Aviation Technical Training Module 4: Propeller Systems PDF
Document Details
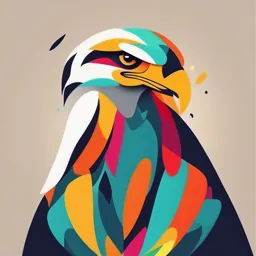
Uploaded by EnterprisingMagnolia
Tags
Summary
This document is a student resource on propeller systems, specifically propeller aerodynamics. It covers topics like airflow characteristics, forces acting on a propeller, and different components, such as the hub, spinner, and blades. The document also includes a table of contents, learning outcomes and assessment criterion, and practice questions.
Full Transcript
Aviation Technical Training Air Force Aircraft Technician (TMP No. 213409) Student Resource Module 4: Propeller Systems Topic 2: Propeller Aerodynamics This document is FOR TRAINING PURPOSES ONLY and is not subject to amendment. For current information, the reader should consult the appr...
Aviation Technical Training Air Force Aircraft Technician (TMP No. 213409) Student Resource Module 4: Propeller Systems Topic 2: Propeller Aerodynamics This document is FOR TRAINING PURPOSES ONLY and is not subject to amendment. For current information, the reader should consult the appropriate Defence Instruction or Technical Manual. This document is not to be used as an authority in the working environment. © BAE Systems. This work is copyright. Apart from any use as permitted under the Copyright Act 1968 and under Contract No. AFTG 01/13, no part may be reproduced by any process without prior written permission from BAE Systems. Aviation Technical 213409-SR-04.02_RevE Page: 2 Template Version No: 1.4 Training © BAE Systems Document Updated: 28/04/20 Table of Contents LEARNING OUTCOMES AND ASSESSMENT CRITERIA........................................................................ 5 AIRFLOW CHARACTERISTICS..................................................................................................... 6 LIFT.......................................................................................................................................... 6 DRAG........................................................................................................................................ 6 THRUST..................................................................................................................................... 7 SLIP.......................................................................................................................................... 7 FORCES ACTING ON A PROPELLER.............................................................................................. 8 CENTRIFUGAL FORCE.................................................................................................................... 8 CENTRIFUGAL TWISTING MOMENT.................................................................................................. 9 AERODYNAMIC TWISTING MOMENT.............................................................................................. 11 TORQUE BENDING FORCE............................................................................................................ 12 THRUST BENDING FORCE............................................................................................................. 13 FORCE ACCENTUATION/COUPLING...........................................................................................14 PROPELLER ROTATIONAL VELOCITY AND AIRCRAFT VELOCITY...........................................................15 INCREASED ROTATIONAL VELOCITY................................................................................................ 16 INCREASED FORWARD VELOCITY................................................................................................... 17 TOTAL REACTION....................................................................................................................... 18 TOTAL REACTION FORCE.............................................................................................................. 19 PROPELLER COMPONENTS......................................................................................................20 HUB....................................................................................................................................... 20 SPINNER.................................................................................................................................. 21 BLADES................................................................................................................................... 21 PROPELLER BLADE CONSTRUCTION...........................................................................................23 BLADE ZONES........................................................................................................................... 23 ROOT AND BUTT........................................................................................................................ 23 SHANK.................................................................................................................................... 23 TIP......................................................................................................................................... 23 CAMBER FACE........................................................................................................................... 24 THRUST FACE............................................................................................................................ 24 LEADING EDGE.......................................................................................................................... 24 Aviation Technical 213409-SR-04.02_RevE Page: 3 Template Version No: 1.4 Training © BAE Systems Document Updated: 28/04/20 TRAILING EDGE......................................................................................................................... 24 CHORD LINE............................................................................................................................. 24 BLADE REFERENCING SYSTEM...................................................................................................... 25 CUFF...................................................................................................................................... 26 TAPER BORE............................................................................................................................. 26 BLADE MANUFACTURE............................................................................................................... 27 PROPELLER SURFACE PROTECTION............................................................................................30 ANODISING.............................................................................................................................. 30 CADMIUM PLATING................................................................................................................... 30 SHOT PEENING.......................................................................................................................... 30 ENGINE POWER AND PROPELLER DESIGN....................................................................................31 POWER REQUIREMENTS.............................................................................................................. 32 AIRCRAFT STABILITY AND CONTROL...........................................................................................34 PROPELLER TORQUE REACTION..................................................................................................... 34 PROPELLER SLIPSTREAM.............................................................................................................. 35 PROPELLER GYROSCOPIC EFFECT................................................................................................... 35 ENGINE CONFIGURATIONS........................................................................................................... 36 SELF-CHECK YOUR TOPIC KNOWLEDGE.......................................................................................37 Aviation Technical 213409-SR-04.02_RevE Page: 4 Template Version No: 1.4 Training © BAE Systems Document Updated: 28/04/20 Learning Outcomes and Assessment Criteria Module learning outcomes 4.1 Describe propeller system layout and operation. Assessment criteria 4.1.3. Describe the characteristics of propeller airflow. 4.1.4. Describe forces acting on a propeller. 4.1.5. Describe the aerodynamic factors of propeller rotational velocity and aircraft velocity. 4.1.6. Identify the major components used in the construction of a propeller. 4.1.7. Describe the construction of propeller blades. 4.1.8. Describe the relationship between engine power and propeller design. 4.1.9. Describe the factors that affect aircraft stability and control. Aviation Technical 213409-SR-04.02_RevE Page: 5 Template Version No: 1.4 Training © BAE Systems Document Updated: 28/04/20 Airflow Characteristics The aerofoil shape of a propeller is designed to increase airflow velocity over the blade’s camber surface. Lift Lift is the aerodynamic force created by air flowing over an aerofoil and is shown in Figure 1. Figure 1: Lift The increase in airflow decreases pressure above the aerofoil. The pressure differential above and below the aerofoil produces a force upward and is termed lift. Drag Drag is caused by the disruption or impact of airflow over, or onto, an aerofoil. Drag is the force that opposes thrust and is shown in Figure 2. Figure 2: Drag Aviation Technical 213409-SR-04.02_RevE Page: 6 Template Version No: 1.4 Training © BAE Systems Document Updated: 28/04/20 Thrust Thrust is a forward acting force and is the reaction to the mass of air being accelerated rearwards from the propeller, as shown in Figure 3. Thrust is felt on the propeller thrust face. Figure 3: Thrust Slip Slip is defined as the difference between geometric pitch and effective pitch as shown in Figure 4. Slip can also be said to be the loss of efficiency, due mainly to air compressibility. Slip = geometric pitch minus effective pitch Figure 4: Slip Geometric pitch Geometric pitch is a calculated distance that a propeller would advance forward through a solid medium in one revolution at a given angle. Slip is the differnece between the two is slip Effective pitch Effective pitch is the distance that a propeller actually does advance forward in one revolution at a given angle. Aviation Technical 213409-SR-04.02_RevE Page: 7 Template Version No: 1.4 Training © BAE Systems Document Updated: 28/04/20 Forces Acting on a Propeller The operation of a propeller during flight depends on a number of factors. Dynamic and aerodynamic forces either combine or work in opposition to create the resultant lift and thrust at each blade. The following forces are generated when a propeller is rotating and producing thrust:  centrifugal force  centrifugal twisting moment (CTM)  aerodynamic twisting moment (ATM)  torque bending force  thrust bending force. Centrifugal force Centrifugal force is a physical force that tends to throw the rotating blades away from the hub, as shown in Figure 5. It is proportional to propeller RPM, i.e. the higher the RPM, the greater the centrifugal force acting on the blades. Figure 5: Centrifugal Force Aviation Technical 213409-SR-04.02_RevE Page: 8 Template Version No: 1.4 Training © BAE Systems Document Updated: 28/04/20 Centrifugal twisting moment Centrifugal twisting moment (CTM) is a force that tends to rotate propeller blades toward a fine blade angle, as shown in Figure 6. Figure 6: Centrifugal Twisting Moment CTM acting on propeller blades produces tensile stress at the blade shank and root, and a torque about the pitch change axis, as shown in Figure 7. CTM is a force that propeller manufacturers utilise on variable pitch propellers, to alter the blade angle from a coarser to a finer angle. Aviation Technical 213409-SR-04.02_RevE Page: 9 Template Version No: 1.4 Training © BAE Systems Document Updated: 28/04/20 Figure 7: Centrifugal Twisting Moment Aviation Technical 213409-SR-04.02_RevE Page: 10 Template Version No: 1.4 Training © BAE Systems Document Updated: 28/04/20 ATM = Course CTM = Fine Aerodynamic twisting moment Aerodynamic twisting moment (ATM) generates forces that tend to move the propeller blades to a coarser blade angle. ATM works against CTM. CTM is approximately 20 times as great as ATM. Figure 8 illustrates that the centre of pressure is forward of the rotational axis of the blade. The rotational axis is the mid-point of the chord line. ATM generates forces that tend to increase the blade angle and is used to aid in feathering the propeller. Figure 8: Aerodynamic Twisting Moment Aviation Technical 213409-SR-04.02_RevE Page: 11 Template Version No: 1.4 Training © BAE Systems Document Updated: 28/04/20 Torque bending force Torque bending force is a resultant force that is generated from air resistance (drag) opposing propeller rotation due to engine torque. Torque bending force bends the propeller blades opposite to the direction of rotation, as shown in Figure 9. Figure 9: Torque Bending Force Aviation Technical 213409-SR-04.02_RevE Page: 12 Template Version No: 1.4 Training © BAE Systems Document Updated: 28/04/20 Thrust bending force Thrust bending force causes the blades to bend forward of their plane of rotation as the aircraft is pulled through the air, as shown in Figure 10. Figure 10: Thrust Bending Force Aviation Technical 213409-SR-04.02_RevE Page: 13 Template Version No: 1.4 Training © BAE Systems Document Updated: 28/04/20 Force Accentuation/Coupling Aerodynamic and centrifugal twisting moments increase with engine speed. For example, if the engine speed is doubled, the stresses quadruple. The combination of centrifugal force and thrust create forces that cause severe stress on the propeller. These forces are at their greatest near the hub. The propeller blade is exposed to:  tension from centrifugal force  torsion from CTM  tension and compression from blade bending. The propeller is designed to withstand these stresses; however, a simple scratch or dent in the blade can have severe repercussions. Scratches or dents can develop into stress concentrators. Stress will concentrate in these areas and cause a rapid deterioration in the propeller. Aviation Technical 213409-SR-04.02_RevE Page: 14 Template Version No: 1.4 Training © BAE Systems Document Updated: 28/04/20 Propeller Rotational Velocity and Aircraft Velocity The performance (thrust) of a fixed pitch propeller will vary with changes in either propeller rotational velocity or aircraft velocity. In this example we will consider the fixed pitch propeller with a blade angle of 20°. This propeller, when stationary, at 1500 rpm and no forward velocity will have an angle of attack of 20° as the relative airflow is directly opposite the movement of the propeller. When aircraft forward speed is increased to 50 MPH, whilst maintaining the engine speed at 1500 RPM, the correct angle of attack (two to four degrees) produced. This is the forward velocity and rotational speed it is designed to operate at as shown in Figure 11. The propeller will produce the required amount of thrust until either rotational velocity or forward velocity alters, as shown in Figure 12 and Figure 13. Figure 11: Effects on Angle of Attack Aviation Technical 213409-SR-04.02_RevE Page: 15 Template Version No: 1.4 Training © BAE Systems Document Updated: 28/04/20 Going into climb Increased rotational velocity When forward velocity is maintained and rotational velocity is increased to 2000 RPM, the angle of attack will become larger and inefficient. Excessive angles of attack are ineffective because of the possibility of blade stall. Figure 12 compares an increase in rotational velocity to the efficient operation of a propeller blade. Figure 12: Increased Rotational Velocity Aviation Technical 213409-SR-04.02_RevE Page: 16 Template Version No: 1.4 Training © BAE Systems Document Updated: 28/04/20 Going into dive Increased forward velocity When aircraft forward velocity increases and the propeller rotational velocity is maintained, the blade path will move from being behind the chord line, a positive angle of attack, to being in front of the chord line, a negative angle of attack, as shown in Figure 13. Figure 13: Increased Forward Velocity Increased forward velocity can result in the angle of attack moving from a positive to a negative value, which produces little forward thrust. Thrust could then be produced in the opposite direction and have a windmilling or braking effect on the propeller. The management of this phenomenon is described later in this module. Aviation Technical 213409-SR-04.02_RevE Page: 17 Template Version No: 1.4 Training © BAE Systems Document Updated: 28/04/20 Total reaction Total reaction of a blade is the resultant of a combination of the following vector forces:  lift and drag  thrust and torque. Total reaction is calculated in newtons (a unit of force) by measuring the diagonal line that joins the above forces. Lift and drag Figure 14 represents a vector diagram with a resultant reaction force for lift and drag. Figure 14: Resultant Reaction – Lift and Drag Thrust and torque Figure 15 represents a vector diagram with a resultant reaction force for thrust and torque. Figure 15: Resultant Reaction – Thrust and Torque Aviation Technical 213409-SR-04.02_RevE Page: 18 Template Version No: 1.4 Training © BAE Systems Document Updated: 28/04/20 Total reaction force The total reaction of all forces is determined by combining vector diagrams Figure 14 and Figure 15, and is shown as the total reaction force in Figure 16. Figure 16: Total Reaction – Lift and Drag plus Thrust and Torque Aviation Technical 213409-SR-04.02_RevE Page: 19 Template Version No: 1.4 Training © BAE Systems Document Updated: 28/04/20 Propeller Components Hub A propeller hub assembly is typically a barrel-shaped metal housing that mounts and secures the blade root in a multi-bladed propeller assembly. The hub houses the spider, which enables fitment of the blade within the hub, and transmits engine torque to the propeller. It also provides a means of attaching the propeller to the engine. Larger hub assemblies are usually divided into forward and rear halves that are matched together for life. The rear half provides a means of attaching the propeller onto the engine propeller shaft and transmits engine torque, while the front barrel half provides mounting for dome and spinner assemblies. An example of a two-bladed counterweight propeller hub assembly is shown in Figure 17. Figure 17: Hub Assembly Aviation Technical 213409-SR-04.02_RevE Page: 20 Template Version No: 1.4 Training © BAE Systems Document Updated: 28/04/20 Spinner A spinner is an aerodynamic fairing fitted to a propeller assembly to enclose the hub and pitch change assemblies. An example of a propeller spinner is shown in Figure 18. Figure 18: Spinner Assembly Blades Blade assemblies create the desired thrust by moving air rearward. A light aircraft propeller may be a fixed pitch, solid metal structure, as shown in Figure 19, or a more complex multi- bladed structure, as shown in Figure 20. Figure 19: Fixed Pitch Blades Aviation Technical 213409-SR-04.02_RevE Page: 21 Template Version No: 1.4 Training © BAE Systems Document Updated: 28/04/20 Figure 20: Multi-bladed Propeller Aviation Technical 213409-SR-04.02_RevE Page: 22 Template Version No: 1.4 Training © BAE Systems Document Updated: 28/04/20 Propeller Blade Construction Blade Zones A propeller blade is typically identified by zones. Figure 21 shows the basic zones of a blade. Root and butt The blade root is round and forms part of the propeller blade that fits into the propeller hub. It consists of the blade butt, thrust shoulders, thrust ring, and the inboard end of the shank. The blade root and butt are shown in Figure 21. Shank The blade shank is the cylindrical part of the blade located near the blade root, as shown in Figure 21. The blade shank is thickened for strength. Tip The blade tip is typically defined as the last six inches of the blade and is the portion of the blade that is at the opposite end to the hub assembly, as shown in Figure 21. Figure 21: Propeller Blade Aviation Technical 213409-SR-04.02_RevE Page: 23 Template Version No: 1.4 Training © BAE Systems Document Updated: 28/04/20 Camber face The blade camber face is the forward facing convex side of the propeller blade aerofoil. The camber face joins the leading and trailing edges, as shown in Figure 22. Thrust face The blade thrust face, shown in Figure 22, is the flat side of a propeller blade and is the face that produces the thrust. Figure 22: Blade Thrust and Camber Face Leading edge The leading edge of a blade is the thick edge of the blade that first meets the air as the propeller rotates, as shown in Figure 23. Trailing edge The trailing edge is the tapered edge of the blade opposite to the leading edge. It is the point where the blade camber face and thrust face join, as shown in Figure 23. Figure 23: Blade Leading and Trailing Edges Chord line The chord line is an imaginary line drawn through the centre of the leading and trailing edges, as shown in Figure 24. The chord line assists in determining propeller blade angles. Figure 24: Blade Chord Line Aviation Technical 213409-SR-04.02_RevE Page: 24 Template Version No: 1.4 Training © BAE Systems Document Updated: 28/04/20 Figure 25 shows a summary of the terms relating to a propeller blade surface. Figure 25: Blade Terms – Summary Blade Referencing System Propeller blade referencing system, similar to aircraft referencing stations, assists in identifying a blade area, and enable correct assessment of blade damage. The blade stations are at a designated distance of six inches and are measured from the centre of the hub as shown in Figure 26 below. Figure 26: Blade referencing Aviation Technical 213409-SR-04.02_RevE Page: 25 Template Version No: 1.4 Training © BAE Systems Document Updated: 28/04/20 Cuff Propeller blade cuffs are designed to restore the round section of the blade shank to an aerofoil shape. Blade cuffs increase airflow to the engine and can be constructed from metal, foam or plastic. Figure 27 shows foam cuffs bonded to the blade shanks. Figure 27: Blade Cuff Taper bore The taper bore is used to house an interchangeable bush. The bush provides an accurate machined surface for the blades to rotate on when blade angle is changed. The taper bore also houses a balance plug, which is used when balancing the blade and propeller assembly. Figure 28 shows the taper bore and balance plug components. Figure 28: Taper Bore and Balance Plug Aviation Technical 213409-SR-04.02_RevE Page: 26 Template Version No: 1.4 Training © BAE Systems Document Updated: 28/04/20 Blade Manufacture The blade assembly is the aerofoil part of the propeller. The blade converts the torque of the engine into thrust. Most propeller blades are made of one of the following materials:  metal  composite. Metal Metal propellers start out as a single bar of aluminium alloy. These bars are then shaped and finished to the desired aerofoil shape by machine-forging, copying the shape of a master blade (sometimes referred to as a profile) onto the bar of aluminium. Metal propellers incorporate a centre bore for the installation of steel hubs or adaptors. The installation of hubs and adaptors enables the propeller to be fitted to different installations. Composite Composite blade construction involves the use of special plastic resins that are reinforced with fibres, or filaments composed of one of the following:  glass  carbon  Kevlar®  Boron. Composite blades can be manufactured by forming a composite:  aluminium spar  shell form. Aviation Technical 213409-SR-04.02_RevE Page: 27 Template Version No: 1.4 Training © BAE Systems Document Updated: 28/04/20 Composite aluminium spar The composite aluminium spar uses any of the resins, fibres or filaments from the materials listed above. These are then shaped around an aluminium alloy spar and have a foam core to strengthen the blade against distortion, as shown in Figure 29. Figure 29: Composite Aluminium Spar Blade Composite shell form Figure 30 shows a composite material shell used to form the blade profile. A foam core is placed into the shell to provide resistance to distortion. Figure 30: Composite Shell Form Aviation Technical 213409-SR-04.02_RevE Page: 28 Template Version No: 1.4 Training © BAE Systems Document Updated: 28/04/20 Kevlar® – fibre reinforced plastic (FRP) moulding The FRP moulding is a variation of the composite blade. The FRP blade consists of a laminated Kevlar® shell into which is placed a foam core. Kevlar® is layered lengthwise and crosswise to boost the strength of the shell. The leading and trailing edges of the blade are reinforced with solid unidirectional Kevlar®. Two unidirectional Kevlar® shear webs are placed between the camber and the thrust face surfaces of the shell to provide resistance to flexing and buckling. The polyurethane foam filling supplies additional resistance to any distortion caused by operating stresses that the propeller encounters. Figure 31 illustrates the construction of a blade using this process. Figure 31: Fibre Reinforced Plastic (FRP) Moulding Aviation Technical 213409-SR-04.02_RevE Page: 29 Template Version No: 1.4 Training © BAE Systems Document Updated: 28/04/20 Propeller Surface Protection Surface protection of metal propeller blades is provided to protect the blades against corrosion, erosion and damage. Surface protection methods include:  anodising  cadmium plating  shot peening. Anodising Anodising is an electroplating process used to produce a hard coating on an aluminium surface. The hard coating is corrosion-resistant, waterproof and airtight. Cadmium plating Cadmium plating is also an electroplating process where a thin coating is applied to steel components as a form of sacrificial corrosion protection. Shot peening Shot peening of metals is designed to distribute stresses evenly around the blade shank. Shot peening also increases fatigue strength. The shaded area in Figure 32 shows the area of an aluminium propeller that is shot-peened. Figure 32: Shot Peening Specially-designed beads are thrown by centrifugal force, or projected by compressed air through a nozzle. The beads may be glass or steel. The impact of the shot causes plastic deformation of the surface to a depth of a few thousandths of an inch. Aviation Technical 213409-SR-04.02_RevE Page: 30 Template Version No: 1.4 Training © BAE Systems Document Updated: 28/04/20 Engine Power and Propeller Design A propeller must be capable of efficiently converting engine power into effective thrust. Many factors contribute to the selection of an appropriate propeller assembly for a particular aircraft. Factors such as aircraft and engine design, the nature of aircraft operation, conditions of flight, take-off, landing roll and aircraft performance requirements are all considered. A constant speed automatic propeller would be appropriate for a multi-engine aircraft required to fly long distances with reasonable economy. This propeller would adjust blade angle to meet engine power and aircraft speed values selected by the pilot. Feather capability would also be appropriate for this example. By contrast, a less expensive counterweight propeller would be appropriate for a small aircraft performing short local flights. Larger propellers were manufactured in the early years of propeller design as engine power increased. This enabled engine power to be absorbed by the propeller; however, it created efficiency problems. Blade tip vibration and flutter developed at high rotational speeds as blade tips approached the speed of sound. Designers then considered reduction gearing to reduce propeller speed. More blades were added to the assembly to utilise the power more effectively. Wide section blades are also more capable of absorbing engine power because they can do more work on the air. This enables the propeller to absorb increased torque from engines that power propellers through reduction gearboxes. Contra-rotating propellers are another means of effectively utilising engine power because the overall diameter of each propeller is reduced per engine. To absorb the large power capabilities of engines, larger propellers were designed and produced. However, the increase in propeller diameter did not increase efficiency. In fact, larger propellers lost performance through tip flutter and turbulence caused by shock waves (compressibility effects) as the tip speed of the propeller approaches the speed of sound, which is approximately 1116.4 feet per second (or 660 knots) at sea level on a standard day of 15°C. The following design features can be incorporated to reduce blade flutter and absorb engine power:  reducing propeller tip speed through engine reduction gearing  reducing propeller diameter and increasing the number of blades  altering blade shape and section  using scimitar or gull wing-bladed propellers. Aviation Technical 213409-SR-04.02_RevE Page: 31 Template Version No: 1.4 Training © BAE Systems Document Updated: 28/04/20 Power requirements The propeller must be able to absorb the power of the engine. If this does not occur the propeller will speed up, resulting in the engine and the propeller becoming inefficient. The following four factors are considered when selecting a propeller for an engine with a known power output:  propeller diameter  number of blades on the propeller  propeller blade shape and section  propeller mass or solidity. Propeller diameter As more powerful engines were utilised, the propeller diameter was increased to absorb the extra power. The diameter of propellers had to be limited due to the tips reaching the speed of sound, causing turbulence. This limitation was overcome by using either contra-rotating propellers or increasing the number of blades fitted to the propeller. Fitting of contra- rotating propellers to an engine is in effect putting two propellers onto the one engine, thereby allowing the diameter of the propeller to be reduced. Scimitar or gull wing bladed propellers alleviate blade tip problems by raising the critical Mach number (Mcrit) of the propeller blade tips. Number of blades An increase in the number of blades was used to reduce the overall diameter of a propeller. Increasing the number of blades allowed engine power to be utilised without increasing the propeller diameter. Increasing the number of blades is the most efficient method of reducing turbulence and absorbing engine power. Figure 33 illustrates an aircraft with a five- bladed propeller. Figure 33: Multi-blade Propellers Aviation Technical 213409-SR-04.02_RevE Page: 32 Template Version No: 1.4 Training © BAE Systems Document Updated: 28/04/20 Blade shape and section Altering the blade shape or camber of the propeller blade is another method used to absorb engine power. However, if camber is increased to produce extra lift/thrust, then drag is proportional. A compromise is made in the propeller shape and size to achieve a balance. Adding to the increased drag is the extra weight of each propeller. Any advantage gained from any lift/thrust is lost by drag and the increase in weight. Figure 34 illustrates a blade with an increase in camber, and the proportional increase in size and weight. Figure 34: Blade Shape and Section Propeller solidity The propeller solidity is the ratio between the part of the propeller disc at the ¾ radius (viewed from the front) to that which is solid, as shown in Figure 35. The propeller area may be 10% of the total area of the disc, therefore its solidity would be a ratio of 1:10. The propeller blades beyond the ¾ radius are not included in the total of solidity as these areas are deemed to be inefficient due to air compressibility effects. Solidity can be increased by the number of blades or increasing the chord of the blades. Increasing propeller solidity can absorb greater engine power, however increasing the blade chord increases CTM and therefore strain on the shanks, hubs and pitch changing mechanisms. Figure 35: Propeller Solidity Aviation Technical 213409-SR-04.02_RevE Page: 33 Template Version No: 1.4 Training © BAE Systems Document Updated: 28/04/20 Aircraft Stability and Control It is imperative to maintain aircraft stability and control. The following factors have an effect on aircraft stability and control:  propeller torque reaction  propeller slipstream  propeller gyroscopic effect  engine configurations. Propeller torque reaction Torque reaction is the force generated by a rotating propeller that is transferred to the aircraft structure via the powerplant. As the propeller is being rotated, the torque being developed to drive the propeller tends to rotate the aircraft in the opposite direction, as shown in Figure 36. Torque effect is greatest in single engine aircraft at low airspeeds with high power settings. Figure 36: Propeller Torque Reaction Aviation Technical 213409-SR-04.02_RevE Page: 34 Template Version No: 1.4 Training © BAE Systems Document Updated: 28/04/20 Propeller slipstream A rotating propeller imparts a rotational motion to the slipstream. The spiralling airflow affects the aircraft’s vertical stabiliser and rudder. The impact increases with higher power settings. Figure 37 shows how the spiralling airflow moves rearward and impacts the left surfaces of the vertical stabiliser and rudder. This causes the aircraft to yaw left. Rudder deflection must change to counter any change in slipstream over these surfaces; this is particularly evident during take-off with high power and low airspeed. Figure 37: Propeller Slipstream Propeller gyroscopic effect The rotating mass of the propeller may cause a gyroscopic effect. A rotating propeller tends to resist any change in its plane of rotation. The propeller will resist a turn to the left or right in straight and level flight. If a change does take place to straight and level flight, there is a tendency for the plane of rotation to change in a direction at right angles (90 degrees) to where the force was applied. The nose will tend to yaw to the right if the propeller rotates clockwise. Aviation Technical 213409-SR-04.02_RevE Page: 35 Template Version No: 1.4 Training © BAE Systems Document Updated: 28/04/20 Engine configurations The fitment of a contra-rotating propeller, as shown in Figure 38, largely eliminates the effects of propeller slipstream, torque reaction and propeller gyroscopic effect because these forces are cancelled out. Figure 38: Contra-rotating Propeller Depending on engine placement, slipstream effect on multi-engine aircraft configurations can be reduced because the slipstream is directed over the wings with reduced impact on the tail fin, as shown in Figure 39. Figure 39: Multi-engine Aircraft Aviation Technical 213409-SR-04.02_RevE Page: 36 Template Version No: 1.4 Training © BAE Systems Document Updated: 28/04/20 Self-check your Topic Knowledge Activity Take some time to think back on the information you have learnt in this topic. Make a list of the key points. The following questions may have already been asked in class. Write down the answers to as many questions as you can. Then, check back in the notes to see if you are correct. 1. What is meant by the following terms: a) lift b) drag c) thrust d) slip 2. Describe the effects of the following forces acting on a propeller: a) centrifugal force b) centrifugal twisting moment c) aerodynamic twisting moment d) torque bending force e) thrust bending force 3. What is meant by the term ‘force accentuation/coupling’ in relation to propeller operation? 4. Describe the affect that increased propeller rotational velocity has on blade angle of attack. 5. Describe the affect that increased aircraft velocity has on blade angle of attack. 6. Describe the relationship between engine power and propeller design requirements. 7. Describe the following terms and their effects on aircraft stability: a) propeller torque reaction b) propeller slipstream c) propeller gyroscopic effect 8. Define propeller slip with relation to the terms geometric pitch and effective pitch. Aviation Technical 213409-SR-04.02_RevE Page: 37 Template Version No: 1.4 Training © BAE Systems Document Updated: 28/04/20