ventilation-perfusion ratio and is 0.8 (4.2 + 5.0 = 0.8). This means that for each liter of blood that perfuses the lungs, 0.8 liters of ventilation is available to the alveoli of the lungs. During maximal exercise there is a disproportionate increase in alveolar ventilation and the ventilation-perfusion ratio may increase beyond 5.0 to provide adequate supply of air to the blood returning to the lungs. iii. **Availability of O~2~ and Elimination of CO~2~** Maximal exercise increases the quantity of air that is moved in and out of the lungs by many folds. There is an increase in the tidal volume and in the frequency of breathing per minute. In well conditioned athletes, the minute volume may increase to 150 liters in response to maximal exercise. The amount of O~2~ that will reach the alveoli is greater and its diffusion across the alveoli membrane and to the arterial blood is increased. During acute exercise above 90% of the lungs are ventilated compared to only 60% ventilation at rest. Hence the amount of O~2~ available for diffusion in the blood through the alveoli is more and hence the greater purification of blood takes place. The CO~2~ from the venous blood diffuses into the lungs and are exhaled quickly so as to maintain normal acid-base balance. iv. **Increased Area for Exchange of Gases** Important factors that are necessary for exchange of gases are (a) Increased surface area of the alveoli \(b) Increased surface tension around the alveoli and (c) a reduced thickness of the membrane of the alveoli. Regular exercise causes continuous movement of air in and out of the lungs in large volumes and as a result the elasticity and surface tension of alveoli are greatly increased. This helps in faster exchange of O~2~ and CO~2~ across the membranes of the alveoli. v. **Respiratory Muscles** The amount of O~2~ consumed by the respiratory muscles at is about one to two percent of the total oxygen consumption of the body. During heavy exercise, with the increased active movement of the respiratory muscles, the oxygen consumption of the ventilatory muscles increases to about eight to ten percent of the total oxygen consumption of the body. This increase in oxygen cost of the respiratory muscles is sufficient to meet the demands of strenuous exercises. vi. **Body Temperature and pH** One of the most important functions of respiratory system is to maintain the body Homeostasis (normal body's internal environment). By increasing or decreasing the respiratory rate, the respiratory system is able to maintain the normal body temperature and pH, so that the quantity of CO~2~ present in the blood is maintained at normal levels. Athletes compared to non athletes have greater ability to fluctuate above or below normal homeostasis, which is brought back to normal within a short span of time by increasing the respiratory frequency (hyperpnoea) and expelling the excess CO~2~' vii. **Ability to function at low O~2~ levels** Since athletes are used to performing at O~2~ debt, their capacity to continue exercise is far greater than non-athletes. The athletes can also perform exercise anaerobically for a longer time than non- athletes. In hill stations, where the availability of O~2~ is low in the atmosphere, the athletes quickly develop physiological coping mechanisms, such as increase in concentration of hemoglobin and in glycolytic oxidative enzyme. **UNIT IV** **METABOLISM** All energy originates from the sun as light energy. Chemical reactions in plants (photosynthesis) convert light into stored chemical energy. In turn, humans obtain energy either by eating plants or by eating animals that feed on plants. Nutrients from ingested foods are provided in the form of carbohydrates, fats, and proteins. These three basic fuels, or energy substrates, can ultimately be broken down to release the stored energy. Each cell contains chemical pathways that convert these substrates to energy that can then be used by that cell and other cells of the body, a process called bioenergetics. All of the chemical reactions in the body are collectively called metabolism. Because all energy eventually degrades to heat, the amount of energy released in a biological reaction can be calculated from the amount of heat produced. Energy in biological systems is measured in calories. By definition, 1 calorie (cal) equals the amount of heat energy needed to raise 1 g of water 1 °C, from 14.5 °C to 15.5 °C. In humans, energy is expressed in kilocalories (kcal), where 1 kcal is the equivalent of 1,000 cal. Sometimes the term Calorie (with a capital C) is used synonymously with kilocalorie, but kilocalorie is more technically and scientifically correct. Thus, when one reads that someone eats or expends 3,000 Cal per day, it really means the person is ingesting or expending 3,000 kcal per day. **Energy Substrates** Energy is released when chemical bonds---the bonds that hold elements together to form molecules---are broken. Substrates are composed primarily of carbon, hydrogen, oxygen, and (in the case of protein) nitrogen. The molecular bonds that hold these elements together are relatively weak and therefore provide little energy when broken. Consequently, food is not used directly for cellular operations. Rather, the energy in food molecular bonds is chemically released within our cells and then stored in the form of the high-energy compound introduced in chapter 1, adenosine triphosphate (ATP), which is discussed in detail later in this chapter. At rest, the energy that the body needs is derived almost equally from the breakdown of carbohydrates and fats. Proteins serve important functions as enzymes that aid chemical reactions and as structural building blocks but usually provide little energy for metabolism. During intense, short-duration muscular effort, more carbohydrate is used, with less reliance on fat to generate ATP. Longer, less intense exercise utilizes carbohydrate and fat for sustained energy production. **Adenosine triphosphate (ATP)** is a complex organic chemical that provides energy to drive many processes in living cells, e.g. muscle contraction, nerve impulse propagation, and chemical synthesis. In our cells, that substance is almost always ATP. Because of its central role in metabolism, ATP frequently referred as the "**Common chemical intermediate**". - The enzyme which help to convert ATP from ADP is Creatin Kinase where the enzyme which convert ATP into ADP is Myosin ATPase. - ATP splits into ADP by water and the reaction is called Hydrolysis - This is produced by Chemical breakdown of ATP - ATP ADP + Pi + Energy - There is a limited supply of ATP in muscles (only stored in muscles) which is depleted within 3-4 sec.  **The standard free energy of ATP is -7.3 Kcal/mol or (-30.88 Kj/mol).** **Carbohydrate** The amount of carbohydrate utilized during exercise is related to both the carbohydrate availability and the muscles' well-developed system for carbohydrate metabolism. All carbohydrates are ultimately converted to the simple six-carbon sugar, glucose, a monosaccharide (one-unit sugar) that is transported through the blood to all body tissues. Under resting conditions, ingested carbohydrate is stored in muscles and liver in the form of a more complex polysaccharide (multiple linked sugar molecules), glycogen. Glycogen is stored in the cytoplasm of muscle ce