Ethiopian Aviation Academy Aviation Maintenance Training ET-PP03.1 Propeller Fundamentals PDF
Document Details
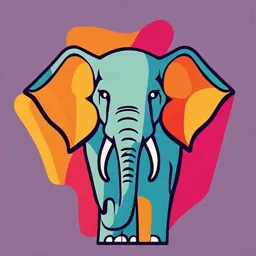
Uploaded by BrightestChimera
National Aviation University
2020
Tags
Summary
This document is a participant manual for Ethiopian Aviation Academy's Aviation Maintenance Training program, focusing on propeller fundamentals. It covers basic propeller theory, materials, and construction for various aircraft types. The manual also details propeller location and installation, along with types of propellers and auxiliary systems.
Full Transcript
Ethiopian Aviation Academy Aviation Maintenance Training ET-PP03.1 Propeller Fundamentals Participant Manual First Edition March 2020 Propeller Fundamentals ---------------------------------------------------- 2...
Ethiopian Aviation Academy Aviation Maintenance Training ET-PP03.1 Propeller Fundamentals Participant Manual First Edition March 2020 Propeller Fundamentals ---------------------------------------------------- 2 Basic Propeller Theory ----------------------------------------------------------------2 Purpose of Propeller -------------------------------------------------------------2 Nomenclature, Terms and Definitions------------------------------------------- 2 Basic Propeller Principles -------------------------------------------------------- 5 Force and Stress on Propeller Blade -------------------------------------------- 8 Propeller Materials and Construction----------------------------------------------- 12 Wooden Propellers ------------------------------------------------------------- 12 Metal Propellers ---------------------------------------------------------------- 13 Composite Propellers ---------------------------------------------------------- 14 Propeller Location and Installation ------------------------------------------------- 15 Propeller Location -------------------------------------------------------------- 15 Propeller installation ----------------------------------------------------------- 17 Types of Propellers------------------------------------------------------------------ 20 Fixed Pitch Propellers ---------------------------------------------------------- 20 Ground-Adjustable Propeller -------------------------------------------------- 21 Controllable Pitch Propeller ---------------------------------------------------- 21 Propeller Governor ------------------------------------------------------------------ 26 Governor Mechanism ---------------------------------------------------------- 28 Under speed Condition --------------------------------------------------------- 30 Over speed Condition ---------------------------------------------------------- 30 On-Speed Condition------------------------------------------------------------ 31 Governor System Operation --------------------------------------------------- 32 Propeller Auxiliary System --------------------------------------------------------- 32 Ice Control Systems ----------------------------------------------------------- 32 Propeller Synchronization and Synchrophasing ------------------------------ 36 Auto feathering System-------------------------------------------------------- 37 Regulation Pertaining to Propellers ------------------------------------------------ 37 Propeller Requirements for Aircraft Certification ----------------------------- 37 Propeller Maintenance Regulations -------------------------------------------- 40 Ethiopian Aviation Academy Aviation Maintenance Revision No. 0 Training March 2020 ET-PP03 M01: Propeller Fundamentals Propeller Fundamentals Basic Propeller Theory Throughout the development of controlled flight as we know it, every aircraft required some kind of device to convert engine power to some form of thrust. Nearly all of the early practical aircraft designs used propellers to create this thrust. As the science of aeronautics progressed, propeller designs improved from flat boards, which merely pushed the air backwards, to airfoil shapes. These airfoils produced lift to pull the aircraft forward through aerodynamic action. As aircraft designs improved, propellers were developed which used thinner airfoil sections and had greater strength. Because of its structural strength, these improvements brought the aluminum alloy propeller into wide usage. The advantage of being able to change the propeller blade angle in flight led to wide acceptance of the two-position propeller and, later, the constant speed propeller system. Today, propeller designs continue to be improved by the use of new composite materials, new airfoil shapes and multi blade configurations. Purpose of Propeller The purpose of the propeller Is to convert engine horsepower to useful thrust. The blades, which are actually rotating wings, have a leading edge, trailing edge, tip, shank, face and Back. Nomenclature, Terms and Definitions Propeller What is a propeller? A propeller normally consists of two or more blades attached to a central hub which is mounted on an engine shaft. Blade is one arm of a propeller from the hub to the tip. Each blade of an aircraft propeller is essentially a rotating wing. As a result of their construction, the propeller blades produce forces that create thrust to pull or push the airplane through the air. Refer figure 1.1 for the terminologies described below. Leading & Trailing Edge The leading edge is the thick edge of the blade hat meets the air as the propeller rotates. Trailing edge is the thin edge at the rear of a propeller blade. Chord and Chord Line The chord line is an imaginary line drawn through the blade from the leading edge to the trailing edge. Issue No. 0 ET-PP03.1 Page 2 of 42 Ethiopian Aviation Academy Aviation Maintenance Revision No. 0 Training March 2020 ET-PP03 M01: Propeller Fundamentals Camber & Mean Camber Line camber is the asymmetry between the two acting surfaces of an airfoil, with the top surface of a wing (or correspondingly the front surface of a propeller blade) commonly being more convex (positive camber). An airfoil that is not cambered is called a symmetric airfoil. Mean Camber line is a line joining the leading and trailing edges of an airfoil equidistant from the upper and lower surfaces. The mean camber line determines the characteristics of the airfoil. Also known as a camber line or a mean line. Thickness The thickness of an airfoil varies along the chord. It may be measured in either of two ways: Thickness measured perpendicular to the camber line. This is sometimes described as the "American convention" Thickness measured perpendicular to the chord line. This is sometimes described as the "British convention". Figure 1-1. Cross-section of a propeller blade. Back and Face Back is the curved side of a propeller airfoil section that can be seen while standing in front of the airplane. Face is the fiat or thrust side of a propeller blade. Shank and Cuff The blade shank is the thick, rounded portion of the propeller blade near the hub and is designed to give strength to the blade. A blade cuff is a metal, wood, or plastic structure designed for attachment to the shank end of the blade, with an outer surface that will transform the round shank into an airfoil section. The cuff is designed primarily to increase the flow of cooling air to the engine nacelle. Butt and Tip The blade butt, also called the blade base or root, is the end of the blade that fits flat side of the propeller blade. blade tip is the opposite end from the root of a propeller blade. Issue No. 0 ET-PP03.1 Page 3 of 42 Ethiopian Aviation Academy Aviation Maintenance Revision No. 0 Training March 2020 ET-PP03 M01: Propeller Fundamentals The central portion of a propeller which is fitted to the engine crankshaft and carries the blades. Refer figure 1.2. Figure 1-2. Basic nomenclature of propellers. Propeller pitch is defined as the distance in inches that a propeller Will move forward ln one revolution. This ls based on the propeller blade angle at the 75% blade station. Geometric Pitch Geometric pitch is the theoretical distance that an aircraft will move forward in one revolution of the propeller. Effective Pitch Effective pitch is the actual distance a propeller advances in one revolution through the air. Propeller Slip Slip is the difference between geometric pitch and effective pitch. Figure 1-3. Effective pitch and geometric pitch. Issue No. 0 ET-PP03.1 Page 4 of 42 Ethiopian Aviation Academy Aviation Maintenance Revision No. 0 Training March 2020 ET-PP03 M01: Propeller Fundamentals Equivalent Shaft Horse Power A unit of measured power output of turboprop or some turboshaft engines. The equivalent shaft horsepower is the sum of shaft horsepower and horsepower derived from jet thrust. The approximate amount of thrust horsepower is found by dividing static thrust by 2.6. Propeller Efficiency The efficiency of a propeller is a measure of the ratio of the thrust horsepower to the torque horsepower. Thrust horsepower acts perpendicular to the plane of propeller rotation and is the amount of power the engine-propeller combination converts into thrust. Torque horsepower, on the other hand, acts in the plane of rotation and is the power used by the engine to rotate the propeller. Basic Propeller Principles The thrust produced by a propeller blade is determined by five things: the shape and area of the airfoil section, the angle of attack, the density of the air, and the speed the airfoil is moving through the air. There are two aspects of the overall theory that explain the operation of a propeller: the momentum theory and blade-element theory. Blade Element Theory The momentum theory considers a propeller blade an airfoil that, when rotated by the engine, produces a pressure differential between its back and face which accelerates and deflects the air. The amount of thrust is determined by the change in the momentum of air passing through the propeller, multiplied by the area of the propeller disc. The blade element theory considers a propeller blade to be made of an infinite number of airfoil sections, with each section located a specific distance from the axis of rotation of the propeller. Each blade element travels at a different speed because of its distance from the center of the hub, and to prevent the thrust from increasing along the length of the blade as its speed increases, the cross-sectional shape of the blade and its blade pitch, or blade angle, vary from a thick, high pitch angle near the low-speed shank to a thin, low pitch angle at the high-speed tip. By using the blade element theory, a propeller designer can select the proper airfoil section and pitch angle to provide the optimum thrust distribution along the blade. Relative Airflow on Blade A knowledge of relative airflow is particularly essential for an understanding of aerodynamics of rotating propeller because relative air flow may be composed of multiple components. Relative air flow is defined as the airflow relative to an airfoil: Relative air flow is created by movement of an airfoil through the air. As an example, consider a person sitting in an automobile on a no-wind day with a hand extended out the window. There is no airflow about the hand since the automobile is not moving. However, if the automobile is driven at 50 miles per hour, the air will flow under and over the hand at 50 miles per hour. A relative wind has been created by moving the hand through the air. Relative Issue No. 0 ET-PP03.1 Page 5 of 42 Ethiopian Aviation Academy Aviation Maintenance Revision No. 0 Training March 2020 ET-PP03 M01: Propeller Fundamentals wind flows in the opposite direction that the hand is moving. The velocity of airflow around the hand in motion is the hand's airspeed. When the aircraft propeller is stationary on a no-wind day, rotational relative wind is produced by rotation of the propeller blades. Since the propeller blades are moving vertically, the effect is to displace some of the air backwards. The blades travel along the same path and pass a given point in rapid succession (a three-bladed system rotating at 320 revolutions per minute passes a given point in the tip-path plane 16 times per second). Figure 1-4. Relative air floe (wind) created opposite to moving airfoil direction Blade Angle, Angle of Attack, Rotational Speed A propeller’s blade angle is the acute angle formed by a propeller’s plane of rotation and the blade’s chord line. The angle of attack of a propeller blade is the acute angle between the chord line of a propeller blade section and the relative wind. Angle of attack relates to the blade pitch angle, but it is not a fixed angle. It varies with the forward speed of the airplane and the RPM of the propeller. The rotational speed of a propeller is determined by multiplying the circumference by engine speed in r.p.m. to find rotational velocity. For example, to determine blade velocity at a point 18 inches from the hub that is rotating at 1800 r.p.m., use the following formula: 2𝜋𝜋𝜋𝜋=203,575 inches per minute. To compensate for the difference in velocity along a propeller blade, the blade angle changes along its length. In addition to blade twist, most propellers have a thicker, low speed airfoil near the blade hub and a thinner, high speed airfoil near the tip. This, along with blade twist, enables the propeller to produce a relatively constant amount of thrust along the entire length of a propeller blade. Issue No. 0 ET-PP03.1 Page 6 of 42 Ethiopian Aviation Academy Aviation Maintenance Revision No. 0 Training March 2020 ET-PP03 M01: Propeller Fundamentals Figure 1-5. Blade angle, Angle of attack and rotational speed of propeller blade. Aerodynamics of propellers A propeller is an airfoil like a wing it will generate an aerodynamic force much the same way. It has a leading and trailing edge, camber and chord. The cambered side is called the back and the flatter side the blade face. Propeller tip speed and efficiency The maximum rotational speed of an engine is limited by propeller tip speed. To produce thrust efficiently, the tip speed of a propeller blade cannot exceed the speed of sound. Keeping in mind that the further a point is from the propeller hub, the faster it moves through the air, high-powered engines operating at high speed must be fitted with short propeller blades or propeller reduction gearing. Reduction gears enable the propeller to turn at a slower, more efficient speed. Blade stations A reference position on a propeller blade that is a specified number of inches from the center of the propeller hub. The typical propeller blade can be described as a twisted airfoil of irregular planform. Two views of a propeller blade are shown in Figure 1.5. For purposes of analysis, a blade can be divided into segments that are located by station numbers in inches from the center of the blade hub. blade station. Issue No. 0 ET-PP03.1 Page 7 of 42 Ethiopian Aviation Academy Aviation Maintenance Revision No. 0 Training March 2020 ET-PP03 M01: Propeller Fundamentals Figure 1-6: Propeller blade stations Pitch distribution and blade design The gradual decrease in blade angle from the hub to the tip is called pitch distribution, or twist. Blade twist enables a propeller to provide a fairly constant angle of attack along most of the length of the blade. Effects of propeller unbalance One of the type of propeller unbalance, aerodynamic unbalance, results when the thrust (or pull) of the blades is unequal. This type of unbalance can be largely eliminated by checking blade contour and blade angle setting. Force and Stress on Propeller Blade The propeller is one of the most highly stressed components in an airplane, and five basic forces act on a propeller turning at a high speed. These are centrifugal force, thrust bending force, torque bending force, aerodynamic twisting force, and centrifugal twisting force. Centrifugal Force Centrifugal force puts the greatest stress on a propeller as it tries to pull the blades out of the hub. It is not uncommon for the centrifugal force to be several thousand times the weight of the blade. For example, a 25-pound propeller blade turning at 2,700 RPM may exert a force of 50 tons (100,000 pounds) on the blade root. Issue No. 0 ET-PP03.1 Page 8 of 42 Ethiopian Aviation Academy Aviation Maintenance Revision No. 0 Training March 2020 ET-PP03 M01: Propeller Fundamentals Thrust Bending Force Thrust bending force is caused by the aerodynamic lift produced by the airfoil shape of the blade as it moves through the air. It tries to bend the blade forward and the force is at its greatest near the tip. Torque Bending Force Torque bending force tries to bend a propeller blade in its plane of rotation opposite to the direction of rotation. Aerodynamic Twisting Moment Aerodynamic twisting (or turning) moment tries to twist a blade to a higher angle. This force is produced because the axis of rotation of the blade is at the midpoint of the chord line, while the center of the lift of the blade is forward of this axis. This force tries to increase the blade angle. Aerodynamic twisting moment is used in some designs to help feather the propeller. Figure 1.6 illustrates how ATM is produced. If the pitch change mechanism is behind the center of pressure (the normal situation) the Total Reaction will tend to try to turn the blade towards a coarse pitch. It should be noted that in the normal forward thrust situation the CTM and ATM oppose each other, but be aware that CTM is a much greater force than ATM and hence CTM will always prevail and try to turn the propeller towards the windmill condition. Centrifugal Twisting Moment Centrifugal twisting (or turning) moment tries to decrease the blade angle, and opposes aerodynamic twisting moment. This tendency to decrease the blade angle is produced since all the parts of a rotating propeller try to move in the same plane of rotation as the blade centerline. This force is greater than the aerodynamic twisting moment at operational RPM and is used in some designs to decrease the blade angle. Figure 1.7 illustrates how the centrifugal force on the blade produces tensile stress at the blade root and a torque about the pitch change axis. The CTM tends to 'fine' the pitch and therefore the effort required by the pitch change mechanism to increase the blade angle towards 'coarse pitch' is increased. Issue No. 0 ET-PP03.1 Page 9 of 42 Ethiopian Aviation Academy Aviation Maintenance Revision No. 0 Training March 2020 ET-PP03 M01: Propeller Fundamentals Figure 1-7. Forces acting on a rotating propeller. Stress (Tensile, Bending, Torsion) A rotating propeller is subjected to many forces that cause tension, twisting, and bending stresses. Of all the forces acting on a propeller, centrifugal force causes the greatest stress. Centrifugal force can be described as the force tending to pull the blades out of the hub. Therefore, the force that causes high tensile stress near to the root of the propeller blades is centrifugal force. Thrust bending force tends to bend the propeller blades forward at the tips because the lift toward the tip of the blade flexes the thin blade sections forward. On the other hand, Torque bending force is a force which tends to bend the propeller blade back in the direction opposite to the direction of rotation. Therefore, both thrust bending and torque bending forces induce the highest bending stress near to the root of the blades of a propeller. Aerodynamic twisting moment tries to twist a blade to a higher angle by aerodynamic action. On the other hand, centrifugal twisting moment tends to decrease blade angle and opposes aerodynamic twisting moment. The resulting twisting moment will induce the torsion stress on each section of propeller blades. Vibration stress and forces When a propeller is producing thrust, aerodynamic and mechanical forces are present which cause the blades to vibrate. If not compensated for in the design, these vibrations may cause excessive flexing. work hardening of the metal, and result in section of the propeller blade breaking off during operation. Therefore, the vibration stress are due to the aerodynamic force fluctuating and mechanical forces of engine operations causes vibration and fatigue load to induce stresses in the blades and hub of the propeller. Gyroscopic effect A rotating propeller has the properties of a gyro. If the plane of rotation is changed, a moment will be produced at right angles to the applied moment. For example, if an aircraft with a right handed propeller is yawed to the right it will experience a nose down pitching moment due to the gyroscopic effect of the propeller. Similarly, if the aircraft is pitched nose up, it will experience a yaw to the right. On most aircraft, the gyroscopic effects are small and easily controlled. Issue No. 0 ET-PP03.1 Page 10 of 42 Ethiopian Aviation Academy Aviation Maintenance Revision No. 0 Training March 2020 ET-PP03 M01: Propeller Fundamentals The property of a gyroscope that is discussed above is known as precession. See figure 1.7. If a torque is applied as shown, then precession will occur as shown. Direction of precession can be determined by taking the force causing the torque and rotating it through 90° in the direction of rotation. Figure 1-8. Gyroscopic Effect Asymmetric effect In general, the axis of the propeller will be inclined upwards to the direction of flight due to the angle of attack of the aircraft. This causes the downward moving blade to have a greater effective angle of attack than the upward moving blade and therefore to develop a greater thrust. The difference in thrust on the two sides of the propeller disc causes a yawing moment. For a right-handed propeller in a nose-up attitude, the yaw will be to the left. Figure 1-9. Asymmetric Effect (P-Factor) Issue No. 0 ET-PP03.1 Page 11 of 42 Ethiopian Aviation Academy Aviation Maintenance Revision No. 0 Training March 2020 ET-PP03 M01: Propeller Fundamentals Vibration and Resonance When a propeller is producing thrust, aerodynamic and mechanical forces are present which cause the blades of the propeller to vibrate (see figure 1.9). A person designing a propeller must take this into consideration. If this is not done, these vibrations may cause excessive flexing, hardening of the metal and could result in sections of the propeller breaking off during operation. Aerodynamic forces have a great vibration effect at the tip of the blade where the effects of transonic speeds cause buffeting and vibrations. Mechanical vibrations are caused by power pulses in a piston engine and are more destructive then aerodynamic vibrations. Figure 1-10. Propeller Vibration Propeller Materials and Construction Almost all propellers produced are made of wood, steel, aluminum, composite materials or a combination of materials. In the early years of aircraft development, all propellers were made of wood. Due to wood’s susceptibility to damage, steel propellers became prevalent. Today, aluminum alloys and composite materials are used for both fixed and adjustable-pitch propellers. Wooden Propellers Wood propellers have been used since the Wright Flyer's first flight in 1903 and are still popular on many amateur-built airplanes. Although many of the wood propellers were used on older airplanes, some are still in use. The construction of a fixed pitch, wooden propeller is such that its blade pitch cannot be changed after manufacture. The choice of the blade angle is decided by the normal use of the propeller on an aircraft during level flight when the engine performs at maximum efficiency. The impossibility of changing the blade pitch on the fixed-pitch propeller restricts its use to small aircraft with low horsepower engines in which maximum engine efficiency during all flight conditions is of lesser importance than in larger aircraft. The wooden, fixed-pitch propeller is well suited for such small aircraft because of its light weight, rigidity, economy of production, simplicity of construction, and ease of replacement. A wooden propeller is not constructed from a solid block, but is built up of a number of separate layers of carefully selected and well-seasoned hardwoods. Many woods, such as Issue No. 0 ET-PP03.1 Page 12 of 42 Ethiopian Aviation Academy Aviation Maintenance Revision No. 0 Training March 2020 ET-PP03 M01: Propeller Fundamentals mahogany, cherry, black walnut, and oak, are used to some extent, but birch is the most widely used. Five to nine separate layers are used, each about 3⁄4 inch thick. The several layers are glued together with a waterproof, resinous glue and allowed to set. The blank is then roughed to the approximate shape and size of the finished product. The roughed-out propeller is then allowed to dry for approximately one week to permit the moisture content of the layers to become equalized. Figure 1-11. Fixed-pitch wooden propeller assembly. Metal Propellers Improvements in metallurgy and manufacturing techniques have enabled metal propellers to replace wood propellers for modem commercially manufactured airplanes. Metal propellers are forged from high-strength aluminum alloy, and after being ground to their finished dimensions and pitch, are anodized to protect them from corrosion. Metal propellers cost more than wood for the same engine and airplane, but their increased durability, resistance to weathering, and ability to be straightened after minor damage have made them more cost effective in the long run. Figure 1-12. Fixed-pitch metallic propeller. Issue No. 0 ET-PP03.1 Page 13 of 42 Ethiopian Aviation Academy Aviation Maintenance Revision No. 0 Training March 2020 ET-PP03 M01: Propeller Fundamentals Aluminum Alloy Propellers Aluminum propellers are the most widely used types of propeller in aviation. They provide better engine cooling by carrying the airfoil sections closer to the hub and directing more air over the engine. Aluminum propellers are made from aluminum alloy and are finished to the desired airfoil shape by machining and manual grinding. Twisting the blades to the desired angles sets the pitch. Once the propeller is ground to the desired contour, it must then be balanced. This is done by removing some metal from the tip of the blade. After the propeller is balanced the surfaces are finished by plating, chemical etching and or painting. Anodizing is the most commonly used finishing process. Steel Propellers Steel propellers are found primarily on transport aircraft. They are normally of hollow construction, which helps to reduce weight. Solid steel propellers are forged and machined to the desired contours and the proper twist is achieved by twisting the blades. Hollow steel blades are constructed by assembling a rib structure, attaching steel sheets to the structure, and filling the outer section of the blade with a foam material to absorb vibration and maintain a rigid structure. Composite Propellers Laminated wood, forged aluminum alloy, and brazed sheet steel propellers have been the standard for decades. But the powerful turbo-propeller engines and the demands for higher speed flight and quieter operation have caused propeller manufacturers to exploit the advantages of modem advanced composite materials. Composite materials used in propeller manufacturing consist of two constituents: the fibers and the matrix. The fibers most generally used are glass, graphite, and aramid (Kevlar), and the matrix is a thermosetting resin such as epoxy. The strength and stiffness of the blades are determined by the material, diameter, and orientation of the fibers. The matrix material supports the fibers, holds them in place, and completely encapsulates them to protect them from the environment. Issue No. 0 ET-PP03.1 Page 14 of 42 Ethiopian Aviation Academy Aviation Maintenance Revision No. 0 Training March 2020 ET-PP03 M01: Propeller Fundamentals Figure 1-13. Composite blade construction Propeller Location and Installation Propeller Location Tractor Propellers Tractor propellers are those mounted on the upstream end of a drive shaft in front of the supporting structure. Most aircraft are equipped with this type of propeller. The tractor type of propeller comes in all types of propellers. A major advantage of the tractor propeller is that lower stresses are induced in the propeller as it rotates in relatively undisturbed air. Figure 1-14. Tractor propeller: Diamond 40 NG Issue No. 0 ET-PP03.1 Page 15 of 42 Ethiopian Aviation Academy Aviation Maintenance Revision No. 0 Training March 2020 ET-PP03 M01: Propeller Fundamentals Figure 1-15 Tractor propeller configuration: Dash 8 Q400 Pusher Propellers Pusher propellers are those mounted on the downstream end of a drive shaft behind the supporting structure. Pusher propellers are constructed as fixed- or variable-pitch propellers. Seaplanes and amphibious aircraft have used a greater percentage of pusher propellers than other kinds of aircraft. On land planes, where propeller-to-ground clearance usually is less than propeller-to-water clearance of watercraft, pusher propellers are subject to more damage than tractor propellers. Rocks, gravel, and small objects dislodged by the wheels are quite often thrown or drawn into a pusher propeller. Similarly, planes with pusher propellers are apt to encounter propeller damage from water spray thrown up by the hull during landing or takeoff airspeed. Consequently, the pusher propeller is mounted above and behind the wings to prevent such damage. Figure 1-16. Pusher propeller configuration: Beech Starship Issue No. 0 ET-PP03.1 Page 16 of 42 Ethiopian Aviation Academy Aviation Maintenance Revision No. 0 Training March 2020 ET-PP03 M01: Propeller Fundamentals Propeller installation Installation and removal procedures for a propeller depend upon the type of propeller shaft, and there are three types found on reciprocating engines: flanged, splined, and tapered. The larger turboprop engines have splined shafts, and the smaller ones have flanged shafts. Flanged Shaft Most modern engines, both reciprocating and turbine, have flanged crankshafts or propeller shafts. Some of these flanges have integral internally threaded bushings that fit into counter bores in the rear of the propeller hub around each bolt hole. Propellers with these bushings are attached to the shaft with long bolts that pass through the propeller. A stub shaft, or pilot, fits into the center bore of the propeller to center it on the shaft. Figure 1.17 shows a cross section of the flanged crankshaft of a direct-drive reciprocating engine. Notice that this shaft is fitted for a fixed-pitch propeller. If a constant speed propeller were installed, the expansion plug in the end of the shaft would be removed so propeller control oil from the governor could flow into and out of the propeller. Figure 1-17. Propeller flange on the crankshaft of a direct crank reciprocating engine Issue No. 0 ET-PP03.1 Page 17 of 42 Ethiopian Aviation Academy Aviation Maintenance Revision No. 0 Training March 2020 ET-PP03 M01: Propeller Fundamentals Figure 1-18. Flanged propeller shaft for a turboprop engine Tapered Shaft Some small engines have a tapered crankshaft onto which the propeller is locked with a steel key fitting in a keyway slot cut into the tapered surface. Most propellers installed on tapered shafts are made of wood, which requires a steel hub with a tapered hole and a keyway slot cut into it. The hub is installed in the propeller and fitted to the tapered shaft. With the key installed in the slot in the shaft, apply Prussian blue transfer dye to the shaft and install the hub, tightening the retaining nut to the required torque. Remove the nut and hub, and note the amount of dye that has transferred to the taper inside the hub. If less than 70% of the surface is covered, remove the key and lap the hub to the shaft, using a fine-grit lapping compound. Be sure to follow the engine manufacturer's instructions in detail when lapping the hub to the shaft. When the lapping is completed, clean the hub and shaft to remove all traces of lapping compound and install the propeller. Figure 1-19. Tapered propeller shaft Issue No. 0 ET-PP03.1 Page 18 of 42 Ethiopian Aviation Academy Aviation Maintenance Revision No. 0 Training March 2020 ET-PP03 M01: Propeller Fundamentals Splined Shaft The most popular type of propeller shaft on reciprocating engines built through World War II and on the larger turboprop engines is the splined shaft. The sizes of splined shafts are identified by an SAE (Society of Automotive Engineers) number. SAE 20 splines are used on engines in the 200-horsepower range; SAE 30 splines are used in the 300-and 400- horsepower range, and SAE 40 in the 500- and 600-horsepower range. SAE 50 in the 1,000- horsepower range and SAE 60 and 70 are used for larger engines. Splines are longitudinal grooves cut in the periphery of the shaft. The grooves and lands (the space between the grooves) are the same size, and one groove is either missing or has a screw in it to form a master spline. The purpose of the master spline is to ensure the propeller's correct installation in relation to the throws of the crankshaft. This orientation is critical to minimize vibration. See Figure 1.20. The inside of the propeller hub is splined to match the shaft, and the hub is centered on the shaft with two cones. The rear cone is a single-piece split bronze cone, and is considered to be part of the engine. The front cone is a two-piece hardened steel cone and is considered to be part of the propeller. The two halves are marked with the same serial number to ensure that only a matched set is used. Figure 1-20. Splined propeller shaft showing the master spline Issue No. 0 ET-PP03.1 Page 19 of 42 Ethiopian Aviation Academy Aviation Maintenance Revision No. 0 Training March 2020 ET-PP03 M01: Propeller Fundamentals Propeller Cones To help ensure that the propeller hub is centered on the crankshaft, a front and rear cone are installed on each side of the propeller hub. The rear cone is typically made of bronze and is split to allow flexibility during installation and to ensure a tight fit. The front cone, on the other hand, is made in two pieces as a matched set. The two halves are marked with a serial number to identify them as a set as shown in figure 1-21. Figure. 1-21. Propeller shaft cones. Types of Propellers There are various types or classes of propellers, the simplest of which are the fixed-pitch and ground-adjustable propellers. The complexity of propeller systems increases from these simpler forms to controllable-pitch and complex automatic systems. Various characteristics of several propeller types are discussed in the following paragraphs, but no attempt is made to cover all types of propellers. Fixed Pitch Propellers As the name implies, a fixed-pitch propeller has the blade pitch, or blade angle, built into the propeller. The blade angle cannot be changed after the propeller is built. Generally, this type of propeller is one piece and is constructed of laminated wood or aluminum alloy. Fixed-pitch propellers are designed for best efficiency at one rotational and forward speed, usually cruise speed. They are rather inefficient at take-off and landing speeds. They are designed to fit a set of conditions of both aircraft and engine speeds, and any change in these conditions reduces the efficiency of both the propeller and the engine. The fixed-pitch propeller is used on aircraft of low power, speed, range, or altitude. Issue No. 0 ET-PP03.1 Page 20 of 42 Ethiopian Aviation Academy Aviation Maintenance Revision No. 0 Training March 2020 ET-PP03 M01: Propeller Fundamentals Ground-Adjustable Propeller The ground-adjustable propeller operates as a fixed-pitch propeller. The pitch or blade angle can only be changed when the propeller is not turning. It is done by loosening the clamping mechanism, which holds the blades in place. After the clamping mechanism has been tightened, the pitch of the blades cannot be changed in flight to meet variable flight requirements. Like the fixed-pitch propeller, the ground-adjustable propeller is used on aircraft of low power, speed, range, or altitude. A ground adjustable propeller may have blades made of wood or metal. The hub is usually of two-piece steel construction with clamps or large nuts used to hold the blade securely in place. When the angle of the blade is to be changed, the clamp or blade nuts are loosened and the blades rotated to the desired angle as indicated by a propeller protractor. The angle markings on the hub are not considered accurate enough to provide a good reference for blade adjustment, so they are only used for reference. Controllable Pitch Propeller The controllable-pitch propeller permits a change of blade pitch, or angle, while the propeller is rotating. This allows the propeller to assume a blade angle that gives the best performance for particular flight conditions. The number of pitch positions may be limited, as with a two- position controllable propeller, or the pitch may be adjusted to any angle between the minimum and maximum pitch settings of a given propeller. The use of controllable-pitch propellers also makes it possible to attain the desired engine rpm for a particular flight condition. This type of propeller is not to be confused with a constant speed propeller. With the controllable-pitch type, the blade angle can be changed in flight, but the pilot must change the propeller blade angle directly. The blade angle will not change again until the pilot changes it. The use of a governor is the next step in the evolution of propeller development, making way for constant-speed propellers with governor systems. An example of a two-position propeller is a Hamilton Standard counterweight two-position propeller. Two Position Propellers One of the first controllable-pitch propellers to see widespread use was the Hamilton- Standard counterweight propeller. This propeller, developed in the 1930’s, permitted a pilot to select low pitch or high pitch. The low pitch setting was used during takeoff and climb, so the engine operated at its maximum speed and developed its full rate horsepower. The high pitch setting was used to gain more efficient operation and improved fuel economy during cruise flight. Similar to a ground-adjustable propeller, the Hamilton-Standard propeller uses a two-piece hub to hold the propeller blades in place. To enable the propeller blades to rotate between the low and high pitch stops, each blade rides on a set of roller bearings. In addition, a counterweight bracket is installed at the base of each propeller blade. Issue No. 0 ET-PP03.1 Page 21 of 42 Ethiopian Aviation Academy Aviation Maintenance Revision No. 0 Training March 2020 ET-PP03 M01: Propeller Fundamentals The blade angle on the Hamilton-Standard propeller is changed by using a combination of hydraulic and centrifugal forces. Hydraulic force decreases blade angle while centrifugal force acts on a set of counterweights to increase blade angle. The hydraulic force that decreases blade angle is derived from engine oil that flows out of the crankshaft and acts on a piston assembly on the front of the propeller hub. The flow of engine oil into the piston assembly is controlled by a three-way selector valve that is mounted in the engine and controlled from the cockpit. When this valve moves forward to decrease propeller blade angle, it routes engine oil into the piston assembly to force the piston outward. The piston assembly is linked to each counterweight bracket so that, as the piston moves out, it pulls the counterweights in and decreases blade angle. After the blades reach their low pitch stop in the counterweight assembly, oil pressure holds the blades in this position. Figure 1-22. When low pitch is selected, engine oil pressure forces the cylinder forward. This motion moves the counterweights and blades to the low pitch position. To move the blades to a high pitch position, the propeller control lever is moved aft, which rotates the selector valve to release oil pressure in the propeller hub. With no oil pressure to counteract it, the centrifugal force acting on the counterweights moves them outward, rotating the blades to their high pitch position. As the blades rotate, oil is forced out of the propeller cylinder and returned to the engine sump. The blades stop rotating when they contact their high pitch stops located in the counterweight assembly. The low pitch stop on a constant speed propeller is usually set so that the engine will turn at its rated takeoff RPM at sea level when the throttle is opened to allowable manifold pressure. Issue No. 0 ET-PP03.1 Page 22 of 42 Ethiopian Aviation Academy Aviation Maintenance Revision No. 0 Training March 2020 ET-PP03 M01: Propeller Fundamentals Figure 1-23. When high pitch is selected, engine oil pressure is removed from the piston assembly, which permits centrifugal force to move the counterweights outward. This rotates the blades to the high pitch position. In most cases, the pitch stops of a two-position propeller can be adjusted. This adjustment can be made only when the engine is not operating. To do this, rotate a pitch stop adjusting nut until the desired blade angle is obtained. The operation of a two-position propeller is straightforward. Prior to engine shutdown, the propeller should be placed in its high pitch position. Doing so retracts the piston assembly, protecting it from corrosion and accumulation of dirt. Furthermore, most of the oil is forced from the piston, where it could otherwise congeal in cold weather. Constant Speed Propeller The propeller has a natural tendency to slow down as the aircraft climbs and to speed up as the aircraft dives because the load on the engine varies. To provide an efficient propeller, the speed is kept as constant as possible. By using propeller governors to increase or decrease propeller pitch, the engine speed is held constant. When the airplane goes into a climb, the blade angle of the propeller decreases just enough to prevent the engine speed from decreasing. The engine can maintain its power output if the throttle setting is not changed. When the airplane goes into a dive, the blade angle increases sufficiently to prevent over speeding and, with the same throttle setting, the power output remains unchanged. If the throttle setting is changed instead of changing the speed of the airplane by climbing or diving, the blade angle increases or decreases as required to maintain a constant engine rpm. The power output (not the rpm) changes in accordance with changes in the throttle setting. The Issue No. 0 ET-PP03.1 Page 23 of 42 Ethiopian Aviation Academy Aviation Maintenance Revision No. 0 Training March 2020 ET-PP03 M01: Propeller Fundamentals governor-controlled, constant-speed propeller changes the blade angle automatically, keeping engine rpm constant. One type of pitch-changing mechanism is operated by oil pressure (hydraulically) and uses a piston-and-cylinder arrangement. The piston may move in the cylinder, or the cylinder may move over a stationary piston. The linear motion of the piston is converted by several different types of mechanical linkage into the rotary motion necessary to change the blade angle. The mechanical connection may be through gears, the pitch-changing mechanism that turns the butt of each blade. Each blade is mounted with a bearing that allows the blade to rotate to change pitch as shown in figure 1-24. Figure 1-24. Blade bearing areas in hub. In most cases, the oil pressure for operating the different types of hydraulic pitch-changing mechanisms comes directly from the engine lubricating system. When the engine lubricating system is used, the engine oil pressure is usually boosted by a pump that is integral with the governor to operate the propeller. The higher oil pressure (approximately 300 pounds per square inch (psi)) provides a quicker blade-angle change. The governors direct the pressurized oil for operation of the hydraulic pitch-changing mechanisms. The governors used to control hydraulic pitch-changing mechanisms are geared to the engine crankshaft and are sensitive to changes in rpm. When rpm increases above the value for which a governor is set, the governor causes the propeller pitch-changing mechanism to turn the blades to a higher angle. This angle increases the load on the engine, and rpm decreases. When rpm decreases below the value for which a governor is set, the governor causes the pitch changing mechanism to turn the blades to a lower angle; the load on the engine is decreased, and rpm increases. Thus, a propeller governor tends to keep engine rpm constant. Issue No. 0 ET-PP03.1 Page 24 of 42 Ethiopian Aviation Academy Aviation Maintenance Revision No. 0 Training March 2020 ET-PP03 M01: Propeller Fundamentals In constant-speed propeller systems, the control system adjusts pitch through the use of a governor, without attention by the pilot, to maintain a specific preset engine rpm within the set range of the propeller. For example, if engine speed increases, an over speed condition occurs and the propeller needs to slow down. The controls automatically increase the blade angle until desired rpm has been reestablished. A good constant speed control system responds to such small variations of rpm that for all practical purposes, a constant rpm is maintained. Each constant-speed propeller has an opposing force that operates against the oil pressure from the governor. Flyweights mounted to the blades move the blades in the high pitch direction as the propeller turns. Other forces used to move the blades toward the high pitch direction include air pressure (contained in the front dome), springs, and aerodynamic twisting moment. Feathering Propellers Feathering propellers must be used on multi-engine aircraft to reduce propeller drag to a minimum under one or more engine failure conditions. A feathering propeller is a constant- speed propeller used on multi-engine aircraft that has a mechanism to change the pitch to an angle of approximately 90°. A propeller is usually feathered when the engine fails to develop power to turn the propeller. By rotating the propeller blade angle parallel to the line of flight, the drag on the aircraft is greatly reduced. With the blades parallel to the airstream, the propeller stops turning and minimum wind milling, if any, occurs. The blades are held in feather by aerodynamic forces. Almost all small feathering propellers use oil pressure to take the propeller to low pitch and blade flyweights, springs, and compressed air to take the blades to high pitch. Since the blades would go to the feather position during shutdown, latches lock the propeller in the low pitch position as the propeller slows down at shutdown as shown figure 1-25. These can be internal or external and are contained within the propeller hub. In flight, the latches are prevented from stopping the blades from feathering because they are held off their seat by centrifugal force. Latches are needed to prevent excess load on the engine at start up. If the blade were in the feathered position during engine start, the engine would be placed under an undue load during a time when the engine is already subject to wear. Issue No. 0 ET-PP03.1 Page 25 of 42 Ethiopian Aviation Academy Aviation Maintenance Revision No. 0 Training March 2020 ET-PP03 M01: Propeller Fundamentals Figure 1-25. Feathering latches. Reverse-Pitch Propellers Additional refinements, such as reverse-pitch propellers (mainly used on turbo props), are included in some propellers to improve their operational characteristics. Almost all reverse- pitch propellers are of the feathering type. A reverse pitch propeller is a controllable propeller in which the blade angles can be changed to a negative value during operation. The purpose of the reversible pitch feature is to produce a negative blade angle that produces thrust opposite the normal forward direction. Normally, when the landing gear is in contact with the runway after landing, the propellers blades can be moved to negative pitch (reversed), which creates thrust opposite of the aircraft direction and slows the aircraft. As the propeller blades move into negative pitch, engine power is applied to increase the negative thrust. This aerodynamically brakes the aircraft and reduces ground roll after landing. Reversing the propellers also reduces aircraft speed quickly on the runway just after touchdown and minimizes aircraft brake wear. Propeller Governor A governor is an engine rpm-sensing device and high pressure oil pump. In a constant-speed propeller system, the governor responds to a change in engine rpm by directing oil under pressure to the propeller hydraulic cylinder or by releasing oil from the hydraulic cylinder. The change in oil volume in the hydraulic cylinder changes the blade angle and maintains the propeller system rpm. The governor is set for a specific rpm via the cockpit propeller control, which compresses or releases the governor speeder spring. Issue No. 0 ET-PP03.1 Page 26 of 42 Ethiopian Aviation Academy Aviation Maintenance Revision No. 0 Training March 2020 ET-PP03 M01: Propeller Fundamentals A propeller governor is used to sense propeller and engine speed and normally provides oil to the propeller for low pitch position. There are a couple of non-feathering propellers that operate opposite to this. Fundamental forces, some already discussed, are used to control blade angle variations required for constant-speed propeller operation. These forces are: 1. Centrifugal twisting moment—a component of the centrifugal force acting on a rotating blade that tends at all times to move the blade into low pitch. 2. Propeller-governor oil on the propeller piston side—balances the propeller blade flyweights, which moves the blades toward high pitch. 3. Propeller blade flyweights—always move the blades toward high pitch. 4. Air pressure against the propeller piston—pushes toward high pitch. 5. Large springs—push in the direction of high pitch and feather. 6. Centrifugal twisting force—moves the blades toward low pitch. 7. Aerodynamic twisting force—moves the blades toward high pitch. All of the forces listed are not equal in strength. The most powerful force is the governor oil pressure acting on the propeller piston. This piston is connected mechanically to the blades; as the piston moves, the blades are rotated in proportion. By removing the oil pressure from the governor, the other forces can force the oil from the piston chamber and move the propeller blades in the other direction. Figure 1-26. Parts of a governor. Issue No. 0 ET-PP03.1 Page 27 of 42 Ethiopian Aviation Academy Aviation Maintenance Revision No. 0 Training March 2020 ET-PP03 M01: Propeller Fundamentals Governor Mechanism The engine-driven single-acting propeller governor (constant-speed control) receives oil from the lubricating system and boosts its pressure to that required to operate the pitch-changing mechanism. It consists of a gear pump to increase the pressure of the engine oil, a pilot valve controlled by flyweights in the governor to control the flow of oil through the governor to and away from the propeller, and a relief valve system that regulates the operating oil pressures in the governor. A spring called the speeder spring opposes the governor flyweight’s ability to fly outward when turning. The tension on this spring can be adjusted by the propeller control on the control quadrant. The tension of the speeder spring sets the maximum rpm of the engine in the governor mode. As the engine and propeller rpm is increased at the maximum set point (maximum speed) of the governor, the governor flyweights overcome the tension of the speeder spring and move outward. This action moves the pilot valve in the governor to release oil from the propeller piston and allows the blade flyweights to increase blade pitch, which increases the load on the engine, slowing it down or maintaining the set speed. Figure 1-27 shows typical propeller governor. Issue No. 0 ET-PP03.1 Page 28 of 42 Ethiopian Aviation Academy Aviation Maintenance Revision No. 0 Training March 2020 ET-PP03 M01: Propeller Fundamentals Figure 1-27. Typical governor. Issue No. 0 ET-PP03.1 Page 29 of 42 Ethiopian Aviation Academy Aviation Maintenance Revision No. 0 Training March 2020 ET-PP03 M01: Propeller Fundamentals Under speed Condition When the engine is operating below the rpm set by the pilot using the cockpit control, the governor is operating in an under speed condition. In this condition, the flyweights tilt inward because there is not enough centrifugal force on the flyweights to overcome the force of the speeder spring. The pilot valve, forced down by the speeder spring, meters oil flow to decrease propeller pitch and raise engine rpm. If the nose of the aircraft is raised or the blades are moved to a higher blade angle, this increases the load on the engine and the propeller tries to slow down. To maintain a constant speed, the governor senses the decrease in speed and increases oil flow to the propeller, moving the blades to a lower pitch and allowing them to maintain the same speed. When the engine speed starts to drop below the rpm for which the governor is set, the resulting decrease in centrifugal force exerted by the flyweights permits the speeder spring to lower the pilot valve (flyweights inward) as shown in figure 1-28, thereby opening the propeller-governor metering port. The oil then flows through the valve port and into the propeller piston causing the blades to move to a lower pitch (a decrease in load). Figure 1-28. Underspeed condition. Over speed Condition When the engine is operating above the rpm set by the pilot using the cockpit control, the governor is operating in an over speed condition. In an over speed condition, the centrifugal force acting on the flyweights is greater than the speeder spring force. The flyweights tilt outward and raise the pilot valve as shown in figure 1-29. The pilot valve then meters oil flow to increase propeller pitch and lower engine rpm. When the engine speed increases above the rpm for which the governor is set, note that the flyweights move outward against the force of the speeder spring, raising the pilot valve. This opens the propeller-governor metering port, allowing governor oil flow from the propeller piston allowing counterweights on the blades to increase pitch and slow the engine. Issue No. 0 ET-PP03.1 Page 30 of 42 Ethiopian Aviation Academy Aviation Maintenance Revision No. 0 Training March 2020 ET-PP03 M01: Propeller Fundamentals Figure 1-29. Overspeed condition. On-Speed Condition When the engine is operating at the rpm set by the pilot using the cockpit control, the governor is operating on speed. In an on-speed condition, the centrifugal force acting on the flyweights is balanced by the speeder spring, and the pilot valve is neither directing oil to nor from the propeller hydraulic cylinder. In the on-speed condition, the forces of the governor flyweights and the tension on the speeder spring are equal; the propeller blades are not moving or changing pitch. If something happens to unbalance these forces, such as if the aircraft dives or climbs, or the pilot selects a new rpm range through the propeller control (changes tension on the speeder spring), then these forces are unequal and an under speed or over speed condition would result. A change in rpm comes about in the governing mode by pilot selection of a new position of the propeller control, which changes the tension of the governor speeder spring or by the aircraft changing attitude. The governor, as a speed- sensing device, causes the propeller to maintain a set rpm regardless of the aircraft attitude. The speeder spring propeller governing range is limited to about 200 rpm. Beyond this rpm, the governor cannot maintain the correct rpm. Figure 1-30. On-speed condition. Issue No. 0 ET-PP03.1 Page 31 of 42 Ethiopian Aviation Academy Aviation Maintenance Revision No. 0 Training March 2020 ET-PP03 M01: Propeller Fundamentals Governor System Operation If the engine speed drops below the rpm for which the governor is set, the rotational force on the engine-driven governor flyweights becomes less. This allows the speeder spring to move the pilot valve downward figure 1-28. With the pilot valve in the downward position, oil from the gear type pump flows through a passage to the propeller and moves the cylinder outward. This in turn decreases the blade angle and permits the engine to return to the on-speed setting. If the engine speed increases above the rpm for which the governor is set, the flyweights move against the force of the speeder spring and raise the pilot valve. This permits the oil in the propeller to drain out through the governor drive shaft. As the oil leaves the propeller, the centrifugal force acting on the counterweights turns the blades to a higher angle, which decreases the engine rpm. When the engine is exactly at the rpm set by the governor, the centrifugal reaction of the flyweights balances the force of the speeder spring, positioning the pilot valve so that oil is neither supplied to nor drained from the propeller. With this condition, propeller blade angle does not change. Note that the rpm setting is made by varying the amount of compression in the speeder spring. Positioning of the speeder rack is the only action controlled manually. All others are controlled automatically within the governor. Propeller Auxiliary System Two systems that enable the propeller to operate more efficiently are the synchronizer system and its elaboration, the synchrophaser, and the ice control system which includes anti-icing and deicing. Ice Control Systems Ice formation on a propeller blade, in effect, produces a distorted blade airfoil section that causes a loss in propeller efficiency. Generally, ice collects asymmetrically on a propeller blade and produces propeller unbalance and destructive vibration and increases the weight of the blades. Anti-Icing Systems A typical fluid system includes a tank to hold a supply of anti-icing fluid shown in figure 1-31. This fluid is forced to each propeller by a pump. The control system permits variation in the pumping rate so that the quantity of fluid delivered to a propeller can be varied, depending on the severity of icing. Fluid is transferred from a stationary nozzle on the engine nose case into a circular U-shaped channel (slinger ring) mounted on the rear of the propeller assembly. The fluid under pressure of centrifugal force is transferred through nozzles to each blade shank. Issue No. 0 ET-PP03.1 Page 32 of 42 Ethiopian Aviation Academy Aviation Maintenance Revision No. 0 Training March 2020 ET-PP03 M01: Propeller Fundamentals Figure 1-31. Typical propeller fluid anti-icing system. Because airflow around a blade shank tends to disperse anti-icing fluids to areas where ice does not collect in large quantities, feed shoes, or boots, are installed on the blade leading edge. These feed shoes are a narrow strip of rubber extending from the blade shank to a blade station that is approximately 75 percent of the propeller radius. The feed shoes are molded with several parallel open channels in which fluid flows from the blade shank toward the blade tip by centrifugal force. The fluid flows laterally from the channels over the leading edge of the blade. Isopropyl alcohol is used in some anti-icing systems because of its availability and low cost. Phosphate compounds are comparable to isopropyl alcohol in anti-icing performance and have the advantage of reduced flammability. However, phosphate compounds are comparatively expensive and, consequently, are not widely used. This system has disadvantages in that it requires several components that add weight to the aircraft, and the time of anti-ice available is limited to the amount of fluid on board. This system is not used on modern aircraft, giving way to the electric deicing systems. Deicing Systems An electric propeller-icing control system consists of an electrical energy source, a resistance heating element, system controls, and necessary wiring shown in figure 1-32. The heating elements are mounted internally or externally on the propeller spinner and blades. Electrical power from the aircraft system is transferred to the propeller hub through electrical leads, which terminate in slip rings and brushes. Flexible connectors are used to transfer power from the hub to the blade elements. Issue No. 0 ET-PP03.1 Page 33 of 42 Ethiopian Aviation Academy Aviation Maintenance Revision No. 0 Training March 2020 ET-PP03 M01: Propeller Fundamentals Figure 1-32. Typical electrical deicing system. A deice system consists of one or more on-off switches. The pilot controls the operation of the deice system by turning on one or more switches. All deice systems have a master switch, and may have another toggle switch for each propeller. Some systems may also have a selector switch to adjust for light or heavy icing conditions or automatic switching for icing conditions. The timer or cycling unit determines the sequence of which blades (or portion thereof) are currently being deiced, and for what length of time. The cycling unit applies power to each deice boot, or boot segment, in a sequence or all on order. A brush block, which is normally mounted on the engine just behind the propeller, is used to transfer electricity to the slip ring. A slip ring and brush block assembly is shown in figure 1- 33. The slip ring rotates with the propeller and provides a current path to the blade deice boots. A slip ring wire harness is used on some hub installations to electrically connect the slip ring to the terminal strip connection screw. Issue No. 0 ET-PP03.1 Page 34 of 42 Ethiopian Aviation Academy Aviation Maintenance Revision No. 0 Training March 2020 ET-PP03 M01: Propeller Fundamentals Figure 1-33. Deicing brush block and slip ring assembly. A deice wire harness is used to electrically connect the deice boot to the slip ring assembly. A deice boot contains internal heating elements or dual elements. The boot is securely attached to the leading edge of each blade with adhesive as shown in figure 1-34. Electric deicing systems are usually designed for intermittent application of power to the heating elements to remove ice after formation but before excessive accumulation. Proper control of heating intervals aids in preventing runback, since heat is applied just long enough to melt the ice face in contact with the blade. Figure 1-34. Electric deice boot. Issue No. 0 ET-PP03.1 Page 35 of 42 Ethiopian Aviation Academy Aviation Maintenance Revision No. 0 Training March 2020 ET-PP03 M01: Propeller Fundamentals Cycling timers are used to energize the heating element circuits for periods of 15 to 30 seconds, with a complete cycle time of 2 minutes. A cycling timer is an electric motor driven contactor that controls power contactors in separate sections of the circuit. Controls for propeller electrical deicing systems include on-off switches, ammeters or load meters to indicate current in the circuits, and protective devices, such as current limiters or circuit breakers. The ammeters or load meters permit monitoring of individual circuit currents and reflect operation of the timer. To prevent element overheating, the propeller deicing system is used only when the propellers are rotating and for short test periods of time during the takeoff check list or system inspection. Propeller Synchronization and Synchrophasing Most multi-engine aircraft are equipped with propeller synchronization systems. Synchronization systems provide a means of controlling and synchronizing engine rpm. Synchronization reduces vibration and eliminates the unpleasant beat produced by unsynchronized propeller operation. A typical synchrophasing system is an electronic system as shown in figure 1-35. It functions to match the rpm of both engines and establish a blade phase relationship between the left and right propellers to reduce cabin noise. The system is controlled by a two-position switch located forward of the throttle quadrant. Turning the control switch on supplies direct current (DC) power to the electronic control box. Input signals representing propeller rpm are received from magnetic pickup on each propeller. The computed input signals are corrected to a command signal and sent to an rpm trimming coil located on the propeller governor of the slow engine. Its rpm is adjusted to that of the other propeller. Figure 1-35. Synchrophasing system. Issue No. 0 ET-PP03.1 Page 36 of 42 Ethiopian Aviation Academy Aviation Maintenance Revision No. 0 Training March 2020 ET-PP03 M01: Propeller Fundamentals Auto feathering System An auto feather system is used normally only during takeoff, approach, and landing. It is used to feather the propeller automatically if power is lost from either engine. The system uses a solenoid valve to dump oil pressure from the propeller cylinder (this allows the prop to feather) if two torque switches sense low torque from the engine. This system has a test-off-arm switch that is used to arm the system. Regulation Pertaining to Propellers To understand the guidelines set down by the FAA regarding propeller system designs and maintenance. It ls necessary to understand some of the regulations concerning propellers. This will be accomplished by looking at parts of the following Federal Aviation Regulations: FAR Part 23, Airworthiness Standards: Normal, Utility, and Acrobatic Aircraft, and FAR Part 25, Airworthiness Standards: Transport Category Aircraft, outline the requirements for propellers and their control systems for aircraft certification. Because there is very little difference in the wording of Parts 23 and 25, they are considered identical for the purposes of this discussion. Part 43 of the FAR defines the different classes of maintenance for the propeller system and the minimum requirements for 100 hour and annual inspection. The Information that is required to be permanently affixed to a propeller is discussed briefly with reference to FAR Part 45, Identification and Registration Markings. The licenses required to perform or supervise the maintenance or repair of a propeller and related systems are covered by FAR Part 65, Certificat1on: Airmen Other Than f1lght Crewmembers. This section distinguishes between the authority of a Powerplant mechanic, a propeller repairman. and an authorized inspector. Subparts of each FAR have been arranged for ease of presentation and do not follow the order as written in the FARs. Propeller Requirements for Aircraft Certification Aircraft propellers must be certificated under FAR Part 35 and must contain the folloW1ng Information on the hub or butt of the propeller blade: builder’s name. model designation. serial number, type certificate number, and production certificate number {FAR 43.13}. Static RPM An aircraft which uses a fixed pitch propeller will not operate at maximum RPM (tachometer reading) on the ground in no wind condition when the engine ls producing maximum allowable horsepower. This is designed into the system to satisfy the requirement that the propeller must limit engine RPM to the maximum allowable when the engine is operating at full power and the aircraft is flying at its best rate-of-climb speed, thus preventing engine damage due to over speeding. The propeller must also prevent the engines from exceeding the ratted RPM by no more than Issue No. 0 ET-PP03.1 Page 37 of 42 Ethiopian Aviation Academy Aviation Maintenance Revision No. 0 Training March 2020 ET-PP03 M01: Propeller Fundamentals 10% in a closed throttle dive at the aircraft's never-exceed speed (FAR 23/25.33). As airspeed or wind speed increases, engine RPM will increase because it Is easier for the propeller to rotate. This explains why the listed static RPM (RPM at full power) on the ground, with no wind) for an aircraft is less than the engine rated RPM (tachometer redline). All two position and controllable-pitch propellers must comply with FAR 23/25.33 at their low blade angle setting. A constant speed propeller system must limit engine speed to rated RPM at all times when the system is operating normally. If the governor should fail, the system must be designed to prevent a static RPM of no more that 103% of rated RPM [FAR 23/25.33). This is accomplished by using the correct low blade angle setting, the greater the blade angle, the lower the static RPM. Cockpit Controls And Instruments Propeller control levers in the cockpit must be arranged to allow Easy operation of all controls at the same time, but not to restrict the movement of individual controls (FAR 23/25.1149). The propeller controls must be Jigged so that an increase in RPM is achieved by moving the controls forward and a decrease in RPM ls caused by moving the controls aft. The throttles must be arranged so that forward thrust is increased used by forward movement of the control and reverse thrust is increased by aft movement of the throttle. (FAR 23/25.779). (When operating in reverse, the throttles are used to place the propeller blades at a negative angle.) Cockpit Powerplant controls must be arranged to prevent confusion as to which engine they control. Recent regulation changes require that control knobs be distinguished by shape and color (FAR 23/25.781) as shown in Figure 1-36. Cockpit instruments such as tachometers and manifold pressure gauges must be marked with a green arc to indicate the normal operating range, a yellow arc for takeoff and precautionary range, a red are for critical vibration range and a red radial line for maximum operating limit (FAR 23/25.1549). Figure 1-36. Cockpit controls shape and color Issue No. 0 ET-PP03.1 Page 38 of 42 Ethiopian Aviation Academy Aviation Maintenance Revision No. 0 Training March 2020 ET-PP03 M01: Propeller Fundamentals Minimum Terrain and Structural Clearances The minimum ground clearance (distance from the level ground to the edge of the propeller disc for a tailwheel aircraft in the takeoff attitude is nine inches. For a tricycle geared aircraft. in the most nose low nominal attitude (stationary, taxi, or takeoff attitude) the minimum ground clearance is seven inches. These clearances are based on normal tire and strut inflation. If the tire and strut are deflated there need be only a positive ground clearance (the propeller disc must not touch the ground). For a seaplane there must be a minimum of 18 inches clearance between the water and the propeller disc. All aircraft must be designed so that the edge of the propeller disc does not come any closer than one inch to the airframe. This is known as radial clearance. The propeller must be positioned at least one-half inch in front of, or behind any part of the airframe, other than in the area of the spinner and cowling where only a positive clearance is required. FAR 23/25.925 gives full details of the propeller clearance requirements. Figure 1-37. Propeller ground and structural Clearances Issue No. 0 ET-PP03.1 Page 39 of 42 Ethiopian Aviation Academy Aviation Maintenance Revision No. 0 Training March 2020 ET-PP03 M01: Propeller Fundamentals Feathering System Requirements If a propeller can be feathered. there must be some means of unfeathering it in flight (FAR 23/25.1153). If a propeller system uses oil to feather the propeller, a supply of oil must be reserved for feathering use only. A provision must be made in this system to prevent sludge or foreign matter from affecting the feathering oil supply (FAR 23/25.1027). These requirements are normally met by using a standpipe in the engine oil tank with an outlet only to the propeller feathering system. A separate feathering control is required for each propeller and must be configured to prevent accidental operation (FAR 23/25.1153). This may be done by the use of a separate feathering control such as a feathering button or by requiring an extreme movement of the propeller control. Propeller Maintenance Regulations Autbodzed Maintenance Personnel The inspection adjustment, installation and minor repair of a propeller and its related parts and appliances on the engine are the responsibility of the Powerplant mechanic. The Powerplant mechanic may also perform the 100-hour inspection of the propeller and related components (FAR 65.87). A propeller repairman may perform or supervise the major overhaul and repair of propellers and related parts and appliances for which he is certificated. The repair and overhaul must be performed in connection with the operation of a certified repair station, commercial operator or air carrier (EAR 65. l 03). An A&P mechanic who holds an Inspection Authorization may perform the annual inspection of a propeller. but he may not approve major repairs and alterations to propellers and related parts and appliances for return to service. Only an appropriately rated facility such as a propeller air station. may return a propeller or accessory to service after a major repair or alteration (FAR 65.81 and 65.91). Preventive Maintenance The following are types of preventive maintenance that may be associated with propellers and their systems: repairing defective safety wiring or cotter keys; lubrication not requiring disassembly other than removal of nonstructural Hems such as cover plates, cowlings, and fairings; applying preservative or protective material (paint. wax, etc.) to components when no disassembly its required and the coat is not prohibited or contrary to good practice {FAR Part 43, Appendix A(c)}. Major Alterations And Repairs The following are major propeller alterations when not authorized in the F'AA propeller specifications: a change to the blade or hub design; a change in the governor or control Issue No. 0 ET-PP03.1 Page 40 of 42 Ethiopian Aviation Academy Aviation Maintenance Revision No. 0 Training March 2020 ET-PP03 M01: Propeller Fundamentals design; installation of a governor or feathering system; installation of a propeller de-icing system; installation of parts not approved for the propeller. Propeller major repairs are classified as any repair to. or straightening of steel blades; repairing or machining of steel hubs; shortening of blades; retipplng of wood propellers; replacement of outer laminations on fixed-pitch wood propellers; repairing elongated bolt holes in the hub of fixed pitch wood propeller; inlay work on wood blades: repairs to composition blades; replacement of tip fabric; replacement of plastic covering; repair of propeller governors; overhaul of controllable-pitch propellers; repairs to deep dents. cuts, scars, nicks, etc. and straightening of aluminum blades; the repair or replacement of Internal blade elements {FAR Part 43 Appendix A(a) (3) and {b}(3)}. Major repairs and alterations to propellers and control devices are normally performed by the manufacturer or a certified repair station. When a propeller or control device is overhauled by a repair facility, a maintenance release tag will be attached to the Item to certify that the item ls approved for return to service. This tag takes the place of a FAA Form 337 and should be attached to the appropriate logbook (FAR Part 43 Appendix B(b)). Annual And 100-Hour lnspection When performing a 100 hour or annual Inspection, Appendix 0 of FAR 43 specifies that the following areas related to propellers and their controls must be inspected: engine controls for defects, improper travel and improper safety; lines, hoses, and clamps for leaks, improper condition and looseness; accessories for apparent defects in security of mounting: all systems for improper installation. poor general condition, defects, and insecure attachment; propeller assembly for cracks, nicks, binds, and oil leakage; bolts for improper torqueing and lack of saftyfng, anti-icing and de-icing devices for improper operation and obvious defects, control mechanisms for improper operation insecure mounting, and restricted travel. These inspections are the minimum required by regulation. Always refer to the manufacturer's manuals for specific inspection procedures. Issue No. 0 ET-PP03.1 Page 41 of 42