Essentials Of Geology PDF
Document Details
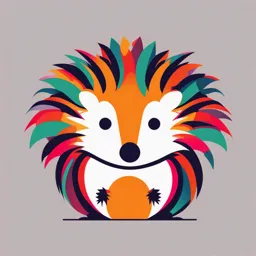
Uploaded by GoodYellow5907
University of Rizal System
Aboy, Remar; Bustamante, John Michael; Capistrano, Crystal Kei; De Juan, Johnrick; Ibarra, Crystal Sheen; Malapitan, Justin; Gacho, John Andrei; Orpilla, James Christian; Sibayan, Shane; Zulueta, Haze
Tags
Summary
This document is a set of notes on Essentials of Geology. The notes outline the attitude of beds, the importance of geological maps, the bearing of folds, faults, and joints, and the physical and mechanical properties of rocks. The document also discusses factors influencing wave velocity in rocks, and includes contributions of several students in the University of Rizal System.
Full Transcript
Republic of the Philippines UNIVERSITY OF RIZAL SYSTEM Antipolo City Engineering Program ESSENTIALS OF GEOLOGY GEOLOGY FOR ENGINEERS (FINALS) BRIEF CONTENTS 1 Attitude of Beds and the Component and Importance of Geological Maps 2 The Bearing of Folds...
Republic of the Philippines UNIVERSITY OF RIZAL SYSTEM Antipolo City Engineering Program ESSENTIALS OF GEOLOGY GEOLOGY FOR ENGINEERS (FINALS) BRIEF CONTENTS 1 Attitude of Beds and the Component and Importance of Geological Maps 2 The Bearing of Folds, Faults, and Joints in Engineering Construction 3 Physical and Mechanical Properties of Rocks 4 Properties of Rocks and the Factors Influencing the Wave Velocity References at the end of each chapter CONTENTS 1 Attitude of Beds and the Component and Importance of Geological Maps 1.1 Bed and Dip 1.2 Geological Maps Definition of Bed and Dip Geological Map Dip (Inclination) Components of Geological Map Importance of Geological Map Classifications Bedding Planes and Beds Principle of Superposition Strike 2 The Bearing of Folds, Faults, and Joints in Engineering Construction 2.1 Faults 2.3 Joints Faults Joints Types of Faults Formation of Joints Types of Strike Slip Faults Types of Joints 2.2 Folds 2.4 Joints, Folds, and Faults Folds Relevance in Engineering Types of Folds Domes and Basins CONTENTS 3 Physical and Mechanical Properties of Rocks 3.1 Physical Properties of Rocks Physical Properties of Rocks Specific gravity Unit weight Moisture Content and Degree of Saturation Permeability and Electrical and Thermal Properties Swelling, Anisotropy, and Durability 3.2 Mechanical Properties of Rock Compressive Strength Tensile Strength Types of Tensile Strength Shear Strength Shear Strength Tests 4 Properties of Rocks and the Factors Influencing the Wave Velocity 4.1 Properties of Rocks 4.3 Factors Influencing Wave Velocity Hardness Wavelength Streaks Frequency Luster Elasticity Color Density Density Temperature Wave type 4.2 Wave Velocity Rock materials Parts of a Wave Depth Poisson's ratio Attitude of Beds and the Components and Importance of Geological Maps Reported by: Aboy, Remar Bustamante, John Michael Capistrano, Crystal Kei De Juan, Johnrick Ibarra, Crystal Sheen Malapitan, Justin Gacho, John Andrei Orpilla, James Christian Sibayan, Shane Zulueta, Hazel Definition of Bed and Dip: (De Juan, Johnrick) What is a Bed? In geological terms, a bed refers to the fundamental unit of stratigraphy, representing a layer of rock or sediment formed through deposition processes. It serves as a base surface for geological activities such as abrasion, plucking, and deposition, often due to the movement of glaciers, water, wind, or other transportation mediums. Each bed is defined by its unique physical properties, like thickness, composition, and texture, which distinguish it from layers above or below. Beds can vary in thickness, with those thinner than 1 cm classified as laminae. These layers are key records of the depositional environment and processes at the time of their formation. Attitude of Bed The attitude of a bed refers to the orientation of a geological layer in three-dimensional space. It is defined by two main parameters: Strike refers to the line formed by the intersection of a horizontal plane and an inclined surface. This line is called a strike line, and the direction the line points in (either direction, as a line points in two opposite directions) is the strike angle. Dip is the angle between that horizontal plane and the inclined surface (such as a geological contact between tilted layers) measured perpendicular to the strike line down to the inclined surface. Strike Dip Dip (Inclination): Represents the angle of inclination of a rock layer from the horizontal. This parameter is crucial in understanding geological structures like folds, faults, and overall stratigraphic orientation. Definition: The angle between the horizontal plane and the tilted surface of the bed. Measurement: Dip is measured in degrees (0° to 90°) relative to the horizontal plane, indicating the bed's steepness. Classification: 0°–20°: Shallow dip 20°–50°: Moderate dip 50°–90°: Steep dip Bedding Planes and Beds: Definition: Bedding planes are the surfaces that separate individual layers or strata in sedimentary rocks. Each layer is called a bed or stratum, the smallest unit of stratigraphy. Formation: Bedding planes signify changes in sediment deposition, often caused by shifts in energy levels or sediment sources. Beds thicker than 1 cm are considered true beds, while thinner layers are called laminae. Varves: These are thin beds deposited in repetitive cycles (e.g., daily or seasonally), valuable for studying past climates. Principle of Superposition: (Capistrano, Crystal Kei) In a series of undisturbed layers, the oldest layer is on the bottom and the youngest on the top when the outcrop lines are parallel, they have the same amount of dip. According to Britannica.com.Principle of Superposition, a major principle of stratigraphy stating that within a sequence of layers of sedimentary rock, the oldest layer is at the base and that the layers are progressively younger with ascending order in the sequence. On occasion, however, deformation may have caused the rocks of the crust to tilt, perhaps to the point of overturning them. It is also said that Danish geologist Nicolaus Steno discovered this principle and outlined in his book De Solido Intra Naturaliter Contento Dissertationis Prodomus. Another use of this principle is that geologists can determine the relative ages of rock strata in order to reconstruct history, and also help geologists determine the age of geologic structures such as mountains and volcanoes, that appear in the middle of existing rock layers. The Law of Superposition can be seen in both vertical and horizontal layers of rock, such as on the Earth's surface. The principle of superposition is crucial for relative dating, allowing geologists to establish a timeline of geological events without needing absolute dates. That is the reason why they found out that earth is much older than we thought. It also helps them to comprehend earth’s history. The Four Types of Dip: 1. True Dip 2. Apparent Dip 3. Primary or Initial Dip 4. Secondary Dip 5. Local or Regional Dip According to Direction (Sibayan, S.) 1. True Dip: The angle between a horizontal plane and the steepest line of descent on a plane (such as a rock layer or fault). This is the maximum angle of inclination and is measured perpendicular to the strike line of the rock layer. The azimuth of the dip is at right angles to the strike of a bed. That means that the true dip always has a 90-degree angle to the strike of a line. Take note that the apparent dip is always less than the true dip and that the angle of the true dip is fixed at 90 degrees from the strike. (Orpilla, James Christian) 2. Apparent Dip: The dip angle measured in any direction other than the true dip direction. This angle is always less than the true dip unless it's measured directly perpendicular to the strike. It can be useful for measuring dip from directions not aligned with the true dip. Since the confusing part is that you also see the true dip from the sides and also the apparent dip is because that's how they supposed to be…you will see their angle from the top view, you will see that the true dip creates a 90° angle while the apparent is less than that, mainly because it will go straight to the other end of it not the same x or y axis of that point…”diagonally”. While a true dip is measured at exactly 90°, an apparent dip is less than 90° which makes it an acute angle which is also a shallow, moderate and steep dip that was discussed up above. (As observed from the image above, apparent dip is a diagonal line that is ranging 1° up until 89°, image show it as it is…a slanted line that is made to section the top point to the opposite end of it on a taken part of the bedline fault) According to Deposition (Malapitan, Justin) 3. Primary or Initial Dip: The initial dip of a rock layer during its formation. This is typically the result of original deposition processes, such as sedimentary layers formed by water, which may have a slight angle due to environmental conditions. Basically, it is the inclination induced in the strata/the layers of rocks due to the original slope to the basin. These dips are less than 35° usually ranging from 0°-20° (Bustamante, John Michael) 4. Secondary Dip: The dip angle that results from tectonic events or structural deformation after the rock layer has formed. Secondary dips are typically caused by folding, faulting, or tilting due to tectonic forces. In simpler terms, the secondary dip can result from a change in the angle of the rock layer due to forces like tectonic activity that alter the layer's orientation after initial deposition. Secondary dip measurements are important for understanding the deformation history and structural features of an area. (Gacho, John Andrei) 5. Local or Regional Dip: The dip of a rock layer observed at a specific location. This dip may vary across a region due to localized structural effects, such as small-scale folds or variations in rock composition that affect the angle of inclination in specific areas. It is the variation of different types of dips like Primary dip which has an inclination of 5-10% and Secondary dip which has an inclination ranging to 90 degrees in a wide area that is being observed. Strike: (Zulueta, Hazel Anne) Strike refers to the line formed by the intersection of a horizontal plane and an inclined surface. It is also measured in degrees relative to the north. This can also be understood as the direction or orientation of a horizontal line that lies on an inclined plane. Using strike and dip, we can figure out the direction and angle of rock layers. The strike shows the direction of a horizontal line on the slanted rock surface, while the dip tells us how steep the surface is and which way it slopes. By looking at these, we can tell which rock layer is younger, based on how the layers are stacked and tilted. Geological Map: (Aboy) A geological map is a specialized type of map that displays the distribution of geological features and materials on or below the Earth's surface. Geological maps are an essential tool in geological research, mineral exploration, and natural resource management, as they provide valuable information about the structure and composition of the Earth's crust. Components of Geological Map: 1. Rock Types Layers of rock. Different colors and symbols 5. Outcrops represent different types of rock and their ages. A legend on the side or The expression of rocks at the bottom of the map explains the surface. names and abbreviations of the rock types. 6. Strike and Dip 2. Faults A symbol that indicates the orientation of rock strata in relation Fractures in the Earth's crust, often to north-south and horizontal. associated with tectonic movements. 7. Cross sections 3. Folds A vertical line drawn across the map Bends in layers of rock, where the that shows the arrangement of rock middle of the fold is a different age layers below the surface. than the outer edges. 8. Topographic lines 4. Beds Show the topography of the land surface. Importance of Geological Map: (Ibarra, Crystal Sheen) Provides a visual representation of the distribution of different rock types, structures, and other geological features across a particular area. Also, used to identify potential hazards such as landslides, earthquakes, and volcanic eruptions. Identifying Natural Resources: Mineral Exploration: Geologic maps help locate valuable minerals like gold, silver, copper, and iron ore. Energy Resource Exploration: They assist in finding oil, natural gas, and geothermal energy sources. Water Resource Assessment: Geologic maps help identify groundwater aquifers and assess their potential for water supply. Assessing Natural Hazards: Earthquake Risk: By identifying fault lines and seismic activity, geologists can assess earthquake risk and develop mitigation strategies. Volcanic Eruptions: Maps help identify volcanic areas and assess the potential for future eruptions. Landslides: Geologic maps can identify areas prone to landslides, helping to prevent property damage and loss of life. Flooding: Understanding the geology of a region can help predict flood risks and inform flood control measures. Land-Use Planning: Infrastructure Development: Geologic maps help identify suitable sites for building roads, bridges, and other infrastructure. Urban Planning: They can help assess the suitability of land for urban development, considering factors like soil stability and groundwater availability. Environmental Protection: Geologic maps can help identify sensitive ecosystems and areas prone to pollution. References Deline, H. (2019, September 10). 12.3: Strike and Dip. Geosciences LibreTexts. Retrieved November 26, 2024, from https://geo.libretexts.org/Learning_Objects/Laboratory/Book%3A_Laboratory_Manual_For_Intro ductory_Geology_(Deline_Harris_and_Tefend)/12%3A_Crustal_Deformation/12.3%3A_Strike_a nd_Dip Geological Maps » Geology Science. (2023, April 23). Geology Science. Retrieved November 26, 2024, from https://geologyscience.com/geology-branches/structural-geology/geological-maps/ Johnson, C., Affolter, M. D., Inkenbrandt, P., & Mosher, C. (2023, November 6). 5.4: Sedimentary Structures. Geosciences LibreTexts. Retrieved November 26, 2024, from https://geo.libretexts.org/Bookshelves/Geology/Book%3A_An_Introduction_to_Geology_(Johns on_Affolter_Inkenbrandt_and_Mosher)/05%3A_Weathering_Erosion_and_Sedimentary_Rocks/ 5.04%3A_Sedimentary_Structures https://viva.pressbooks.pub/physicalgeologylab/chapter/tilted-beds-strike-dip/ https://www.slideshare.net/slideshow/attitude-of-beds/233432092?fbclid=IwZXh0bgNhZW0CMT AAAR0B0gQ8CECCFLwhkX5e3ER7UhiN_VMRBOb1ZrdHcOsSo1wD_YPhjppBu54_aem_yGr SXU0sVf-EgZChNYroVA https://www.scribd.com/document/386727354/true-dip https://www.britannica.com/science/law-of-superposition https://www.vedantu.com/geography/law-of-superposition https://library.fiveable.me/key-terms/introduction-geology/principle-of-superposition THE BEARING OF FOLDS, FAULTS, AND JOINTS IN ENGINEERING CONSTRUCTION FAULTS (Padanan, Melvin) - Is a Plane of Dislocation Where Rocks on One Side of the Fault Have Moved Relative to Rocks on the Other Side. - A Fault Plane Divides a Rock Into Two Blocks. One Block is Referred to as the Hanging Wall, the Other as the Footwall. - Fault is a fracture along which motion has occurred, so that the rocks on either side are offset from each other. TYPES OF FAULTS Dip-Slip Faults - Faults in which movement is primarily parallel to the slope of the fault surface. - Geologists identify the rock surface immediately above the fault as the hanging wall block and the rock surface below as the footwall block. Normal Faults - When the hanging wall block moves down relative to the footwall block, dip-slip faults are classified as normal faults. - Normal faults are associated with tensional stresses that pull rock units apart, thereby lengthening the crust laterally and thinning it vertically. Reverse Faults - Dip-slip faults in which the hanging wall block moves up relative to the footwall block are called reverse faults. - Compressional forces typically push the hanging wall upward relative to the footwall. - Reverse and thrust faults result from compressional stresses that produce horizontal shortening of the crust. Thrust Faults - A thrust fault is a type of reverse fault in which the dip is less than 45 degrees. - In which the dip of the fault plan is so small and almost horizontal. - Reverse and thrust faults result from compressional stresses that produce horizontal shortening of the crust. Strike Slip/Transverse Faults (Colangoy, Curt) - A fault in which the dominant displacement is horizontal and parallel to the trend (direction) of the fault surface - Is typically produced by shear forces when one block slips horizontally past another. TYPES OF STRIKE SLIP FAULTS Right Lateral/Left Lateral Strike-Slip Fault - Because movement along the San Andreas Fault causes the crustal block on the opposite side of the fault to move to the right as you face the fault, it is called a right-lateral strike-slip fault. - The Great Glen Fault in Scotland, which exhibits displacement in the opposite direction, is a well-known example of a left-lateral strike-slip fault. Transform Faults - Transform faults are a unique class of strike-slip faults that slice through Earth’s lithosphere and accommodate motion between two tectonic plates. Most transform faults cut the oceanic lithosphere and link spreading oceanic ridges. FOLDS (Galac, Bill) - Along convergent plate boundaries, rock strata are often bent into a series of wavelike undulations called folds. - Most folds result from compressional stresses that result in a lateral shortening and vertical thickening of the crust. - Folds are also described by their axial plane, which is a surface that connects all the hinge lines of the folded strata. TYPES OF FOLDS (Miñon, Jaydee) Antiforms - Folds that are folds that are concave down, oldest rocks at the hinge zone. down, oldest rocks at the hinge zone. - Anticlines - A specific type of antiform where the oldest rocks are at the center. Synforms - Folds that are concave up, youngest rocks at the hinge. - Synclines - A specific type of synform where the youngest rocks are at the center. Domes and Basins (Galac, Bill) Dome - When a broad upwarping of basement rock deforms the overlying cover of sedimentary strata to produce a circular or slightly elongated bulge, the feature is called a dome. Basins - The inverse of a dome is a downwarped structure termed a basin. - Because structural basins usually contain sedimentary beds sloping at low angles, they are identified by the age of the rocks composing them. JOINTS (Tizon, JRommel) - Among the most common rock structures are fractures called joints. As mentioned earlier, joints differ from faults because no appreciable displacement has occurred along the fracture. Although some joints have a random orientation, most occur in roughly parallel groups. Formation of Joints TENSIONAL STRESS - When rocks are pulled apart, they can fracture. This type of joint is often found in areas where the earth's crust is being stretched, such as in rift valleys. COMPRESSIONAL STRESS - When rocks are squeezed together, they can also fracture. This type of joint is often found in areas where the earth's crust is being compressed, such as in mountain ranges. SHEAR STRESS - When rocks are pushed past each other, they can fracture. This type of joint is often found in areas where the earth's crust is being faulted. TYPES OF JOINTS (De Leon, Aira) Columnar Joints - Form when igneous rocks cool and develop shrinkage fractures that produce elongated, pillarlike columns. Curved Joints - Develop more or less parallel to the surface of large exposed igneous bodies such as batholiths. JOINTS, FOLDS, AND FAULTS RELEVANCE IN ENGINEERING (Lagos, Gian) JOINTS ROCK STABILITY - Joints influence the stability of rock masses. A high density of joints can make rocks weaker, leading to potential failure in structures like tunnels, slopes, and foundations. GROUNDWATER FLOW - Joints can serve as pathways for water flow, affecting groundwater levels and seepage, which can impact construction projects like dams or foundations. SUPPORT DESIGN - Engineering designs for tunnels, slopes, or foundations may need additional support if the rock mass has extensive jointing. FOLDS SLOPE STABILITY - In hilly or mountainous areas, the orientation of folds can impact slope stability. folded rocks can lead to increased landslide risks, affecting road or building constructions. TUNNEL ALIGNMENT - Tunneling projects must consider the orientation of folds to avoid excessive excavation difficulties and reduce structural stress. FAULTS SEISMIC RISK - faults are often associated with seismic activity, building structures near active faults increases the risk of earthquake damage, requiring special design considerations (e.g... reinforced structures, seismic retrofitting). DIFFERENTIAL SETTLEMENT - faults can lead to uneven settling of foundations, causing structural instability. TUNNEL AND DAM CONSTRUCTION - faults can weaken rock masses, complicating tunneling, dam construction, and slope stabilization projects. They may serve as water conduits, increasing the risk of leaks or seepage. SLOPE STABILITY - fault zones can create zones of weakness that may lead to landslides, requiring reinforcement or modification of slope angles in affected areas. REPORTERS: Agas, Mary Ann M. (PPT, revise and expand the combined report, provide more details, such as images and additional information) Cabanas, Renz Angelo I. (PPT, Consolidate the report) Colangoy, Curt Ansley M. De Leon, Aira Rica A. Galac, Bill Herald Jasper A. Lagos, Gian Mari Carlo Minon, Jaydee Paddanan, Melvin Tizon, John Rommel DP. PHYSICAL AND MECHANICAL PROPERTIES OF ROCKS Physical Properties of Rocks (Reported by Escalante) - Are characteristics describe how rocks behave in response to electromagnetic fields, environmental conditions, and other factors. Physical Properties of Rocks include: 1. Density: A physical property of rocks 2. Porosity: The percentage of void space in a rock 3. Water content: The amount of water in a rock 4. Degree of saturation: A physical property of rocks 5. Pore number: A physical property of rocks 6. Permeability: A physical property of rocks 7. Propagation velocity: The velocity of elastic or ultrasonic waves in a rock 8. Thermal properties: A physical property of rocks 9. Electrical conductivity: A physical property of rocks that influences how rocks respond to electromagnetic fields 10. Dielectric permittivity: A physical property of rocks that influences how rocks respond to electromagnetic fields 11. Magnetic permeability: A physical property of rocks that influences how rocks respond to electromagnetic fields Mechanical Properties of Rocks (Reported by Escalante) - Describe how rocks react to external forces, such as pushing, pulling, or twisting. Physical Properties (Reported by Escalante) 1. Mineralogical Composition - -The chemical composition of a mineral determines its properties, including its color, density, hardness, and shape. 2. Crystal structure - The crystal structure of a mineral is determined by the conditions under which it forms, such as temperature and pressure. 3. Rock texture - The texture of a rock is determined by the size, shape, and arrangement of its mineral grains or crystals. The texture of a rock can also be affected by the rock's homogeneity, or how uniform its composition is throughout. 4. Rock classification - Rocks are classified based on their composition and texture. For example, igneous rocks are classified based on their composition and texture, and the texture of an igneous rock is primarily determined by how quickly it cooled during formation. 5. Rock analysis - Analyzing a rock's texture can provide information about its source material, geologic history, and deposition conditions. Specific gravity (Reported by Laurente) It is the ratio of solids' density to water's density. Specific gravity is a ratio that measures the density of a substance relative to the density of a reference material, usually water. It's also known as relative density. 𝐷𝑒𝑛𝑠𝑖𝑡𝑦 𝑜𝑓 𝑡ℎ𝑒 𝑂𝑏𝑗𝑒𝑐𝑡 𝑃 Specific Gravity = 𝐷𝑒𝑛𝑠𝑖𝑡𝑦 𝑜𝑓 𝑊𝑎𝑡𝑒𝑟 = 𝑃 𝑂𝑏𝑗𝑒𝑐𝑡 𝐻2𝑂 Below is a table that lists the value of specific gravity for some common materials. Density Of Water In Various Units - 1000 kilograms per cubic meter - 1 gram per cubic centimeter - 62.43 pounds per cubic foot - 0.036 pounds per cubic inch Unit weight (Reported by Laurente) Unit weight, also known as specific weight, is one of the terms that loosely describe and relate the size, amount of concentration, force, and specific gravity. Unit weight is the weight of a material per unit volume, also known as specific weight. The symbol for unit weight is the Greek letter gamma (γ). 2 It is usually measured in 𝑘𝑔 · 𝑚/𝑠 which is equal to Newton p = density; g = gravity acceleration W = weight; V = volume Following is the table with the SI unit, CGS unit, and the dimension of weight: Other units known are pound-force (lbf), slug, and gram, amongst others. Converting The Units of Weight - 1 g = 0.001 kg - 1 mg = 0.000001 kg = 0.001 g - 1 centigram = 0.00001 kg = 0.01 g - 1 metric ton = 1000 kg (Morales, Kyle Matthew) Density is a fundamental physical property of rocks, defined as mass per unit volume, it's expressed in units of mass per unit volume, typically grams per cubic centimeter (g/cm³) or kilograms per cubic meter (kg/m²) Void Ratio - The void ratio (e) is a measure of the proportion of voids (pores, fractures, and cavities in a rock's total volume Porosity - Porosity (p) is a measure of the void space in a rock's total volume, expressed as a percentage. It represents the proportion of pores, tractures, and cavities. MOISTURE CONTENT AND DEGREE OF SATURATION (VELASCO) Moisture Content The moisture content is the ratio of the weight of water present in the soil sample to the weight of the dry soil sample whereas the degree of saturation is the ratio of the volume of water present in the soil sample to the volume of the void present in the soil sample. Generally, the moisture content is expressed in terms of percentages, so the moisture content formula is given as: MCd = mh2o / md (1) where MCd = moisture content on dry basis mh2o = mass of water (kg, lb) md = mass of dry solid (kg, lb) Degree of Saturation The ratio expressed as the volume of water present in the soil sample to the volume of the void present in the soil sample. Generally, the degree of saturation is expressed in terms of percentages, so the degree of saturation formula is given as Degree of Saturation (%) = (Amount of solute dissolved / Maximum amount of solute that can be dissolved) × 100 Degree of Saturation (S) = (Vw / Vv) × 100 Where: S = Degree of saturation (%) Vw = Volume of water in the soil sample Vv = Volume of voids in the soil sample RECAP! Moisture content: Is the quantity of water contained in a material such as soil, rock, wood and other solid material. Often referred to as water content. Saturation %: Is the ratio of the volume of water compared to the volume of voids within a matrix. Generally expressed as a percentage. For example it is equal to zero when a soil is absolutely dry and 100% when the soil is fully saturated. PERMEABILITY AND ELECTRICAL AND THERMAL PROPERTIES (COLMO) Permeability Properties (COLMO) This refers to a porous material's ability to let liquid pass through its pores. Porosity and permeability are related properties of any rock or loose sediment, both related to the number, size, and connections of openings in the rock. In the top diagram, even though there is good porosity, there is material in the rock to fill in the gaps blocking the pore space between the grains so the water at the bottom cannot flow to the top the pores are not connected. In the diagram on the bottom, there is nothing to stop the flow of water; the pores are all connected. Electrical Properties (COLMO) The majority of rocks are dielectric, and measurements of dielectric constants are used to interpret data. Electrical Conductivity and Resistivity: Rocks exhibit a wide range of electrical resistivity, depending on their composition, porosity, fluid content, and temperature. For example, metallic ores have low resistivity, while dry, crystalline rocks have high resistivity Conductivity is the inverse of resistivity and is influenced by factors such as ion concentration in pore fluids and temperature. Saline water in pores significantly increases rock conductivity compared to dry or freshwater-filled rocks. Porosity and Fluid Content: The presence of fluids in rock pores, particularly saline water, dominates electrical conductivity. Archie's law relates rock porosity, fluid conductivity, and bulk conductivity, providing a framework for interpreting subsurface properties. Temperature Effects: Higher temperatures enhance ion mobility in pore fluids, increasing conductivity. However, this behavior contrasts with metallic conductors, where resistivity increases with temperature. Dielectric Properties: Rocks' dielectric constants reflect their ability to store electrical charge, influenced by mineral composition, fluid saturation, and temperature. This property is vital in high-frequency applications such as radar-based geophysical surveys. Thermal Properties (COLMO) The increase in temperature also makes rock weaker due to the formation of cracks in the rock mass. THERMAL PROPERTIES (COLMO) Increased temperature weakens rocks due to several factors: Thermal Expansion: Different minerals expand at different rates, creating internal stresses that lead to cracks. Thermal Cycling: Repeated heating and cooling cause expansion and contraction, which can fracture the rock. Brittleness: High temperatures make rocks more brittle, increasing the likelihood of fractures under stress. Chemical Weathering: Heat accelerates chemical reactions, destabilizing minerals and forming weak zones. Hydration/Dehydration: Some minerals swell or shrink with temperature changes, promoting crack formation. Weakened Mineral Bonds: High temperatures reduce the strength of mineral bonds, making the rock more porous and prone to cracking. *These factors combine to reduce rock strength and promote fracture formation at higher temperatures. Swelling, Anisotropy, and Durability (Alzate, Diego) SWELLING is the enlargement or the increasing in volume of rocks over time, due to increase in water content or changes in stress. POSSIBLE CAUSES: Low porosity of crystalline rocks. ( granites, diorites, gneisses and metabasites) These rocks swell when saturated with water and shrink when dried out. Sorption and desorption of water. Surface forces and pore pressure. ANISOTROPY The variation of rock properties depending on their direction. DURABILITY Ability of rocks to resist deterioration, weathering and degradation. Maintaining their properties and Integrity. MECHANICAL PROPERTIES OF ROCK (Reported by San Diego, John Brenan B.) -Imagine you're building a bridge. You need a material that's strong enough to hold the weight of cars, flexible enough to withstand wind gusts, and durable enough to last for years. This is where mechanical properties come in! They describe how materials behave when subjected to forces, like pulling, pushing, bending, or twisting. Compressive Strength - The compressive strength of a material is a measure of its ability to resist uniaxial compressive loads without yielding or fracture. - Uniaxial compressive strength is given by the ratio of load at failure or rupture to cross-sectional area of the specimen. DIRECT METHOD UCS represents the maximum axial compressive stress of a cylindrical rock. specimens can withstand before failing when subjected to a load applied in a single direction (uniaxially) without any lateral confinement. It is a fundamental geomechanical parameter used to assess the strength of intact rock samples -Uniaxial Compressive Strength (UCS) is a fundamental geomechanical parameter used to assess the strength of intact rock samples. It represents the maximum compressive stress a cylindrical rock specimen can withstand before failing when subjected to a load applied in a single direction (uniaxially) without any lateral confinement. This means that the rock is being squeezed from only one direction, and there is no pressure pushing on the sides of the sample. This is important because it allows engineers to get a basic understanding of how strong the rock is in a simple, controlled setting. The UCS test is often used to determine the strength of rocks that will be used in construction projects, such as foundations, tunnels, and slopes. The UCS is measured by taking a cylindrical sample of rock and applying a compressive force to it until it breaks. The maximum force that the sample can withstand before breaking is then used to calculate the UCS. Triaxial compression tests are a more sophisticated laboratory method used to assess the shear strength of soils and rocks under controlled stress conditions These tests subject a cylindrical specimen to a confining pressure (lateral stress) while simultaneously applying an axial load. How it works: - Cylindrical Specimen: A cylindrical sample of the soil or rock is used for the test. - Confining Pressure: The specimen is placed in a chamber where it's surrounded by a fluid (like water or oil). This fluid is pressurized, creating a confining pressure or lateral stress on the sample. This simulates the pressure the material would experience from the surrounding soil or rock in the ground. - Axial Load: At the same time, a vertical load is applied to the top of the specimen. This is called the axial load. This simulates the weight of a building, embankment, or other structure that would be placed on the soil or rock in the field. By applying both confining pressure and axial load, the triaxial test creates a three-dimensional stress state within the specimen. This allows engineers to measure the shear strength of the material, which is its resistance to forces that tend to cause it to slide or deform. This test is important because it provides a more realistic picture of how soil and rock will behave under real-world conditions, which is crucial for designing safe and stable structures. INDIRECT METHOD The point load test (PLT) is a widely used index test for assessing the strength of intact rock samples. It is a relatively simple and cost-effective method that can be performed both in the laboratory and in the field. How it works: - Loading: The PLT involves applying a concentrated load to a rock specimen using two hardened steel points. - Failure: The load is increased until the specimen breaks. - Strength Index: The force required to break the specimen, along with the distance between the loading points, is used to calculate a point load strength index (Is50). This index provides a measure of the rock's strength. The Schmidt hammer test (rebound hammer test) is a non-destructive method used to assess the surface hardness and penetration resistance of concrete. It is widely used for quality control and in-situ assessment of concrete structures. How it works: - The Hammer: The Schmidt hammer is a handheld device with a spring-loaded mass inside. - Impact and Rebound: When the hammer is pressed against the concrete surface, the mass is released, striking the surface with a known force. The mass then rebounds back, and the distance of this rebound is measured. - Rebound Number: The rebound distance is recorded as a rebound number, which is an arbitrary value on a scale from 10 to 100. This number is directly related to the hardness of the concrete surface. - Correlation to Strength: While the rebound number doesn't directly measure the compressive strength of the concrete, it can be used to estimate it. This is done by comparing the rebound number to a calibration chart that relates rebound numbers to known compressive strengths. Why is it useful? - Non-destructive: The Schmidt hammer test doesn't damage the concrete, making it ideal for testing existing structures. - Quick and Easy: The test is relatively quick and easy to perform, making it suitable for large-scale assessments. - Quality Control: It helps ensure the quality of concrete during construction by comparing the rebound numbers to expected values. - In-situ Assessment: The test can be used to assess the condition of existing concrete structures, identifying areas of weakness or damage. Tensile Strength (Reported by Calulo, Carlo Miguel G.) The maximum mechanical tensile (pulling) stress that a specimen can withstand before failure, although the definition of failure varies depending on the material's type and its design. Is a measure of the ability of a rock to resist breaking or fracturing under tension. It refers to the maximum stress a rock can withstand when being stretched or pulled apart before it fails. Tensile strengths have dimensions of force per unit area and in the English system of measurement are commonly expressed in units of pounds per square inch, often abbreviated to psi. To correctly measure tensile strength, we divide the force per unit of a cross-sectional area of the material instead of its whole surface. But you won’t be able to calculate a material’s tensile strength directly without first knowing its stress-strain curve. Here’s the equation: σ = F/A – eq 1 σ = stress F = force A = area The SI tensile strength unit is MPa (N/mm2). Basically, the strength equals the stress at the point of failure of the material, and this failure point can be characterized by the force per unit area. Types of Tensile Strength Yield Strength - Yield strength is the point at which permanent (or plastic) deformation begins in a material. For steel, this is once it’s been deformed past its proportional limit, usually seen as a straight line on a stress-strain curve. For material without a distinct yield point, like aluminum, yield strength is reported as the stress that coincides with 0.2% strain. In most cases, metal parts are designed to keep stresses in the service environment below the yield strength. In the case of very brittle materials, like ceramics, these will fail at very small deformations and do not yield at all. Other materials, like elastomers, can be stretched many times their original length without breaking and only show a small amount of permanent deformation once the load has been removed. Breaking Strength - Also known as fracture strength, this refers to the point at which the material can no longer carry any more load and breaks. This can be seen as the end point of a stress-strain curve, i.e. point “ε” in the yield strength diagram above. The breaking point is also the point used for measuring total elongation. Ultimate Strength - This is the maximum stress a material can handle before it ends up breaking under a tensile load. Some metals will experience strain hardening after passing their yield point, and they’ll continue to deform as the stress increases until they get to the material’s ultimate tensile strength. Not all materials, for instance plastics, will have a well-defined ultimate tensile strength. Shear Strength (reported by Garcia, Dominic) - The maximum resistance to deformation caused by shear stress is known as shear strength. Surface frictional resistance along the sliding plane, interlocking between individual rock grains, and cohesion in the sliding surface of the rock all contribute to the shear strength of a rock mass. Shear Strength Tests It primarily concerns the shear strength and shear behavior of the rock's shearing and weakness planes, which hold a rock specimen together. This is the most expensive laboratory strength test because it necessitates a unique approach for collecting samples from the site as fracture planes to be tested, as well as the most complex testing procedures. It mostly deals with the shear strength and shear behavior of the shearing and weakness planes of the rock which hold together a rock specimen. It is a vital geomechanics measure, used for design In general there are two methods for evaluation of Shear Strength of rocks; 1. Direct Shear Test a. Shear Box Test b. Shear Test on Rock Cubes 2. Indirect Shear Test-Punch shear Test Ring Shear Test: This test is used to measure the shear strength of soil. It involves placing a soil sample in a ring-shaped chamber and applying a vertical load and a horizontal shear force. The shear force required to cause failure within the soil is measured. Older Model (Lever-Arm Loaded Ring Shear): * Vertical Load: Weights are manually added to a lever arm to apply the vertical load on the soil sample. * Horizontal Shear Force: A motor-driven mechanism applies the horizontal shear force. Newer Model (Fully Motorised Ring Shear): * Vertical Load: Two independent motors apply the vertical load, eliminating the need for manual weight addition. * Horizontal Shear Force: A separate motor applies the horizontal shear force. Shearing is associated with: Borehole instabilities, breakouts, failure Reservoir shear and induced seismicity Casing shear and well collapse Reactivation of old faults, creation of new ones Hydraulic fracture in soft, weak reservoirs Loss of cohesion and sand production Bit penetration, particularly PCD bits Reported by: Escalante, Alvin Alzate, Diego Garcia, Dominic Anthony Laurente, Yla Miyaka Colmo, Klyde Daniel E. Calulo, Carlo Miguel G. San Diego, John Brennan Velasco, Edlawrence Aloysius Morales, Kyle Matthew References Admin. (2023, February 1). Specific Gravity - Definition, Calculation, solved examples, FAQs. BYJUS. https://byjus.com/physics/specific-gravity/ Admin. (2022, August 2). What is the Unit of Weight? - SI Unit, CGS Unit and Other Units. BYJUS. https://byjus.com/physics/unit-of-weight/#:~:text=Therefore%2C%20the%20SI%20unit% 20of,which%20is%20equal%20to%20N. Sankar, U. (2010). Physico-Mechanical Properties of Rock Materials. Retrieved from https://www.slideshare.net/sankarsulimella/physicomechanical-properties-of-rock-materi als Jaeger, J. C., Cook, N. G. W., & Zimmerman, R. W. (2007). Fundamentals of rock mechanics. Wiley-Blackwell. Goodman, R. E. (1989). Introduction to rock mechanics. Wiley. Pilogan, R. (n.d.). Mechanical Properties of Rocks [Presentation]. Retrieved from Scribd: https://www.scribd.com/presentation/510237699/MECHANICAL-PROPERTIES-OF-RO CKS The Editors of Encyclopaedia Britannica. (2024, October 26). Tensile strength | Definition, Unit, & Facts. Encyclopedia Britannica. https://www.britannica.com/science/tensile-strength De Naoum, K., & Piccoli, R. (2024, November 19). Everything you need to know about tensile strength. Xometry. https://www.xometry.com/resources/3d-printing/tensile-strength/ https://education.nationalgeographic.org/resource/weathering/ Axel Schult, ( October 1997) Hydration Swelling of Crystalline Rocks https://academic.oup.com/gji/article/131/1/179/751518 Republic of the Philippines UNIVERSITY OF RIZAL SYSTEM Antipolo City Engineering Program Properties of Rocks and the Factors influencing the Wave velocity Geology for Civil Engineer Reported by Bardalo, Edgardo Jr E. Capelo, Jedidiah Esquilon, Khyla Lorraine M. Holasca, Pia Franceska Lozada, Jessica P. Nene, Kyla P. Sibayan, Anjelo A. Vinzon, Lovely D. 1. Properties of rocks (Holasca, Pia) a) Hardness - Hardness of mineral can be tested by doing a scratch test b) Streaks - Describe the color left by the rock when drawn across hard surface c) Luster - Describe the appearance of the mineral’s surface d) Color - Describe the color and color combinations e) Density - Describe the weight of the rock 2. Wave velocity - Wave velocity is the velocity with which the wave propagates thru the medium - It is also called Phase velocity - Velocity of transmission of wave where as the velocity of particle vibrations - It is the measure of how quickly the energy of the wave travels from one point to another. A. Wavelength (Bardalo, Edgardo) - Wavelength is the distance between two consecutive crests or troughs of a wave. Imagine a wave as a series of peaks and valleys. The distance between two peaks (crests) or two valleys (troughs) is the wavelength. - Longer Wavelength: Longer waves, like those generated by strong storms, have more energy distributed over a greater distance. They encounter less resistance from the water and therefore travel faster. - Shorter Wavelength: Shorter waves, like those created by a gentle breeze, have less energy concentrated over a smaller area. They experience more friction with the water, slowing them down. B. Wave Frequencies (Sibayan, Anjelo) - Number of complete cycles or waves that occur in a given amount of time, measured in hertz (Hz). - High-frequency music has lots of quick changes in the sound waves, and it feels fast and energetic.Low-frequency music has slower changes in the sound waves, and it feels calm and soothing. - Sound travels faster in denser, more elastic materials because particles in solids are closer together, making it easier for the wave to transfer energy. In hard rocks like granite, sound waves will travel faster than in softer rocks or liquids. C. Temperature (Capelo, Jedidiah) - High temperature leads to a higher rate of vibrations of the molecules. Thus, they can travel faster, such as in sound waves. The higher the temperature, the faster the wave’s speed rate in a given time period. D. Elasticity (Esquilon, Khyla) - Elastic properties relate to the tendency of a material to maintain its shape and not deform when a force is applied to it. Waves can travel quicker through materials with higher elastic qualities like steel than through solids with lower elastic properties like rubber. For instance, when there is an earthquake, seismic waves are faster on solid grounds than soft grounds due to their great elasticity. That is why softer grounds cause significantly more harm than solid ground. E. Density (Esquilon, Khyla) - The mass per unit volume of a material influences wave velocity, as denser materials often allow for faster transmission of waves. The density of the material affects the flow of waves; the more dense the material is, the waves move slower because the particles are heavy. That is why, even though the material contains high elasticity, if the material is dense, the wave will move slowly. But if its density and elasticity are balanced, the transmission of waves will go faster. Iron, platinum, and lead are some examples of dense materials. F. Wave Type (Holasca, Pia) - P waves: Compressional waves and the speed is directly proportional to the compressibility of the material. - P-waves move in the same direction that the wave is moving. Moreover, P-waves are also the first one that will be detected once earthquakes occur. - S waves: Shear waves and the speed is slower than P-waves also it is perpendicular in the direction the wave is travelling in. - S-waves are the second that will be detected once the earthquake occurs and S-waves move rock particles up and down or side to side. - According to Wave Theory, wave type may influence the wave velocity since we have P-waves and S-waves. They are both moving fast through the rigid materials and move slowly through more dense material. G. RockTypes (Lozada, Jessica) Understanding the factors influencing compressional wave velocity is crucial for geological and crustal studies. Research by Condi (2016) highlights that compressional wave velocity at conditions of 600 MPa and 300°C varies significantly based on rock type, composition, and mineral content rather than just metamorphic grade. 5 Categories in wave velocities 1. Velocities Under 6.0 km/s - These velocities are typically associated with lower-density rocks or rocks that have undergone significant alteration. Such rocks are often characterized by reduced wave transmission capacity due to porosity, fractures, or lower mineral density. Rock Types: ○ Serpentinite: A rock formed by the hydration and metamorphism of ultramafic rocks, with a relatively low density. ○ Metagraywacke: A metamorphosed sedimentary rock with varied mineral composition. ○ Andesite: A fine-grained volcanic rock. ○ Basalt: A mafic volcanic rock, which can appear in altered forms that reduce its velocity. 2. Velocities Between 6.0 and 6.5 km/s - This range is common across diverse geological formations and includes both sedimentary and igneous rocks that exhibit moderate density and homogeneity. Rock Types: ○ Slates: Fine-grained, foliated metamorphic rocks derived from shale. ○ Granites: Coarse-grained igneous rocks rich in quartz and feldspar. ○ Altered Basalts: Basalts that have undergone chemical or mineralogical changes. ○ Felsic Granulites: High-grade metamorphic rocks with felsic compositions. 3. Velocities Between 6.5 and 7.0 km/s - This range is characterized by denser, more compositionally uniform rocks, often containing mafic minerals. Rock Types: ○ Amphibolites: Rocks dominated by amphibole minerals, often formed through high-grade metamorphism. ○ Mafic Granulites (without Garnet): Metamorphic rocks with mafic composition but lacking garnet. Exceptions: ○ Marble: A metamorphic rock formed from limestone. ○ Anorthosite: A rock composed predominantly of plagioclase feldspar. These exceptions are minor components of the Earth's crust but can occasionally exhibit velocities within this range. 4. Velocities Between 7.0 and 7.5 km/s - Denser rocks with high mafic content fall within this range. These rocks are often indicative of deeper crustal or upper mantle origins. Rock Types: ○ Gabbro: Coarse-grained mafic igneous rock. ○ Garnet Amphibolite: A variety of amphibolite enriched with garnet minerals. ○ Mafic Garnet Granulite: High-grade metamorphic rock containing garnet. 5. Velocities Above 7.5 km/s - This range is associated with extreme density and high levels of metamorphism, often representing deep crustal or mantle-derived rocks. Rock Types: ○ Non-Serpentinized Ultramafic Rocks: Dense rocks with minimal alteration, often from the Earth's mantle. ○ Eclogite: High-pressure metamorphic rock composed predominantly of garnet and omphacite. Key Observations 1. Velocity Trends and Metamorphic Grade: Compressional wave velocity does not directly correlate with metamorphic grade. Rocks with low-, medium-, and high-grade metamorphism overlap in velocity ranges (6.0–7.5 km/s), underscoring the complexity of crustal material properties. 2. Influence of Composition and Mineral Content: The mineralogical composition and the density of constituent minerals play a more critical role in determining velocity than the degree of metamorphism. 3. Geological Implications: ○ Crustal Analysis: Identifying compressional wave velocities aids in mapping subsurface structures and understanding tectonic processes. ○ Seismic Interpretation: Velocity trends help geologists interpret seismic data more accurately, linking wave propagation to rock properties. H. Depth (Vinzon, Lovely) A distance down from the top surface to the bottom. a measure of how deep something goes Depth as Factor Influencing Wave Velocity The compression of the rocks and level of compress, dramatically with depth is related to the seismic-wave velocities waves tend to increase its velocity in accordance with depth through the earth crust and mantle. waves undergo physical changes in the medium The deeper the wave travels, the faster the wave wave direction changes as it moves through various depths of materials. A 0 to 30 kilometer depth of the crust from the surface has 3.0 to 4.6 km/s S-wave velocity and 5.3 to 7 km/s P-wave velocity. A 30-100 kilometer depth of asthenosphere from the ground has 4.6 to 5.8 km/s S-wave velocity and 7 to 8.7 km/s P-wave velocity. In the asthenosphere, 100 to 250 km below the ground has 5.0 to 5.9 km S-wave velocity and 7.8 to 8.5 km P-wave velocity This region of the Earth is also called the Low Velocity Zone. In this area the seismic waves pass through materials that cause the waves to be slowed. Around the mantle with 250 to 2890 km depth, also the largest part of Earth's interior has a 5 to 7 km/s S-wave velocity and 8.2 to 12.6 km/s P-wave velocity. The core despite being the deepest part of Earth’s interior there is 0 km/s S-wave velocity because S-wave cannot travel through liquid but P-wave increases its velocity between the liquid outer core and the solid inner core. Wave velocity increases at 660 km depth due to Mineralogical Transition. Velocity slows down or decreases in the core-mantle boundary because this area is the Ultra- Low Velocity Zone or the Double Prime Layer; it is because this zone or area is mapped to have a diameter of a hundred kilometres and is tens of kilometres thick. This part also has only 30% of shear lower than the materials in the surroundings. I. Poisson's ratio (Nene, Kyla) Poisson's ratio increases with density, which changes as the composition of rock shifts from felsic to mafic. It also increases with temperature. - Density and Composition: Felsic rocks (rich in feldspar and quartz) are less dense than mafic rocks (rich in basalt and gabbro). As density increases, Poisson's ratio generally rises. - Temperature: As temperature increases, the velocity of shear waves (S-waves) decreases faster than the velocity of compressional waves (P-waves). This change in wave velocity affects Poisson's ratio, which is calculated using these velocities. - Quartz and Feldspar Content: Poisson's ratio tends to increase as feldspar content increases and decreases as quartz content decreases.Quartz: Quartz is a relatively stiff mineral with a low Poisson's ratio. It tends to resist deformation in the direction perpendicular to the applied stress. - Feldspar: Feldspar minerals are generally less stiff than quartz and have a higher Poisson's ratio. They tend to deform more readily in the direction perpendicular to the applied stress. References: Earle, S. (2019). Physical Geology – 2nd Edition. Victoria, B.C.: BCcampus. Retrieved from https://opentextbc.ca/physicalgeology2ed/. JUNDA, J. (2021).Factor influencing wave velocity. Eastern Samar State University,9. https://gpg.geosci.xyz/content/physical_properties/seismic_velocity_duplicate. IOWA State University. “Nondestructive Evaluation Physics : Sound.” Nondestructive Evaluation Physics : Sound, https://www.nde-ed.org/Physics/Sound/speedinmaterials.xhtml. “Unit of Density - Density Definition, SI unit, Solved Examples.” BYJU'S, https://byjus.com/physics/unit-of-density/. GeoSci Developers. “Seismic Velocity.” 2017, https://gpg.geosci.xyz/content/physical_properties/seismic_velocity_duplicate.