Engineering Teachers' Resource Book (Gr11 Term 3) PDF
Document Details
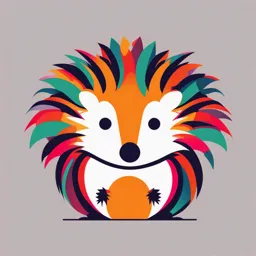
Uploaded by ConciliatorySugilite837
Tags
Summary
This resource book is for grade 11 engineering teachers. It covers energy, battery technology, and related topics. The content includes discussions on different forms of energy, the law of conservation of energy, electricity generating structures, and applications of battery technology. Relevant activities and exercises are also incorporated.
Full Transcript
Contents THEMA: ENERGY..................................................................................................................... 2 INTRODUCTION....................................................................................................................... 3 MODULE 1: HARNESSING...
Contents THEMA: ENERGY..................................................................................................................... 2 INTRODUCTION....................................................................................................................... 3 MODULE 1: HARNESSING ENERGY.................................................................................... 3 TOPIC 1: PROSPECTING THE POWER OF ENERGY...................................................... 4 TOPIC 2: ELECTRICITY – ELECTRICAL ENERGY..........................................................10 TOPIC 3: ELECTRICITY GENERATING STRUCTURES..................................................17 THEMA: BATTERY TECHNOLOGY.........................................................................................42 INTRODUCTION......................................................................................................................43 MODULE 1: CONNECTING BATTTERIES............................................................................43 TOPIC 1: INTRODUCTION TO BATTERIES.....................................................................43 TOPIC 2: ELECTROSTATICS...........................................................................................50 TOPIC 3: APPLICATION OF BATTERY TECHNOLOGY...................................................60 THEMA: ENERGY INTRODUCTION In science, Energy is considered to be a universal concept that is used to ensure work done. It can be manifested in different forms which perhaps contribute towards its versatility in usage and applications. Energy however cannot be considered a substance or ‘thing’ but perhaps an innate characteristic possessed by all things. Though perceived invisible, it can be observed and measured indirectly through its effects on matter that either acquire, lose, or possess it. Modern civilizations exist and thrive today because of how they capture and generate energy to effectively use and this has rapidly transitioned over Earth’s saga. Making fire, riding a bicycle, using fossil fuels for generators, capturing the dynamic flow of water in rivers and seas as hydro-energy and harnessing nuclear energy from the atomic chain reaction of uranium are some examples that employ and utilize energy. Much of the development in energy generation is contributed by the advancements in technology particularly in researching and prospecting the potential of different energy sources. The effective use of energy is therefore influential as it will dictate the amount of power that will be attained and generated. MODULE 1: HARNESSING ENERGY 3 Weeks In this module, Energy is emphasized as omnipotent and in abundance. The module aims to provide and extend this conception to instill in students the principles of Energy and its limitless potential. There are four topics that will expounded; Prospecting the power of Energy; Energy Generating structures using flowing water as an example; Energy Generators; and electricity. TOPIC 1: PROSPECTING THE POWER OF ENERGY Energy is an infinite resource that is ubiquitous and has always existed throughout the history of the universe. From outer space such as the Sun and black holes to Earth’s flowing waters and its heated core; energy exists and is manifested in different forms. This topic aims to provide and instill in students to prospect the power of energy and its boundless potential. Key Take Home Messages: Energy is closely related to work and power. The two major forms of energy are potential and kinetic energy Law of Conservation of Energy states that “Energy can neither be created nor destroyed, only transferred”. All work is done through the conversion of energy Activity 1: Introduction to Energy: Group Discussion In this activity, students will be introduced to energy and its main concepts. Here, they will understand the Law of conservation of Energy and the significance of this governing principle. Procedure: 1. With the attached reference materials and background research, clearly define Energy and Power and its related concepts. 2. Explicitly state and describe the law of conservation of Energy and support using a relevant example. Exercise: Below contains information on the Introduction to Energy that corresponds to questions in the SRB. Assist students in understanding the key concepts in Activity 1. 1. Definition of Energy and its related terms In Physics, Energy is defined simply as the ability or capacity to do work. Work is defined as applying a force that is moved through a distance. This is mathematically expressed as; 𝑾𝒐𝒓𝒌, 𝑾 = 𝑭 × 𝒅 𝒘𝒉𝒆𝒓𝒆 𝑭 = 𝑭𝒐𝒓𝒄𝒆 (𝑵) 𝒘𝒉𝒆𝒓𝒆 𝒅 = 𝒅𝒊𝒔𝒕𝒂𝒏𝒄𝒆 (𝒎) Work can also be defined as a transfer of energy from one system to another that results in a conversion of energy. The SI Unit for measuring Work (and Energy) is in Joules (J) which is equivalent to the Work done by a force of 1 Newton over a distance of 1m. This is mathematically expressed as; 𝑾𝒐𝒓𝒌, 𝑾 = 𝑱 = 𝑵⁄𝒎 (𝑵𝒆𝒘𝒕𝒐𝒏 𝒑𝒆𝒓 𝒎𝒆𝒕𝒆𝒓) Power in physics is defined as the rate at which work is done. The SI unit for measuring Power is in Watts (W) which is equivalent to 1 Joule of work done in a duration of 1 second. This can be mathematically expressed as; 𝑷𝒐𝒘𝒆𝒓, 𝑷 = 𝑾⁄𝒕 = 𝑱⁄𝒔 (𝑱𝒐𝒖𝒍𝒆𝒔 𝒑𝒆𝒓 𝒔𝒆𝒄𝒐𝒏𝒅) The utilization of Energy to do work over time is Power. For example, you pay for electric energy for use at your home that PNG Power transmits through the electrical grids via transmission lines. The electric energy you purchased is monitored through an energy metering box that measures it in kilowatt-hours (kWh). It basically displays how much of the electric energy that can be used. When you connect an electric appliance and use for a certain period, the quantity used to measure the energy used is Power. 2. Forms of Energy All energy is either from a basic chemical or physical processes. This thus translates energy into two general forms; Potential Energy – it is stored energy which is dependent on position. Types of Potential energy includes: o Chemical Energy is the energy stored in the bonds of atoms and molecules. It is the energy that holds these particles together, and can be obtained from food, biomass, petroleum, natural gas, propane, etc. o Elastic Energy is the energy stored by the application of force on an object. Examples include compressed springs and stretched rubbers. o Nuclear Energy is the energy harnessed from either splitting or combining the nucleus of atom. o Gravitational Potential Energy is energy possessed by a body due to its position on place. Kinetic Energy – energy derived from motions of waves, electrons, atoms, molecules, substances, and objects. Types of kinetic energy may include; o Electrical Energy is the energy produce by the movement of electrons. The movement of electron through a wire is known as electricity. o Radiant Energy or electromagnetic energy is the energy possessed in the propagation of transverse waves. o Thermal Energy also termed heat is the internal energy of a body due to the movement of atoms and molecules resulting in the change in temperature. o Motion Energy or mechanical energy is the movement of objects from one position to another. According to Newton’s Laws of Motion, an object moves due to the application of an unbalanced force o Sound energy is the movement of energy through a substance in longitudinal waves. Sound is a result of forces enabling an object to vibrate. 3. Law of Conservation of Energy Statement of the Law of Conservation of Energy o The law of Conservation of Energy states that; “Energy can neither be created nor destroyed – only converted from one form to another” Explanation o Basically, energy cannot be created from nothing and is thus described not as a “thing” that is self-existent but an attribute of matter (and electromagnetic spectrum – e.g., light particles) that can be manifested in different ways. o The energy conversion or transformation is the process of changing one form of energy to another that produces certain changes within a system. Changes in the total energy of systems can only be accomplished by adding or removing the energy from them. o Ideally, in a closed or isolated system (i.e., closed off from the surrounding or external influences) the total energy is always unchanging and thus conserved. This means that the energy of the system is always the same unless there is an external input that is applied to the system. o Energy is utilized when it is transformed from one form to another. o This is evident throughout history from burning carbon-based materials like wood, coal and gas or by harnessing power from the sun, wind, and water. Application: Vehicle with Manual Transmission The significant role of Engineering in energy is to prospect, investigate, design, construct and improve the capture and harnessing of energy. For vehicles that have internal combustion engines, its working mechanism and energy conversion can be explained as follows; Ignition System The car battery (usually lead [Pb] acid) provides power for starting your vehicle. To start the engine, the ignition must be switched “ON”. This can be done either through inserting and turning a key or pressing a button. When switched on, a small electrical current is sent to a starter relay or solenoid that causes a pair of contacts to close, thereby completing the circuit (see Fig. E-E-TRB-1). Figure E-E-TRB-1: Ignition System – Note: All components earthed/grounded to body of material Current flowing to the starter motor turns a shaft where attached is a pinion – a small round gear – that engages with a larger ringed gear situated around the rim of the engine’s flywheel which is a large and heavy mechanical wheel. The center of the flywheel (hub) is connected to a rod that rotates with a crank mechanism which contains a series of cranks and crankpins that convert the rotational motion into reciprocating motion through connecting rods. This cranking device is referred to as the crankshaft (see Fig. E-E-TRB-2). Figure E-E-TRB-2: Starter motor (Left) turns flywheel to rotate crankshaft and reciprocates the pistons (Right) Once the pinion turns the ringed gear, it quickly disengages from the flywheel where the engine is left to run. There are many functions of the flywheel however in the continual transmission of energy for movement; the flywheel has the ability to provide rotational inertia to keep the vehicle engine running. Otherwise, the engine will stall when the accelerator (gas pedal) is depressed. This is all done where no gears (neutral) are engaged so the vehicle does not move forward or backward unexpectedly. Engine Power and Transmission The engine block contains many components however what mainly contributes to the power of the engines are the flywheel, crankshaft and piston-cylinder mechanism. Within the engine, each piston – a disc or cylindrical metal – is housed within a cylinder block which compresses a mixture of air and fuel where it undergoes combustion from a heat source. The controlled explosion in the engine rapidly expands the gas generating pressure. The perpetual firing and resulting pressures rotate the crankshaft and provides the engine power. This power is transferred to the clutch – a device that engages and disengages power transmission - and gear box that control the movement of the vehicle. Stepping on the clutch, shifting to the first gear and simultaneously pressing the gas pedal and releasing the clutch causes the wheels to rotate and launch the vehicle forward. o Conversion of Energy in an Engine and Transmission As explained, there are different energy conversions that contribute to the working mechanism of moving the vehicle. Fig. E-E-TRB-3 can illustrate the different energy conversions that occur within the vehicle. Figure E-E-TRB-3: Energy Conversion for Moving Vehicle At rest (i.e., the car is not running), the potential chemical energy stored within the car battery is converted to electrical energy when the key is inserted and turned. The electrical energy is then converted to mechanical energy in the starter motor that rotates the pinion shaft. The mechanical energy generated interlocks with the ringed gear and rotates the flywheel and crankshaft. This rotation is then primarily driven by the controlled combustion of fuel within the piston-cylinder block. Potential chemical energy in fuel is then converted to kinetic and thermal energy in the form of expanding gas. The energy from this expanding gas is transferred into the linear piston movement that is converted into the rotary crankshaft movement; the rotary crankshaft movement is passed into the transmission assembly. The energy throughout this stage is mechanical. Kinetic energy is then generated when the clutch is engaged to the transmission assembly to shift gears. The engine power of the vehicle is then transferred onto the wheels producing torque thus moving the vehicle. The battery also converts its potential chemical energy when connected to other electrical devices within the vehicle. The radio converts the electrical energy into sound and the car lights convert its electrical energy into light. Resources: May vary based on teacher’s discretion; Chalk and Black Board Markers and White Boards PowerPoint Poster and Charts Handouts Models Experimental Presentations TOPIC 2: ELECTRICITY – ELECTRICAL ENERGY Electrical energy is a form of energy used interchangeably with electricity which refers to the movement of electrons. Electricity is an important form of energy that we have come to depend upon heavily in our daily lives and has greatly impacted and improved our standard of living. In this topic, we will introduce the concepts of electricity to the students to understand electricity and identify ways to improve its transmission, distribution, and usefulness within the society. Key Take Home Messages: Definition of Electricity and Electrical Energy Electricity generation uses the principle of Faradays’ law of electromagnetic induction Activity 1: Electricity: Group Discussion The aim of this activity is to enable students to define electricity and explain the fundamental concepts that are related to it. Procedure: 1. With the attached reference materials and background research, clearly define Electricity and Electrical Energy including other its related concepts. Exercise: Below contains information on the Electricity that corresponds to questions in the SRB. Assist students in understanding the key concepts in activity 1. 1. Definition of Electrical Energy and Electricity Electricity is simply the flow of electrons. These flow of electrons results in a form of energy called the electrical energy. In this case, the force is either from electrical attraction or repulsion between charged particles. Electrical energy can either be in the form of potential energy or kinetic energy, but the former is usually encountered. Potential Energy in this case is defined as the energy stored due to the relative positions of charged particles or electric fields. The movement of these charged particles through a wire or other medium is known as current or electricity. 2. Electrical Circuit In order to harness the usefulness of electrical energy, electricity must be conducted through an electrical circuit. A simple circuit consists of an energy source, set of conductors and an electrical load. The energy source is a component that is responsible for the production of electricity (useful energy). A common example is a battery. Electrical conductors are materials that allow the flow of electricity. Conductors are mostly in the form of wires of which the conductivity is dependent mainly upon the material type. Electrical Loads are any electrical components or portions of an electric circuit that consume electrical power. A common example is a light bulb. To obtain electricity, the electric circuit should be closed and complete meaning that all components in the must be connected. The connections can be in parallel or series or a combination of both. This circuit can include elements such as resistors, capacitors, switches, transformers, and electronics. A basic electric circuit may contain a voltage V source which acts as the force to drive the current, I or charged particles around the circuit, delivering electrical energy into the resistor, R and back to the source completing the circuit. The rate at which electric energy is transferred per unit time is known as the power. This can be calculated as; 𝑄𝑉 𝑃 = 𝑊𝑜𝑟𝑘 𝑑𝑜𝑛𝑒 𝑏𝑦 𝑡ℎ𝑒 𝑐ℎ𝑎𝑟𝑔𝑒 𝑝𝑒𝑟 𝑢𝑛𝑖𝑡 𝑡𝑖𝑚𝑒 = = 𝐼𝑉 𝑡 Where; Q = Electric charge in coulombs (C) t = Time in seconds (s) I = Electric current in amperes (A) V = Electric potential or voltage in volts (V) 3. Electrical Current Electricity or charged particles in a circuit can travel in two different ways; Direct Current (DC) or Alternating Current (AC). In a typical household, electrical devices possess currents that are either DC or AC. DC can be from stored electric energy such as batteries that has a unidirectional flow of electrons from the positive to the negative part of the circuit. AC is any current that flows in a constant changing direction with respect to time. 4. Electrical Power System A basic electrical system contains a generating station, the storage and transmission process and distribution of electricity. The load center is now where the electricity supply is being distributed to the industries and houses/buildings. This is shown in fig. E-E-TRB-4 below. Transmission Distribution System Center Generating Load Center Block Diagram of Power System System Figure E-E-TRB-4: Electrical Power System Resources: May vary based on teacher’s discretion; Chalk and Black Board Markers and White Boards PowerPoint Poster and Charts Handouts Models Experimental Presentations Activity 2: Electrical Energy Generation: Group Discussion In this activity, the fundamental concept of electrical energy generation is briefly discussed of how electricity is generated and the different methods that harness and utilize it. Procedure: 1. With the attached reference materials and background research, clearly explain the concept of electricity generation and the different methods used. 2. Students can then be grouped and tasked to distinguish and name the different sources of energy generating stations in the country. Also suggest what other sources for electricity that could be a possible solution in the valleys, oceans and mountains of Papua New Guinea that can help contribute to the national grid. Exercise: 1. Electricity generation Electricity generation is the process of generating electric power from primary energy sources such as solar, wind, geothermal, hydropower, biomass, nuclear, etc. These sources can be either renewable or non-renewable resources. This stage is prior to its storage (transmission and distribution) to end users for utilities in the power industries. Generation of electricity is carried out in power stations or in power plants. The production of electricity results from the transformation of the different types of energy sources. This is achieved by electromechanical generators, driven primarily by heat engines fueled by combustion. They can also be driven by other means such as the kinetic energy from a flowing river or wind. Fuel generates energy: o Electric-power generation starts with a source of fuel that can be harnessed to create energy. o Fuel types include fossil (coal, oil, natural gas), nuclear, and renewable (such as solar power, wind power, falling water for hydro generation, and even garbage and agricultural waste products). Renewables also reduce emissions during power generation. 2. Methods of generation Several fundamental methods exist to convert other form of energy into electrical energy. Rotating of electric generators or photovoltaic systems achieve utility-scale generation. Utilities distributed by batteries contribute only a small portion. o Electrochemistry As in a battery, electrochemistry is the direct transformation of chemical energy into electricity. Electricity generated from electrochemistry is important in portable and mobile applications. Currently, most electrochemical power comes from batteries. Primary cells act as power sources directly while secondary cells (i.e. rechargeable batteries) are used for storage systems rather than primary generation systems. Open electrochemical systems, known as fuel cells, can be used to extract power either from natural fuels or from synthesized fuels. Osmotic power is a possibility at places where salt and freshwater merge. o Photovoltaic Effect As in solar cells, photovoltaic effect is the transformation of light into electrical energy. Photovoltaic panels convert sunlight directly to DC. DC power is then converted by power inverters to AC if needed. Due to the cost of the panels, solar power electricity is still usually more expensive to produce than large-scale mechanically generated power even though we have free and abundant amount of sunlight. Commercially available now are the multifunction cells with up to 30% conversion efficiency and silicon solar cells with low efficiency have been decreasing in cost. Experiment system has displayed that over than 40% efficiency. As supplementary electricity sources for single homes or businesses, photovoltaic were mostly used in remote sites where there is no access to commercial power grid until recently. o Induction Generators Electric generators transform mechanical energy (i.e. kinetic energy) into electricity. This is the most used form for generating electricity and is based on Faraday's law. It can be seen experimentally by rotating a magnet within closed loops of conducting material (e.g. copper wire). Almost all commercial electrical generation is done using electromagnetic induction, in which mechanical energy forces a generator to rotate. Some prime movers such as an engine or turbines in general drive a rotating magnetic field past stationary coils of wire thereby converting mechanical energy to electricity. Commercial electric power generated on Earth is with turbines, driven by water, steam, wind or by burning of gas. By electromagnetic induction the turbine drives a generator, consequently transforming its mechanical energy into electrical energy. There are many different methods of developing mechanical energy, including heat engines, hydro, wind and tidal power. Most electric generation is driven by heat engines. Activity 3: Electromagnetic Induction: Group Discussion Electric generators produce electrical energy through the application of Faradays’ law that converts mechanical energy into electrical energy. Electrical energy is usually produced by an electric generator that converts mechanical energy into electrical energy that is then utilized by an external circuit. Procedure 1. Having a fair understanding of the information provided below and from other relevant sources on electric generators, clearly articulate to the students on how the electrical energy in an electric generator is produced. Exercise: 1. Working Principle of a Generator The generator does not really create the electricity, instead it is important to understand that the generator uses mechanical energy being supplied to it, that forces the movement of electric charges present in the wires through an external electric circuit in a magnetic field. This flow of electric charge creates the output current provided by the generator. This mechanism can be understood by considering the comparing a generator to a water pump: the generator causes the flow of water but does not actually "create" the water that flows through it. Generators work on the principle of electromagnetic induction discovered by Michael Faraday in 1831-32. Faraday discovered that the aforementioned flow of electric charge can be induced by moving a conductor (such as a wire containing electric charge) in a magnetic field. This movement creates a voltage difference between the ends of the electrical wire or conductor, which in turn causes the charge to flow, generating current. 2. How an electric generator produces Electro-Motive Force (EMF) Figure E-E-TRB-5: A conductor cutting the magnetic field Derivation of the emf induced in a rotating planar coil: The motional emf equation for a straight wire is given as 𝜀 = 𝑣𝐵𝐿 where v, B, and L are all perpendicular to each other. If the left vertical side of the rotating coil moves at a speed v, the component perpendicular to B is vsinθ, which when used in the above equation for emf yields as BLvsinθ. Since the right vertical side contributes the same electromotive force, it is then added to give 𝜀 = 2𝐵𝐿𝑣 sin 𝜃 , where ϴ is the angle between B and the normal to the plane of the loop. 𝑣 𝑊 𝑊 Since the angular velocity of the rotating coil is 𝜔 = , in this case 𝑟 = , then 𝑣 = 𝜔. 𝑟 2 2 ω is in radians / second, so if θ = 0 at t = 0 , then 𝜃 = 𝜔𝑡 , our expression for E becomes 𝑊 𝜖 = 2𝐵𝐿𝑣 sin 𝜃 = 2𝐵𝐿 (𝜔 ) sin 𝜔𝑡 = (𝐿𝑊)𝐵𝜔 sin 𝜔𝑡 2 For a coil of N loops and identifying 𝐴 = 𝐿𝑊 𝜖 = 𝑁𝐵𝐴𝜔 sin 𝜔𝑡 = 𝜖0 sin 𝜔𝑡 𝜀 = 2𝜋𝑓 where f Is the rotational frequency in herts (Hz). So 𝜀 = 𝜖0 sin 𝜔𝑡, 𝜀0 = 𝑁𝐴𝐵𝜔 for a maximum emf 1 2𝜋 Emf Generated is sinusoidal in time. Hence 𝑇 = 𝑝𝑒𝑟𝑖𝑜𝑑 = = 𝑓 𝜔 Example A generator with a circular coil of 75 turns of area 3.0 x 10-2m2 is immersed in a 0.20 T magnetic field and rotated with a frequency of 60 Hz. Find the maximum emf which is produced during a cycle. Solution: The maximum emf for a generator is 𝜀0 = 𝑁𝐴𝐵𝜔 We know 𝑁 = 75, 𝐴 = 3.0 𝑥 10−2 𝑚2 , 𝐵 = 0.20 𝑇 and 𝑓 = 60 𝐻𝑧. Since 𝜔 = 2𝜋𝑓 = 2𝜋(60) = 377 𝑟𝑎𝑑𝑖𝑎𝑛𝑠/𝑠 𝜀0 = (75)(3.0 𝑥 10−2 )(0.20)(377) = 170 𝑉 The electrical energy delivered by a generator and the counter torque Figure E-E-TRB-6: Electricity generated and supplied to the load or consumer When the generator delivers current, the induced magnetic force acts on its coils to make the turbine run, thus converting mechanical energy into electrical energy while maintaining energy conservation. The magnetic force produces a counter-torque opposite to the rotational movement. A reaction torque is caused by the induced force acting on the circuit. These forces are generated by the interaction of the induced current with the applied magnetic field. TOPIC 3: ELECTRICITY GENERATING STRUCTURES Electricity generating structures (often termed power plants for large scale production of electrical energy) are the chief contributors to all the power generated in the world. This topic aims to highlight some of these structures and their differing working principle in how they contribute to supplying power. Key Take Home Messages: The purpose of constructing Electricity generating Structures or Power plants Harnessing different forms of energy mainly from hydro-energy in flowing water and thermal energy in fossil fuels and uranium in nuclear energy The basic setup and fundamental role of each component within certain power plants The different types of turbines used Activity 1: Introduction to Electricity Generating Structures In this activity, students will be introduced to the different types of energy sources and the systems that are used to harness and utilize the electrical energy generated. This activity aims to ground students with the various ways in which the different forms of energy are harnessed from using these different civil structures. Procedure: 1. With the attached reference materials and background research, define and discuss the different types of electricity generating structures. Exercise: Below contains information on the introduction to Electricity Generating Structures that corresponds to questions in the SRB. Assist students in understanding the key concepts in Activity 1. 1. Define Electricity Generating Structures Electricity generating structures are industrial facilities designed to capture energy from a primary energy source and convert it into electricity. They are often referred to as power plants or stations. These structures can be divided into four common power plants: I. Thermal Power Plants II. Hydroelectric Power Plants III. Solar Power Plants IV. Wind Power Plants At large power plants, the electricity is generated most often by electromechanical generators, primarily driven by rotating a turbine from a fluid source such as water, gas, wind or steam. This turbine is attached to a shaft attached to large loops of metal coils that rotate in a magnetic field to generate electricity. This is using the fundamental principle of electromagnetic induction. An exception to power plants that do not employ turbines for power generation are solar photovoltaic power plants which use solar panels instead of turbines to generate electricity. 2. Common Components found in Electricity Generating Structures Turbines o There are different categories of classifying turbines such as their methods of construction, working pressure, size, function, and other factors. However so, considering the transfer and exchange of energy from the fluid onto the blades whether water, gas, wind or steam, there are two basic categories of turbines; I. Impulse Turbines If the turbine wheel is driven by the kinetic energy of the fluid that strikes the turbine blades through the nozzle or otherwise, the turbine is known as an impulse turbine. In these types of turbines, a set of rotating machinery operates at atmospheric pressure and are usually suitable for high head and low flow rates. Pelton, Turgo, and Cross-flow turbines are three types of impulse turbines. The construction of the Pelton and Turgo turbines is similar. However, the Cross-flow turbine is a modified type of impulse turbines that is classified as an impulse turbine due to the rotation of the runner at atmospheric pressure and not as a submerged turbine. a) Pelton Wheels (fig. E-E-TRB-7-a) are the preferred turbine for sites of the heads above 250 meters or so. The Pelton is an impulse turbine that is basically a wheel with a set of double cups or ‘buckets’ mounted around the rim. The water passes round the curved bowls, and under optimum conditions gives up almost all its kinetic energy. The power can be varied by adjusting the jet size to change the volume flow rate, or by deflecting the entire jet away from the wheel. b) Turgo Turbines (fig. E-E-TRB-7-c) are a variant of the Pelton wheel, where the double cups are replaced by single, shallower ones, with the water entering on one side and leaving on the other. It is able to handle a larger volume of water than a Pelton wheel of the same diameter which gives it an advantage for power generation at medium heads. c) The Cross-flow Turbine (fig. E-E-TRB-7-f) is another impulse type. The water enters as a flat sheet rather than a round jet. It is guided on to the blades, travels across the turbine and meets the blades a second time as it leaves. Figure E-E-TRB-7: Types of Turbine Runners II. Reaction Turbines If the sum of potential energy and kinetic energy of water which are due to the pressure and velocity that cause the turbine blades to rotate, the turbine is classified as a reaction turbine. In these types of turbines, the entire turbine is immersed in water where changes in the water pressure with the kinetic energy of the water cause power exchange. Applications of reaction turbines are usually at lower heads and higher flow rates than impulse turbines. Reaction turbines are very diverse. Francis, Kaplan, and Axial flow turbines are among these turbines. a) Francis Turbines (fig. E-E-TRB-7-a) are by far the most common type in present-day medium- or large-scale plants, being used in locations where the head may be as low as 2m or as high as 200m. They are radial-flow turbines, which means the water flow is inwards towards the center. Francis turbines can achieve efficiencies as high as 95% – but only under optimum conditions, as maintaining exactly the right speed and direction of the incoming water relative to the runner blades is important. b) Propeller or Axial-flow Turbines (fig. E-E-TRB-7-b) sweep their blades through the entire area which the water enters. They are therefore suitable for very large volume flows and have become usual where the head is only a few meters. In such turbines, the efficiency can be improved by varying the angle of the blades when the power demand changes. c) Kaplan turbines (fig. E-E-TRB-6-7-e) are axial turbines where the blade angle may be varied to improve efficiency. 3. Electricity Generating Structures Because of the varying energy sources, there are also different types of electricity generating structures however in this section, we will be focusing on two types of power plants: Thermal power plants specifically Fossil Fuel and Nuclear Power Plants and the Hydroelectric Power Plants. Thermal Power Plants: Fossil Fuel Power Plants Fossil fuels are formed from the remains of organisms which have been buried within the Earth’s crust for an extensive period under immense heat and pressure. They are all usually found in large deposits. Since its introduction and ease for accessibility and usage, it is arguably still to date the major source of almost all energy applications. From supplying engine power in vehicles, ships and planes to electric generators for powering homes in rural to urban areas; there is a heavy reliance upon fossil fuels namely coal, oil and natural gas. In regard to large-scale power generation, fossil fuels are a common type of energy source that provides most of the electrical energy used in the world. In fact, most of power stations in Papua New Guinea are fossil fuel based. Fossil fuel power plants are a type of thermal power station which generate heat from combustion to generate electricity. They employ different types of machinery to convert this thermal energy into electrical energy. In large power plants, the prime mover – a machinery that provides the main source of power to convert primary energy into mechanical energy to do useful work – may be a steam turbine, a gas turbine whereas in smaller plants, a reciprocating engine is used. This thermal energy is obtained from the working fluid as expanding gas, either from combusting gas or from heating water and producing steam. The three main fossil fuels mentioned previously clearly identify the three main types of fossil fuel power plants which include; 1. Coal Fired Power Plants 2. Diesel Fired Power plants 3. Natural Gas Fired Power plants These power plants have different working components but ultimately all share the same principle of thermal energy transfer into mechanical energy. Coal fired Power plants will be explained. Coal Fired Power Plants Coal is the solid form of fossil fuel. There are various designs and configurations for a conventional coal fired power plant, but all have similar processes. Coal is firstly processed and burnt to produce steam. This steam is channeled to expand as it rotates a turbine generator to produce electricity. The electricity generated is then fed into an electrical transmission and distribution system. The exhausted steam then enters a cooling system that condenses the steam back into water, thus repeating the cycle. The exiting gas (usually termed flue gas) from combusting coal is vented out through a smokestack where the air pollutants are filtered before it is released. From the various designs, the most common is a pulverized-coal (PC) unit. Fig. E-E- TRB-8 displays a basic schematic diagram to illustrate this power plant setup; Figure E-E-TRB-8: Schematic diagram of a coal Fired Power Plant Main Components of a Coal Fired Power Plants The main units and their respective components include the following; I. Coal and Ash Unit This unit is responsible for receiving, processing and combusting coal. It is also responsible for ash removal after combustion which is necessary for proper burning of coal. It contains the following components; Coal Pulverizer The coal supply is first introduced into a pulverizer that crushes the coal into smaller and finer particles. This is to increase its surface exposure, thus maximizing heat generated without requiring a large supply of air during combustion. The pulverized pieces are then transported to a burner via belt conveyers. Coal Burner The coal is fed into a burner for combustion. The burner is within the furnace unit known as the boiler. The burner controls the ignition mixture of coal and pre-heated air for effective combustion. For each pulverizer, there are typically four to eight burners with at least four pulverizes serving a large boiler. The coal is burnt in the boiler and the residue or ash left after the complete combustion of coal is removed to the ash handling plant and then delivered to the ash storage plant for proper disposal. II. Steam and Electricity Generation Unit The steam generation unit is responsible for the production of steam from heating water. It consists of a boiler and heat transfer equipment that produce steam and other auxiliary equipment that utilize the flue gases. It contains the following components; Steam Boiler The boiler facilitates the transfer of heat from combustion which converts the water into steam at high temperature and pressure. Within the boiler, the steam and water make their journey through the following; i. Super Heater – The steam generated from the boiler is wet and is therefore passed through a super heater where it is dried and superheated (i.e., the steam temperature increases above that of the boiling point of water) through several stages by the flue gases on their way to the smokestack. This is done mostly to ensure that there are no entrained liquids or moisture entering the turbine stage as liquids travelling at a high speed and temperature can rupture the blade resulting in corrosion. Another advantage of superheating steam is to increase the overall efficiency of the plant where the steam can travel faster, rotating the turbines rapidly and thus generating more electrical output. ii. Reheater - In large power plants, a reheater is used where several turbines often referred to as multi-stage turbines are used. The reheater is basically a superheater that reheats some of the exhausted steam which were diverted when travelling to the condenser to be converted back into water. From the reheater, it reintroduces the superheated steam between the inlets of the lower pressure stage of the turbine. By reheating steam between a high- pressure and low-pressure turbine, it is possible to increase the electrical efficiency of the power plant cycle. iii. Economizer – Before the water is fed into the boiler to be converted into steam, a heat exchanger deriving heat from the flue gases is used to increase the feed water temperature so that it is easier for phase conversion. This device is known as an economizer. iv. Air Pre-heater – The air pre-heater essentially extracts heat from the flue gases to increase the temperature of air that is drawn in from the atmosphere by a forced draft fan before being supplied to the boiler furnace. The component responsible for this is referred to as an air pre-heater. The principal benefits of preheating the air are increased thermal efficiency and increased steam capacity per square meter of the boiler surface. Figure E-E-TRB-9: Schematic Diagram of a Steam Boiler Steam Turbine From the super heater, the superheated steam is fed to a steam turbine through the main valve. As the steam passes over the blades, thermal energy is transferred and converted into mechanical energy. The exhausted steam after transfer is then sent to be condensed by a condenser by means of cold- water circulation within a cooling unit. Alternator The steam turbine is coupled to either a generator or an alternator where it converts mechanical energy of rotation into electrical energy. Typically, both work on the same principle however alternators are more efficient mainly in generating a higher electrical output. From the alternator the electricity induced is passed onto a transformer which is increases the voltage. This voltage is then transmitted through power lines to a grid station for distribution to the city. III. Cooling Unit The cooling unit is responsible for supplying and/or recycling water for steam generation. It contains the following components; Condenser The condenser, as its name suggests, condenses the exhausted steam from the turbine back into water or condensates below atmospheric pressure. This is done to increase the temperature difference and thus pressure difference between the inlet and outlet of the turbine thereby increasing the amount of heat available for conversion to mechanical power. It is also responsible for supplying the boiler with feedwater. Some water may be lost during the steam cycle which is suitably made up from an external source either from a nearby river, lake or from a constructed cooling tower. This water is then transported via a pump to the economizer within the boiler for steam generation. Cooling Tower Hot water coming out from the condenser is discharged at a suitable location near a large body of water usually a river or lake. In the case where the availability of water from the source is not assured or in constant supply throughout the year, cooling towers are used. During the scarcity of water in the river, the hot water from the condenser is channeled to cooling towers where it is cooled and the sent back in the condenser to be reused as feed water for the boiler. IV. Pollution Control Unit This unit is responsible for the control of pollutants in compliance with pollution control regulations. These pollutants are obtained from gas emissions and residual ash from combusting coal. The principal emissions include: Sulfur dioxide (SO2), which contributes to acid rain and respiratory illnesses Nitrogen oxides (NOx), which contribute to smog and respiratory illnesses Particulates, which contribute to smog, haze, and respiratory and lung disease Carbon dioxide (CO2), which is the primary greenhouse gas Mercury and other heavy metals, which have been linked to both neurological and developmental damage in humans and other animals Fly ash and bottom ash, which are residues created from burning coal which when not disposed correctly will lead to pollution in groundwater and rupturing the environment. The pollution control unit contains the following components; Selective Catalytic Reduction The Selective Catalytic Reduction (SCR) is an integrated system that functions to remove NOx from the flue gases thereby releasing harmless by- products. The basic principle of SCR is to inject ammonia gas with air drawn from a blower into the flue gas where the reaction between NOx is decomposed into N2 and H2O vapor. This chemical reaction occurs and is facilitated within a SCR catalytic reactor. Particulate Collector The particulate collector is generally referring to the pollution control device that contains fabric filters to remove the micrometer to sub-micrometer particles and an Electrostatic Precipitator (ESP) that eliminates the dust and smoke that accompanies the flue gases. In most cases, an ESP is used as the first in reducing finer particle emissions. This is done simply by use of induced electrostatic charge. Rows of thin vertical wires followed by a stack of large flat metal plates oriented vertically, with the plates typically spaced about 1 cm to 18 cm apart, depending on the application. The air stream flows horizontally through the spaces between the wires, and then passes through the stack of plates. A large negative voltage is applied between wire and plate. If the applied voltage is high enough, the air stream and thus the particles around the electrodes (i.e., the plates and wires) becomes ionized. The ionized particles, due to the electrostatic force, are diverted towards the grounded plates. Particles build up on the collection plates and are removed from the air stream. Figure E-E-TRB-10: Conceptual diagram of a plate and bar – Electrostatic Precipitator More and more installations also apply a fabric filter to further reduce emissions of dust. Fabric filters utilize fabric filtration to remove particles from the contaminated gas stream by depositing the particles on fabric material. The filter's ability to collect these small particles is due to the accumulated dust cake and not the fabric itself. The filter is usually in the form of cylindrical fabric bags, hence the names "fabric filter" or "bag house", but it may be in the form of cartridges that are constructed of fabric, sintered metal, or porous ceramic. Scrubber The sulfur emissions in the flue gases are reduced from coal combustion before reaching the atmosphere most often by use of pollution control towers, referred to as a scrubber. The scrubber is typically constructed with steel alloys and lined with rubber, tiles, or coatings to protect the tower from the corrosive operating environment. It reduces the SO2 by use of a flue-gas desulfurization (FGD) system based in wet or semi-dry scrubbers. In a wet SGD system within the scrubber employ a mixture of materials, mainly water, limestone or lime, that are sprayed inside a tower to “scrub” or absorb and remove the sulfur from the flue gas. A system of pipes, pumps, and grinders prepares and distributes the limestone mixture, and solid wastes are removed and either taken to a landfill or sold as gypsum for drywall or other materials. In Drier scrubbing systems, chemicals mixed with lesser water are sprayed into a tower to remove sulfur from flue gases. These are less efficient at SO2 removal but require less water and other materials leading to lesser maintenance and operating costs. Direct sorbent injection (DSI) is a newer method of SO2 control in which chemicals are injected directly into the furnace after the SCR. This method is attractive because it eliminates the large costs, materials, and water typically required to construct and operate FGD systems. Interviewees stated that this method generally has unfavorable economics due to the high cost and amounts of the injected chemicals required, but DSI is becoming a more attractive option for units that are required to reduce water use. Smoke-Stack After pollutants are reduced from the coal-combustion process, the remaining and less harmful flue gases exit to the atmosphere through chimney referred to as a smokestack. The smokestack is several hundred meters high and is usually constructed from reinforced concrete and lined with either fiberglass- reinforced polymers, borosilicate blocks, or a nickel-metal alloy. These specialty materials are customized for specific applications and are designed to resist the temperatures and corrosive environments in coal-fired power plant chimneys. Thermal Power Plants: Nuclear Energy – Nuclear Power Plants Nuclear energy generates tremendous amounts of heat which spin steam turbines coupled to a power generator. It has a large range of applications from the typical nuclear power plant generating electricity for homes, propelling submarines and certain aircraft carriers as well as producing isotopes used for medical research for treatment of certain medical diseases. All this harness a different type of nuclear reaction. Essentially, nuclear energy is the energy that is possessed and released in significant amounts from the nucleus of an atom thereby altering the characteristics of it. For this reason, it is sometimes referred to as atomic energy. This energy within the nucleus requires a sustained amount of energy to sufficiently initiate a nuclear reaction which is a process involving a change in the characteristics of an atom induced by firing or bombarding its nucleus with an energetic particle. This energetic particle may either be an alpha particle, a gamma-ray photon, a neutron, or a heavy-ion. In any case, the bombarding particle must have enough energy to approach the positively charged nucleus to within range of the strong nuclear force. Nuclear energy is distinctly released either from the nuclear reaction of fusion or fission. Nuclear fusion involves the combination or merging of two light-weight nuclei to from a single heavier nucleus. This results in a tremendous release of energy where the mass of the newly formed nucleus is lighter than the combined mass of two electrons. This phenomenon was explained by Albert Einstein in his famous mass-energy equivalence equation E = mc2. He stated that mass is also a form of energy. The burning of the sun is great example of nuclear fusion. Nuclear fission is the exact opposite to a nuclear fusion reaction. It involves the subdivision or splitting of a heavier nucleus into two lighter ones, roughly of equal mass. This can be through natural means such as radioactive decay or induced and controlled such as in Nuclear Power plants. Nuclear fission takes place when a large and unstable isotope is bombarded by accelerated sub-atomic particles, usually neutrons. These neutrons are accelerated and then slammed into the unstable isotope, causing it to break into smaller particles. During this process, a neutron is accelerated and strikes the target nucleus which splits the target nucleus and breaks it down into two smaller isotopes (the fission products), three high-speed neutrons, and a large amount of energy. Nuclear power plants use the nuclear fission of uranium (Ur), usually U-235 as its main fuel to generate power. According to the increasing mass of the nuclei of all atoms, uranium is one of the heaviest of all the naturally occurring elements. It is 18.7 times as dense as water. It occurs in several forms as radioactive isotopes particularly in their nucleus which possess a different amount of neutrons. Natural uranium as found in the Earth's crust is a mixture, largely of two radioactive isotopes: uranium-238 (U-238), accounting for 99.3% and uranium-235 (U-235) about 0.7%. Uranium as like all radioactive isotopes decays. U-238 decays very slowly where its half-life is about the same as the age of the Earth (4500 million years). This means that it is barely radioactive, less so than many other isotopes in rocks and sand. Nevertheless, it generates 0.1 Watts/ton as decay heat, and this is enough to warm the Earth's core. U-235 decays slightly faster and under certain controlled conditions can be readily split. It is therefore preferable to use it as fuel in most nuclear reactors. Figure E-E-TRB-11: Uranium Atom (Ur-235 has 143 neutrons; Ur-238 has 146 neutrons) The nucleus of the U-235 atom comprises of 92 protons and 143 neutrons (92 + 143 = 235). When the nucleus of a U-235 atom captures a moving neutron, it splits in two (fissions) and releases some energy in the form of heat. During this process, two or three additional neutrons are thrown off. If enough of these expelled neutrons cause the nuclei of other U- 235 atoms to split, more neutrons will be released subsequently causing a fission chain reaction. When this phenomenon occurs repeatedly over many millions of times, a massive amount of heat is produced from a relatively small amount of uranium. It is this process in which uranium is “burnt” or used up. In a nuclear reactor, the uranium fuel is assembled in such a way that a controlled fission chain reaction can be achieved. The heat created by splitting the U-235 atoms is then used to make steam which spins a turbine to drive a generator, producing electricity. Figure E-E-TRB-12: Fission Reaction In a nuclear power station, the fission of uranium atoms replaces the burning of coal or gas in a coal fired power plant. In this manner, nuclear power stations and fossil-fueled power stations share a similar process of generating electricity. Both require heat to produce steam to drive turbines and generators. There are several different types of nuclear reactors however the most commonly used is the Pressurized Water Reactor (PWR) of which its setup will be discussed. The design of PWRs originated as a submarine power plant. PWRs use ordinary water as both a coolant and moderator. The design is distinguished by having two circuits; a primary circuit which flows through the core of the reactor under very high pressure, and a secondary circuit in which steam is generated to drive the turbine. Provided below in Fig. E-E-TRB-13 is a schematic diagram that illustrates a basic PWR plant setup. Figure E-E-TRB-13: Schematic of a Nuclear Power Plant Main Components in a Nuclear Power Plant I. Engine Unit - Nuclear Reactor Figure E-E-TRB-14: Nuclear fuel pellets (Top left) loaded into fuel rods (Bottom Left) and are assembled into the Nuclear Reactor (Right) The nuclear reactor is referred to as the engine of the nuclear power plant in which the fuel is added into for a controlled and sustained nuclear fission reaction. It is found in the primary circuit of the power plant. Water in the reactor core reaches about 325°C; hence it must be kept under about 150 times the atmospheric pressure to prevent it from boiling. Provided below are its main components. i. Fuel and Fuel Rods The uranium (uranium oxide) that is extracted and refined from its ore is typically in its powder form. It is densely pressed into small ceramic cylinders or pellets of which each pellet is approximately 3/8-inch (9.5mm) in diameter and 5/8-inch (15.9mm) in length. (see fig E-E-TRB-14) Each pellet produces about the same amount of energy as 560L of oil. These pellets are then stacked into thin metal tubes known as a fuel rod. All these rods are then grouped together to form fuel assemblies. Generally, the number of rods used range from 90 to well over 200 and varies depending on the type of reactor and amount of desirable power that is to be generated. ii. Coolant The massive amounts of heat generated are safely controlled using a cooling fluid or coolant. It is circulated through the core of the reactor, dissipating the heat where it is channeled to create steam. Most coolants are typically regular or heavy water. iii. Moderator The fast neutrons emitted from the fission reaction require acceptable speeds for an effective fission chain reaction. The fuel elements are therefore surrounded by a substance called a moderator to slow the speed of the emitted neutrons and thus enable the chain reaction to continue. Water, graphite, and heavy water are used as moderators in different types of reactors. iv. Control Rods These are made with neutron-absorbing material such as cadmium, hafnium or boron, and are inserted or withdrawn from the core to control the rate of reaction. In some reactors, special control rods are used to enable the core to sustain a low level of power efficiently. Depending on the type of nuclear reactor, some rods are inserted either from the bottom or top of the core. (Secondary control systems involve other neutron absorbers, usually boron in the coolant – its concentration can be adjusted over time as the fuel burns up.) PWR control rods are inserted from the top. v. Pressure Vessel or Tubes The pressure vessel is usually a robust steel vessel containing the reactor core and moderator/coolant, but it may be a series of tubes holding the fuel and conveying the coolant through the surrounding moderator. II. Steam and Electricity Generation Unit This unit is similar to that of the coal fired power plant and is located in the secondary circuit of the power plant. It is responsible for the production of steam from heating water. It consists of a steam generator that produces steam and the steam turbine and generator for producing electricity. i. Steam Pressurizer The high pressure from the steam is maintained using a pressurizer. It is a large steel vessel that regulates the primary coolant The secondary shutdown system involves adding boron to the primary circuit. The secondary circuit is under less pressure and the water here boils in the heat exchangers which are thus steam generators. The steam drives the turbine to produce electricity, and is then condensed and returned to the heat exchangers in contact with the primary loop. ii. Steam Generator In the reactor, water at high pressure and temperature removes heat from the core and is transported to a heat exchanger referred to as a steam generator. The heat from the primary loop is transferred to a lower-pressure secondary loop also containing water. The water in the secondary loop enters the steam generator at a pressure and temperature slightly below that is required to initiate boiling. Upon absorbing heat from the primary loop, however, it becomes saturated and ultimately slightly superheated. The steam thus generated ultimately serves as the working fluid in a steam-turbine cycle. iii. Steam Turbine and Generator Much like the coal-fired power plant, the setup of the steam turbine and generators are similar. The high pressurized steam transfers its kinetic energy on the blades of the turbine that rotates the shaft of the generator to produce electricity. The expanded steam is then channeled to the condenser where it is reverted into water and introduced back into the steam generator via a pump where the cycle will be repeated. III. Cooling and Unit The cooling unit is responsible for supplying and/or recycling water for steam generation. It contains the following components; Condenser The condenser is responsible for converting the exhausted steam back into water or condensates below atmospheric pressure. This is done to increase the temperature difference and thus pressure difference between the inlet and outlet of the turbine thereby increasing the amount of heat available for conversion to mechanical power. In addition to the above units mentioned, concrete walls are built that surround mainly the nuclear reactor and steam generator as illustrated in fig. E-E-TRB-13. This structure around the reactor and associated steam generator is designed to protect it from any outside intrusion and to protect those outside from the effects of radiation in from within in case of any serious malfunction inside. It is typically a meter-thick concrete and steel structure. Some modern reactor designs install core melt localization devices or “core catchers” under the pressure vessel to catch any melted core material in the event of a major accident. Hydro-Electric Power Plants Hydro-electric power is a clean, safe, and reliable energy source for generating electricity. A hydro-electric power plant converts the gravitational potential energy of flowing water to drive a turbine that is coupled to an alternator to generate the electricity. The electricity generated then flows to a substation, where a transformer increases the voltage and then distributes it to the end user or fed into the power grid. The modern power plant designs are reasonably mature in reaping significant benefits particularly because it is situated at the junction of two major issues for development; Water and Energy. Hydro reservoirs can often deliver services beyond electricity supply. Hydro storage capacity can mitigate freshwater scarcity by providing security during low flows and drought for drinking water supply, irrigation, and flood control and navigation services. Multipurpose hydropower projects may have an enabling role beyond the electricity sector to secure freshwater availability. (Hydropower Europe , 2019) There are several types of hydropower plants classified on different characteristics however for large-scale hydropower plant, all contain similar working components. Each consists of a reservoir for storage of water, a dam, an intake structure for controlling and regulating the flow of water, a conduit system to carry the water from the intake to the turbines coupled with generators, the draft tube for conveying water from waterwheel to the tailrace, the tailrace and a power house which is the building to contain the turbines, generators, and other accessories to generate, control and supply the electrical energy. This can be illustrated in Fig. E-E-TRB-15; Figure E-E-TRB-15: Basic Diagram of a Hydro-electric Plant Water Reservoir Water reservoirs are a basic requirement for any hydro-electric plant. It is a large body of water that functions to store and supply water during excessive and lean flow periods (i.e., during wet and dry seasons) to maintain a constant supply of water to the turbine thus ensuring a constant power supply. For this reason, hydro-power plants are largely desired as they are to a certain extent not influenced by the weather. I. Forebay There are instances where a forebay is used which can sometimes be the separate reservoir in front of a larger body of water before the water passes through the intake and penstock. Forebays have a number of functions. They are used in flood control to act as a buffer during flooding or storm surges which can replace surge tanks. (See surge tanks). Forebays may also be used upstream of reservoirs to trap sediments and debris to prevent siltation in order to keep the reservoir clean. This entails the use of a man-made structure referred to as a dam built upstream of the main reservoir, called a forebay dam or pre-dam. II. Dam A reservoir can be either natural such as lakes in high mountains or artificial where a dam is built usually across a river. The function of a dam is not only to raise the water surface of the stream to create an artificial head but also to provide the pondage, storage, or the facility of diversion into conduits by use of metallic gates. A dam is the most expensive and important part of a hydro-project. Dams are built of concrete or stone masonry, earth, or rock fill. The type and arrangement of the dam is dependent upon the topography of the site. A masonry dam may be built in a narrow canyon. An earth dam may be best suited for a wide valley. The choice of dam construction also depends upon the foundation conditions, local materials, and transportation available, occurrence of earthquakes and other hazards. III. Spillway To maintain the desired water level in the reservoir or forebay, the discharge is regulated by controlling the degree to which the metallic gates are positioned when opened. This is done by using a spillway. It is a structure that we typically constructed out of reinforced concrete and primarily functions to release surplus waters from the reservoir to prevent overtopping and possible failure of the dam. It mainly arises during flood periods and so acts as a safety gate to discharge the overflowing water to the down-stream side when the reservoir is full. IV. Conduit system The conduit system is basically a system that facilitates the flow of water into the main power plant. The system used is dependent mainly on the topography or location of the hydro-electric plant and the effective head of water (i.e., the height of the drop of water into the hydropower plant) Figure E-E-TRB-16: Types of hydropower plants based on effective head In all cases, all water is directed through an opening referred to as a water intake. This is a structure constructed to channel the flow from the reservoir to either a powerhouse where situated are the turbines or a penstock which are large cylinders usually made of steel or reinforced concrete. Most water intakes generally contain a reinforced and metallic screen known as a trash rack that is directly fitted at the entrance to filter debris such as floating logs, branches and ice. This is to protect the turbine blades from damage of unwanted vibrations either from the ingress of floating material or air bubbles that are formed as a result of low pressure from blockage flow. As such, cleaning devices are fitted to regularly remove debris and keep the screen clear. For medium to high head plants (see fig. E-E-TRB-16), the water flows from the intake down to the penstock. In case of medium head power plants each powerhouse unit is usually provided with its own penstock. In case of high head plants, a single penstock is frequently used, and branch connections are provided at the lower end to supply two or more units. V. Surge Tank There are instances where there will be a sudden change in the flow of water down the pipe mainly due to fluctuations in load for power supply, emergency conditions or accidents and for maintenance work for piping and power equipment. In such cases the gates positioned near the water intake or valves along the piping system are forced to close. This abrupt change causes the water to slam against the blockage and reverse in flow direction. Since water is incompressible, a high-pressure backflow is developed and can rupture the conduit or piping system. This phenomenon is referred to as water hammering and is characterized by a loud banging or knocking sound on the pipes. To avoid this, some means are required to be provided for taking the rejected flow. This may be accomplished by providing a small storage reservoir such as the forebay or a tank (open at the top) for receiving the rejected flow and thus relieving the conduit pipe of excessive water hammer pressure. This storage vessel, called the surge tank is usually located as close to the power station as possible, preferably on ground to reduce the height of the tower. A decrease in load demand causes a rise in water level in the surge tank. This produces a retarding head and reduces the velocity of water in the penstock. The reduction in flow velocity to the desired level makes the water in the tank to fall and rise until damped out by friction. Increase in load on the plant allows more water to flow through the penstock to supply the increased load and there is a tendency to cause a vacuum or a negative pressure in the penstock. This negative pressure in the penstock provides the necessary accelerating force and is objectionable for very long conduits due to difficult turbine regulation. Again, under such conditions, the additional water flows out of the surge tank. As a result, the water level in the surge tank falls, an accelerating head is created and flow of water in the penstock is increased. Thus, surge tank helps in stabilizing the veloc- ity and pressure in the penstock and reduces water hammer and negative pressure or vacuum. The ideal location of a surge tank is at the turbine inlet but in the case of medium and high head power plants, the height of the surge tank will become excessive. Because of this reason, the surge tanks are usually provided at the junction of the pressure tunnel and the penstock. VI. Water Turbine Water turbines are used as prime movers to convert the kinetic energy of water into mechanical energy which is further utilized to drive the alternators generating electrical energy. The water turbines differ depending on the type of hydro-power plant constructed however the most commonly utilized are the Francis turbines. The schematic diagram of a Francis turbine is shown in Figure E-E-TRB-17; Figure E-E-TRB-17: Francis Turbine A Francis turbine consists of mainly of the following; 1. Spiral Casing The turbine is usually fitted along a vertical shaft although some smaller plants use horizontal shaft alignment. In any case, the fluid enters from the penstock to a spiral casing which completely surrounds the runner. This casing is known as a spiral casing or volute. The rate of flow along the fluid path in the volute is decreased as a result coming into contact with the blades or runners. To maintain a steady velocity, the cross-sectional area of this casing decreases uniformly along the circumference (see Fig. E-E-TRB-17). 2. Guide or Stay Vanes The guide or stay vanes convert a part of the pressure energy in water at the entrance to kinetic energy and then direct the fluid on to the runner blades at the angle appropriate to the design. Moreover, the guide vanes are pivoted and can be turned by a suitable governing mechanism to regulate the flow while the load changes. The guide vanes are also known as wicket gates. 3. Runner Blades The runner blades receive the transferrable energy that rotate the shaft. The guide vanes direct the fluid impart a tangential velocity and hence an angular momentum to the water before its entry to the runner. The flow in the runner of a Francis turbine is not purely radial but a combination of radial and tangential. The flow is from the outside towards the center. The main direction of flow change as water passes through the runner and is finally turned into the axial direction while entering the draft tube. 4. Draft Tube The draft tube is a conduit which connects the runner exit to the tail race where the water is being finally discharged from the turbine. The primary function of the draft tube is to reduce the velocity of the discharged water to minimize the loss of kinetic energy at the outlet. This permits the turbine to be set above the tail water without any appreciable drop of available head. A clear understanding of the function of the draft tube in any reaction turbine, in fact, is very important for the purpose of its design. The purpose of providing a draft tube will be better understood if we carefully study the net available head across a reaction turbine VII. Tailrace The water after having done its useful work in the turbine is discharged to the tailrace which may lead it to the same stream or to another. The design and size of the tailrace should be such that water has a free exit and the jet of water, after it leaves the turbine, has unimpeded passage. Activity 2: Power Generation, Transmission and Distribution In an electricity grid, transmission lines carry high voltages from the powerhouse of the plant to a substation in which a transformer increases or decreases the voltage supplied depending on the desired output. This voltage is then sent to power homes via distribution lines. In this activity, power transmission and the distribution of electricity in PNG and its concepts will be covered. Procedure: 1. With the attached reference materials on the information on PNGs Power only electricity company, describe how power is distributed and transmitted. Exercise: 1. Generation Station (Power Stations) A generation station or a power station sometimes known as power plant is responsible for the generation of the electrical energy. Power stations are usually connected to electrical grids or transmission lines. These power stations may contain one or more generators that converts the mechanical power into electrical power. The energy source from which the electrical energy is being converted from varies widely depending on the geographic landscapes. Energy is abundant in PNG. 2. Power Stations in PNG The information below is quoted from the PNG Power Information Handbook-2017. In PNG, PNG Power is responsible for the operation of 30 electricity systems at various centers throughout the country. Three of these systems are hydro based with the generation capacity in excess of 10Megawatts (MW). Thermal or diesel generation run the other smaller systems. PNG Power's main source of electricity generation is hydropower, which accounts for 70% of total electricity production. Light fuel oil production is 14%, while the independent energy producer (IPP) has 16%. The current annual production is over 800 GW. Demand for electricity has grown by an average of 2.2% over the past decade, and this trend is expected to continue over the medium term. PNG Power's installed generation capacity exceeds 300 MW. Of these, hydropower plants represent 70% and thermal plants 30% of the total capacity. The transmission voltages are 132 kV, 66 kV and 33 kV and the distribution voltages are 11 kV and 22 kV. Typical voltages are 415 V and 240 V at 50 Hz PORT MORESBY SYSTEM The Port Moresby system serves the National Capital District, the commercial, industrial and administrative center of Papua New Guinea. The Port Moresby system also serves surrounding areas in the Central Province. The main source of generation is the Rouna system consisting of four hydro stations on the Laloki River, controlled water storage in the Sirinumu Reservoir and a small generating set at the toe of Sirinumu dam. The total generation capacity from the Rouna Power Stations is 63MW. PNG Power also operates a thermal power station at Moitaka, outside of Port Moresby which is used to supplement the supply from the Rouna Power Station and has a generation capacity of 30MW. PNG Power also purchases electricity from privately owned power stations at Kanudi and at the LNG Gas Plant. The Posco Daewoo Kanudi Power Station (24MW) and the ExxonMobil LNG Gas Plant Power Station (26MW) sell power to PNG Power Ltd and add 60 MW to the Port Moresby system generation capacity and is being utilized as base load. The load demand for the Port Moresby System is 158MW in 2016. PNG Power also owns the Kanudi Power Station which consists of Gas Turbines. The Port Moresby hydro generation is transported by three 66kV lines and a 33kV feeder and the Moitaka thermal generation output is delivered to the system at Boroko via a single and a double circuit 66kV line which also supplies the Waigani Substation. There is also a 66kV circuit line from Moitaka to Kanudi to improve the security of power supply. RAMU SYSTEM The main source of generation is the Ramu Hydro Power Station with an installed capacity of 75MW, comprising of five units of 15MW each. This station which was previously a run-of- river scheme became a storage scheme when the Yonki dam was commissioned in February, 1991. Additional hydro energy is supplied by Pauanda, a run-of-river station in the Western Highlands Province with 12MW. Power is also purchased when required from the privately owned Baiune Hydro Power Station at Bulolo, Morobe Province and varies between 1-2MW depending on availability. Transmission line outages, energy and peak demands are met by diesel plants at Madang, Lae, Mendi, Wabag, Kundiawa and Goroka. These plants serve as stand-by units. Three radial lines originating from Ramu Hydro Power Station switchyard serve Lae, Madang and the Highlands centers. Currently Madang and the Highlands Region are supplied at 66kV and Lae at 132kV. The Highlands line interconnects with Pauanda hydro station and supplies the townships of Kainantu, Goroka, Kundiawa, Mt Hagen, Wabag and Mendi. GAZELLE PENINSULA SYSTEM The Gazelle Peninsula system serves the townships of Rabaul, Kokopo and Keravat to service Gazelle’s economy based on copra, coconut oil, cocoa, timber and fishing. The Gazelle Peninsula system is powered by a 10MW hydro power system at Warangoi, Ulagunan Diesel Power Station with 8.4MW, and 0.5MW from Keravat Diesel Power Station. A 66kV line linking Warangoi to the Rabaul, Kokopo and Keravat zone substations delivers hydro power from Warangoi Power Station to these load centers. OTHER CENTRES The Kimbe system is supplied by the Ru Creek Mini hydro system and the Bialla system by the Lake Hargy mini hydro scheme, while the other centers are served by diesel generating stations. PNG Power continues to take over the supply of electricity to district centers as its Rural Electrification program expands to connect these centers. In your respective provinces or villages, suggest what type of energy generation station you think would be capable of setting up and why do you think this is so? 3. Power Transmission & storage From generation or the power station, electrical power produced is then transmitted to different locations where it is applied to carry out useful work. This is where the voltage is transformed and is distributed to consumers or other substations. Electrical power transmission lines generally known as the power grid is formed by the interconnected lined that enable the movement of electrical energy. Power itself is defined as the ability or capacity to do work. It is defined as a unit of energy per unit time. 𝑃𝑜𝑤𝑒𝑟 = 𝑤𝑜𝑟𝑘 𝑑𝑜𝑛𝑒/𝑡𝑖𝑚𝑒 𝑡𝑎𝑘𝑒𝑛 𝑜𝑟 𝑃 = 𝑤/𝑡 𝑚𝑒𝑎𝑠𝑢𝑟𝑒 𝑖𝑛 𝑤𝑎𝑡𝑡𝑠 Power transmission is associated most usually with the electric power transmission with the widespread establishment of electrical grids. Here, power generated is moved over long distances to the respective loads without power loss. Electricity itself cannot be stored on any scale hence, can only be converted to other forms for later use. Electricity storage at times of low demand and high electricity production aids fluctuations in electricity supply and demand and then release it back to the electric power grid during low production or high demand periods. These storage systems include the battery, flywheel, compressed air and pumped hydro storage. Any systems are limited to the maximum amount of energy it stores. The chief role of grid-scale batteries nowadays may be designed to provide ancillary services including frequency control to a transmission system. For example, Ramu system; the main source of generation is the Ramu hydro power station with an installed capacity of 75MW, comprising of five units of 15MW each. Additional hydro energy is supplied by Pauanda hydro power station in the Western Highlands province with a total of 12MW. 4. Primary and Secondary transmission. This generated power is stepped up using a transformer to a voltage level before being sent to the distribution centers via transmissions line. Stepping up or down the voltage depends on the distance that it needs to be transmitted; the longer the distance, the higher the voltage. Power loss occurs when it is being transmitted hence, stepping up these voltages to desired levels helps reduces power loss makes it more efficient. The transfer of large quantity of electrical power from initial generating stations to the substations through overhead transmission lines is known as the primary transmission. Underground cables are also used in some cases where transmission takes place over a shorter distance in some other countries While on the other hand, when electrical power transmitted reaches a receiving station, the voltage is stepped back down to a voltage typically between 33kV and 66kV. It is then sent to transmission lines emerging from this receiving station to electrical substations closer to “load centers” such as cities, villages, and urban areas. This process is known as secondary transmission. When electrical power reaches a substation, it is stepped down once more by a step-down transformer to voltages closer to what it was generated at—usually around 11kV. From here, the transmission phase graduates to the distribution phase, and electrical power is used to meet demand from primary and secondary consumers. Research Question With the aid of provided information, and research on the PNG Power transmission in PNG, explain how electricity is transmitted in PNG. 5. Power Distribution Electric power distribution is the final stage in the process of electricity where the delivery of the electric power from the transmission system to each individual consumer. Distributing and retailing of electricity in PNG is provided by PNG Power. Primary distribution lines to distribution located near customer’s buildings are lines that carry the medium voltage power. Here, the voltage is stepped down again to utilization voltage by distribution transformer to be used for lighting purposes, industrial equipment and household appliances. Secondary distribution lines are often used for distributing of electricity to consumers. These consumers are connected to the secondary lines maybe commercial or residential. Customers that usually require a huge amount to power are often connected to the primary distribution levels. This transition of from the transmission to distribution occurs in the substations. Modern distribution systems are heavily integrated with renewable energy sources thus resulting this in becoming more independent from transmission networks. It is challenging at times where there is a need for balancing the supply and the demand that requires the use of various technological such as battery storage power systems and data analytics. Substations transform voltage from high to low or vice versa depending on its needs. The substation contains the circuit breakers, transformers and bus bar. Circuit breakers helps prevent damages to electrical circuits from excess current or a short circuit. Thus it enables the substation to the substation to disconnect from transmission grid or distribution lines. Transformers step down or up the voltage as per the type of distribution transformer. From there, power splits between multiple directions as it goes to the bus bar. Figure E-E-TRB-18: System Overview THEMA: BATTERY TECHNOLOGY INTRODUCTION The development of battery technology has progressed in an unpredictable manner since its emergence and popularized use in the late 19th Century. Batteries were a predominant source of electricity before the invention of Alternating Current (AC) in electric generators and grid stations that pressed a transitional shift because of a need for a controlled and regulated large- scale power supply. However so, the stored potential of this electro-chemical device received significant breakthroughs from the rise of rise of telegraph that enticed scientists and engineers to not be oblivious to its stored potential for the future. The use of mobile phones and computers are some noteworthy examples of battery technology’s constantly evolving application. In Engineering, it is paramount to understand the role and design of battery technology in the modern world. As battery designs over the years have transitioned from a very large, rigid structure to a smaller and densely packed arrangement, the future of this technology has greatly revolutionized certain industries such as transportation of which battery-powered vehicles now compete with combustion heat engines. Because of its portable power supply and recharge- ability - particularly for secondary batteries - this field of technology has become a favorable incipient for innovative applications in electric devices traversing in almost all fields of profession. MODULE 1: CONNECTING BATTTERIES In this module, the fundamentals of battery technology; its history, electrostatic forces and the basic working principle of batteries will be discussed with the aim to insight students on its significant impact on society. In particular, lithium-ion batteries will be discussed to concretely explain how much batteries can become a game changer in the modern technological age. TOPIC 1: INTRODUCTION TO BATTERIES Batteries provide a portable power supply for almost all our everyday appliance from flashlights, phones, cameras, and the current advent of electric vehicle production. This topic discusses a brief history of its invention and introduces students to its basic structure and working principle. Take Home Messages: Batteries are a type of energy storage device which utilize chemical reactions that are converted to electrical energy to power electrical devices. All batteries are made up of two primary components: the electrodes (anode and cathode) and an electrolyte. Reduction-oxidation (Redox) reactions occur simultaneously where if a load is connected to the battery, the anode undergoes oxidation and the cathode undergoes reduction. The battery is discharged or used when a pathway is created for the electrons and ions to flow from the anode and cathode. Activity 1: Invention and Working principle of batteries In this activity, students will appreciate the invention of batteries and understand its working principle that can be applied to any type of battery. This will enable students to grasp a definitive outlook of the battery as an important and portable power supply. Procedure: 1. With the attached reference materials and background research, clearly define battery and its related concepts. 2. State and explicitly describe the components and fundamental working principle of a battery. Exercise: Below contains information on the Introduction of Batteries that correspond to questions in the SRB. 1. Definition of the term “Battery” A Battery is a type of energy storage device or power source with an external connection for powering electrical devices. Given below are some definitions for batteries; o “Batteries are a collection of one or more cells whose chemical reactions create a flow of electrons in a circuit.” (Editors of Sparkfun, n.d.) o “A battery is a device that stores chemical energy and converts it to electrical energy. The chemical reactions in a battery involve the flow of electrons from one material (electrode) to another, through an external circuit.” (Editors of Australian Academy of Science, 2016) o “A battery is a device that converts chemical energy contained within active materials directly into electrical energy by means of an electrochemical oxidation-reduction (redox) reaction. This type of reaction involves the transfer of electrons from one material to another via an electric circuit. “ (What is a battery, n.d.) The term battery is often used but actually refers to a combination of one or more electrochemical cells or units that are connected either in series or parallel depending on the desired output voltage and capacity. 2. Invention of the Battery The invention of the battery is credited to the Italian scientist Alessandro Volta, who actually developed it to prove a point to his colleague Luigi Galvani who was also an Italian scientist. In the late 18th Century, Galvani had conducted an experiment that showed how if the legs of a dead frog were suspended on a brass hook, it would twitch when in contact with a probe (iron scalpel) of some other type of metal. He believed this was caused by electricity from within the frog’s tissues and called it “animal electricity”. Figure E-BT-TRB-a) Galvani's deceased frog experiment; b) Volta's pile experiment Volta, while initially impressed with Galvani’s findings, came to believe that the electrical current came from the two different types of metals (i.e., the hooks that the deceased frog was hanging on and the metal probe) and was merely transmitted through, not from the frog’s tissues. He experimented with stacking layers of silver and zinc interspersed with layers of cloth or paper soaked in brine (saltwater), and found that an electrical current did in fact flow through a wire applied to both ends of the pile. Volta also found out that by using different metals in the pile, the amount of voltage could be increased. His invention earned him sustained recognition in the honor of the “Volt” (a measure of electrical potential) being named after him. 3. Main Components of an Electrochemical Cell There are many different batteries that diverge into different applications based on their design and construction, however all are fundamentally electrochemical cells that possess a comparable working principle. Essentially, a closed external circuit is allowed to facilitate the flow of electrons initiated by a chemical reaction occurring at an electrode and flows over to the other electrode. This can easily be explained by firstly identifying the main cell components as provided below; Figure E-BT-TRB-2: Main components of an Electrochemical Cell I. Electrodes Electrodes are solid conductors through which electricity enters and leaves a component in a circuit and are made of different types of metals. Electrons typically flow from a negatively charged region to a positively charged one depicted by the positive and negative signs on the circuit in Fig. E-BT-TRB-2. For this reason, there are two types of electrodes used; the Anode and Cathode. Any two metals can be used as electrodes as long as there is a standard potential between them, which is the difference between the electrical voltage or energy obtained when it undergoes either oxidation or reduction reaction (this is explained in the cell’s working principle). Ideally, the greater the voltage potential, the greater the energy obtained. This is referred to as the electrochemical potential and involves the average energy of the outermost electrons of the molecule or elements in its two valence states (i.e., the anion and cation). Simply put, electrochemical potential is the tendency of the metals to lose electrons. A series of electrodes (i.e., metals used as electrodes) are arranged in increasing standard oxidizing potentials or in decreasing reduction potential. This series is referred to as the electrochemical potential series or activity series. Anode In a battery, the anode is the negative terminal or electrode. It releases or loses electrons to the external circuit during the electrochemical reaction. Some materials used include metals such as zinc (Zn) and Sodium (Na) as the anode. Some anodes are inert and do not react or participate in the reaction such as graphite however allow the flow of the electrons lost in the salt solutions. Cathode In a battery, the cathode is the positive terminal or electrode. It acquires or gains electrons from the external circuit from the anode during the electrochemical reaction. Some materials used are metals such as copper, semiconductors and even conductive polymers. Metallic Oxides are often used as cathodes in some batteries such as Lithium Cobalt Oxide (Lithium Cobaltate) or Lithium Manganese Oxide (Lithium Manganate). II. Electrolyte The electrolyte is the medium that enables the battery to be conductive by providing an ion transport mechanism between the anode and cathode of a cell. They are often thought of as liquids, such as water or other solvents with dissolved salts, acids or alkalis that are required for ionic conduction. It should however be noted that many batteries including the conventional AA or AAA batteries contain solid electrolytes that act as ionic conductors at room temperature. In terms of batteries, other essential components include a; o Separator Separators are porous materials that prevent physical contact between the anode and cathode, which would cause a short circuit in the battery leading to fires. It also enables the transportation of ions in the cell. Ions in the electrolyte can be either positively charged or negatively charged and c