Endocrine System PDF
Document Details
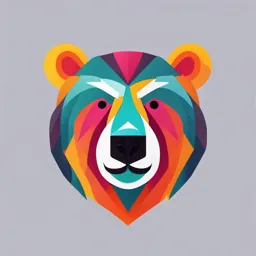
Uploaded by WellBacklitAppleTree
Margaret A. Miller
Tags
Summary
This document provides a detailed overview of the endocrine system, encompassing its structure, function, and associated diseases. It explains the mechanisms of endocrine signaling and the roles of various endocrine glands and tissues. The text extensively covers the various processes within the system and includes discussions of different diseases impacting domestic animals.
Full Transcript
CH APTER 12 Endocrine Systema Margaret A. Miller Key Readings Index Structure and Function, 767 Dysfunction/Responses to Injury, 777 Portals of Entry/Pathways of Spread, 779 Defense Mechanisms/Barrier Systems, 779 Diseases Affecting Multiple Species of Domestic Animals, 780 Diseases of Horses, 799...
CH APTER 12 Endocrine Systema Margaret A. Miller Key Readings Index Structure and Function, 767 Dysfunction/Responses to Injury, 777 Portals of Entry/Pathways of Spread, 779 Defense Mechanisms/Barrier Systems, 779 Diseases Affecting Multiple Species of Domestic Animals, 780 Diseases of Horses, 799 Diseases of Ruminants (Cattle, Sheep, and Goats), 800 Structure and Function Endocrine glands release their secretions (hormones) directly into blood vessels; thus, unlike exocrine glands, they do not need (or have) a ductal system. In endocrine signaling (Fig. 12.1), the hormones released into the bloodstream bind to specific receptors on target cells at distant sites. Steroid hormones are lipid soluble and can cross the plasma membrane of a cell to activate intracellular receptors (e.g., transcription factors that bind nuclear DNA), whereas polypeptide hormones or catecholamines signal through cell surface receptors, such as receptor tyrosine kinases (RTKs). Major endocrine glands, such as the pituitary gland, thyroid gland, and adrenal glands, are composed of cells of diverse origin, but they function as dedicated endocrine organs. Endocrine tissue can also exist as individual or aggregated endocrine cells within another organ that has other functions, including nonendocrine functions. For example, the pancreatic islets are discrete collections of endocrine cells that form only a small portion of the pancreas. In addition, many organs or tissues that are not generally included in the endocrine system, such as lung, liver, skin, and gastrointestinal tract, contain dispersed endocrine cells. Other cells that might not be considered endocrine at first glance, such as adipocytes, in addition to their principal functions, synthesize and secrete chemicals into the bloodstream with a hormonal effect on distant cells and tissues. Because endocrine cells require proximity to the vasculature, endocrine tissue, with the exception of thyroid follicles, is generally arranged as cords or packets of cells in scanty fibrous stroma that is well vascularized by sinusoids or capillaries. Ultrastructurally, endocrine cells that synthesize polypeptide hormones or catecholamines have a prominent rough endoplasmic reticulum (ER), a well-developed Golgi apparatus, and cytoplasmic secretory granules (E-Fig. 12.1). At the light microscopic level, the cytoplasm appears eosinophilic and faintly granular. The secretory granules are variably immunoreactive with antibodies to chromogranins, synaptophysin, and protein gene product (PGP) 9.5, so these immunohistochemical markers can be used to identify so-called “neuroendocrine” cells. aFor a glossary of abbreviations and terms used in this chapter, see E-Glossary 12.1. Diseases of Pigs, 801 Diseases of Dogs, 801 Diseases of Cats, 805 In contrast, endocrine cells that synthesize steroid hormones have abundant smooth ER and cytoplasmic lipid bodies that contain cholesterol and other precursor compounds. Histologically, steroid hormone-producing cells have abundant lipid-vacuolated cytoplasm. Somewhat surprisingly and inexplicably, immunohistochemistry for the melanocytic marker, Melan-A, can be used to label cells that produce steroid hormones. Endocrine glands are subject to all forms of injury and respond by degeneration or cell death, inflammation, vascular disturbances, or disturbances of growth. The endocrine glands are particularly prone to atrophy or proliferation (hyperplasia or neoplasia). These disturbances of growth are often the basis for endocrine dysfunction. Hypofunction of an endocrine gland refers to insufficient production or release of its hormone(s). Primary hypofunction is the result of a biochemical defect in hormone synthesis or the result of either failure of development or destruction of the secretory cells. Hypofunction is considered secondary if the cause occurs outside the hypofunctioning gland; for example, if the pituitary gland fails to release sufficient adrenocorticotrophic hormone, the resultant adrenocortical hypofunction is secondary. Hyperfunction implies excessive hormone production, and it is considered primary if cells of the endocrine gland autonomously produce and secrete the excess hormone. This result is usually the case in functional neoplasms (i.e., neoplasms composed of cells that continue to produce their hormonal products). Hyperfunction is considered secondary if the excessive hormone production is in response to a signal (e.g., one of the pituitary trophic hormones) from outside the hyperfunctioning gland and, as an example, can occur with neoplasms of the pituitary gland that secrete a specific type of trophic hormone (see Structure and Function, Pituitary Gland [Hypophysis], Adenohypophysis). Endocrine dysfunction can also result from (1) failure of target cells to respond to hormones, either through defective receptors or through adenyl cyclase (second messenger system); (2) systemic disease or metabolic disturbances; or (3) administration of exogenous hormones. Pituitary Gland (Hypophysis) The pituitary gland (adenohypophysis [anterior pituitary gland] and neurohypophysis [posterior pituitary gland]) is situated ventral to the hypothalamus and just caudal to the optic chiasm (Fig. 12.2). 767 CHAPTER 12 Endocrine System 767.e1 E-Glossary 12.1 Glossary of Abbreviations and Terms Adrenocorticotrophic hormone Antidiuretic hormone Adenosine triphosphate Cyclic adenosine monophosphate Corticotrophin-like intermediate peptide Corticotrophin-releasing hormone Member of the cytochrome P450 family of microsomal enzymes Diiodotyrosine Deoxyribonucleic acid Dual oxidase of thyroid hormone synthesis Endoplasmic reticulum Growth hormone (also known as somatotrophic hormone) Humoral hypercalcemia of malignancy Islet amyloid polypeptide (also known as amylin) Insulin-like growth factor Immunoglobulin G Multiple endocrine neoplasia Monoiodotyrosine Messenger ribonucleic acid Melanocyte-stimulating hormone Nonesterified fatty acids Na+/I− symporter o,p′-dichlorodiphenyldichloroethane (also known as mitotane) ACTH ADH ATP cAMP CLIP CRH CYP DIT DNA DUOX ER GH HHM IAPP IGF IgG MEN MIT mRNA MSH NEFA NIS o,p′-DDD GA NA C T T S ER NA S L GA NF E-Figure 12.1 Pituitary Gland, Pars Distalis, Normal Dog. Follicular cells (NF) in the pars distalis form a framework and extend cytoplasmic processes (arrows) around extracellular accumulations of colloid (C). Adjacent follicular cells are joined by prominent terminal bars (T). Acidophils in the storage phase of the secretory cycle contain numerous large, uniformly electrondense secretory granules (S), scattered lipofuscin (L) bodies, a small amount of endoplasmic reticulum (ER), and a small Golgi apparatus. Hypertrophied acidophils (NA) have few mature secretory granules but many distended profiles of endoplasmic reticulum and large Golgi apparatuses (GA) associated with prosecretory granules in the process of formation. Transmission electron microscopy (TEM). Uranyl acetate and lead citrate stain. (Courtesy Dr. C. Capen, College of Veterinary Medicine, The Ohio State University.) PAS Pax PCR PGE PGF PGP PKC POMC PP cells PPID PTH PTHrP RET protooncogene RNA RTK SSTR STH T3 T4 TNF TRH TSH TTF Periodic acid-Schiff Paired box gene Polymerase chain reaction Prostaglandin E Prostaglandin F Protein gene product Phosphokinase C Proopiomelanocortin Pancreatic polypeptide-secreting cells Pituitary pars intermedia dysfunction Parathyroid hormone Parathyroid hormone-related peptide “Rearranged during transfection” protooncogene Ribonucleic acid Receptor tyrosine kinases Somatostatin receptor Somatotrophic hormone (also known as growth hormone) Triiodothyronine Tetraiodothyronine (thyroxine) Tumor necrosis factor Thyrotropin-releasing hormone Thyroid-stimulating hormone Thyroid transcription factor 768 SECTION II Pathology of Organ Systems Endocrine signaling (example: thyroid stimulating hormone) Signaling molecule in vesicle (e.g., hormone or chemical messenger) Circulatory system Receptors occur on a different type of target cell located distant (i.e., systemically) from the cells secreting the signaling molecules Autocrine signaling (example: interleukin-1 in monocytes) Receptors occur on the plasma membrane of the same type of cell that secretes the signaling molecules Paracrine signaling (example: fibroblast growth factor family) Plasma membrane receptor Receptors occur on a different type of target cell located near the cells secreting the signaling molecules Intracrine signaling (example: steroid hormones) Receptors occur on the nuclear envelope of the cell that synthesized or internalized the signaling molecules Nuclear envelope receptor Figure 12.1 Endocrine and Other Cell Signaling Pathways. In endocrine signaling, hormones are released into the bloodstream and bind to receptors on distant target cells to exert their effect. In intracrine signaling, internalized or self-generated signaling molecules that remain within the cell (e.g., steroid hormones or angiotensin II) act by binding to nuclear receptors of the same cell. In autocrine signaling, secretory products act on the same type of cell that synthesized them. In paracrine signaling, secreted molecules act on neighboring cells. (Courtesy Dr. M.A. Miller, College of Veterinary Medicine, Purdue University; and Dr. J.F. Zachary, College of Veterinary Medicine, University of Illinois.) Adenohypophysis The adenohypophysis consists of the pars intermedia and the pars distalis. The pars intermedia surrounds the residual Rathke’s pouch and separates the pars nervosa (see Structure and Function, Pituitary Gland [Hypophysis], Neurohypophysis [Posterior Pituitary Gland]) from the pars distalis. Melanotrophs are the predominant cell of the pars intermedia. They synthesize proopiomelanocortin (POMC), which is cleaved first into adrenocorticotrophic hormone (ACTH) and then into α-melanocyte-stimulating hormone (MSH), β-endorphin, and corticotrophin-like intermediate peptide (CLIP). Although melanotrophs can produce bioactive ACTH, they do not express glucocorticoid receptors, so they generally do not respond to negative feedback from cortisol concentrations in the peripheral blood. Instead, they are controlled (down-regulated) by dopamine released from hypothalamic neurons. The pars intermedia is a common site of hyperplasia and neoplasia in older horses and somewhat less so in dogs and cats. The pars distalis (called pars anterior in the horse) consists of cells that produce, store, and release trophic hormones in response to specific releasing hormones or inhibitory factors from the hypothalamus (see Fig. 12.2, C). The hypophyseal trophic hormones act on targeted endocrine and nonendocrine cells in other organs and tissues. Importantly, hormone production in response to trophic hormone secretion from the adenohypophysis provides negative feedback in the hypothalamic-pituitary-target organ axis (Fig. 12.3). Nevertheless, some endocrine glands or cells—e.g., thyroid medullary C cells, parathyroid chief cells, and chromaffin cells of the adrenal medulla—are not under the influence of pituitary trophic hormones and are not regulated by a hypothalamic-hypophysealtarget organ axis. The trophic hormones produced by pars distalis cells include ACTH produced by corticotrophs, growth hormone (GH) (also known as somatotrophin [STH]) produced by somatotrophs, prolactin produced by lactotrophs, thyroid-stimulating hormone produced by thyrotrophs, and follicle-stimulating hormone and luteinizing hormone produced by gonadotrophs. The gonadotrophic hormones are also addressed in Chapter 18, Female Reproductive System and Mammae and Chapter 19, Male Reproductive System. Historically, trophic CHAPTER 12 Endocrine System 769 h o n d A Neurosecretory neurons (hypothalamic neurohypophyseal releasing hormones) Neurosecretory neurons (hypothalamic adenohypophyseal releasing hormones) Hypothalamus 3 1 Superior hypophyseal artery Sella turcica B Optic chiasm Neurohypophysis (posterior pituitary) Capillaries of the posterior pituitary (portal vascular system) Adenohypophysis (anterior pituitary) Sphenoid bone Hypophyseal vein 2 C Secretory cells of the adenohypophysis Capillaries of the anterior pituitary (portal vascular system) 4 Site of discharge of releasing hormones into capillaries Site of discharge of releasing hormones into capillaries Figure 12.2 Pituitary Gland and Hypothalamus. A, Longitudinal section through the brain of a normal dog, illustrating the close relationship of the pituitary gland to the optic chiasm (o) and hypothalamus (h). The pars distalis (d) forms a major part of the adenohypophysis and completely surrounds the pars nervosa (n, posterior pituitary gland). The residual lumen of Rathke’s pouch (arrow) separates the pars distalis and pars nervosa, and it is surrounded by the pars intermedia. B, Schematic of the hypothalamic-pituitary regulatory axis for the neurohypophysis. Hormones synthesized in supraoptic and paraventricular nuclei of the hypothalamus (1) are transported by axons to the neurohypophysis for storage and release into the blood (2). C, Schematic of the hypothalamic-pituitary regulatory axis for the adenohypophysis. Releasing hormones or inhibitory factors synthesized in the hypothalamus (3) are transported hematogenously to the adenohypophyseal pars distalis, where they regulate synthesis and secretion of trophic hormones into the hypophyseal portal vasculature (4). (A courtesy Dr. C. Capen, College of Veterinary Medicine, The Ohio State University. B and C courtesy Dr. M.A. Miller, College of Veterinary Medicine, Purdue University; and Dr. J.F. Zachary, College of Veterinary Medicine, University of Illinois.) hormone-producing cells of the adenohypophysis were classified by their tinctorial characteristics with specialized histochemistry techniques as acidophils, basophils, or chromophobes. With hematoxylin and eosin (H&E) stain (Fig. 12.4 and E-Fig. 12.2), the cytoplasmic granules of an acidophil are intensely eosinophilic. A basophil has fine basophilic granules. Chromophobes are sparsely granulated without differential staining of the granules in comparison to the rest of the cytoplasm; thus, the granules are difficult to perceive in an H&Estained section. Most of the cells in the pars distalis are corticotrophs (basophils or chromophobes) or somatotrophs (acidophils). Because not all basophils or chromophobic cells are corticotrophs, and not all acidophils are somatotrophs, immunohistochemistry is used to identify the trophic hormone produced by a particular adenohypophyseal cell. Molecular techniques can be used to detect mRNA in cases in which a cell has the genetic machinery to make a particular trophic hormone but does not (1) produce it in sufficient quantity for immunohistochemical detection or (2) release sufficient bioactive hormone into the circulation for detection by plasma assay. Each type of trophic hormone-producing cell in the pars distalis is under the control of a specific releasing hormone or factor from the hypothalamus (see Fig. 12.3). These releasing hormones are small peptides synthesized and secreted by hypothalamic neurons and transported by axons to the median eminence at the base of the third ventricle, where they are released into the hypothalamichypophyseal portal system. Importantly, the median eminence and the pituitary gland are not encumbered with a blood-brain barrier. Each releasing hormone or factor stimulates the rapid release of secretory granules containing preformed trophic hormone from the CHAPTER 12 Endocrine System E-Figure 12.2 Pars Distalis, Normal Dog. The pars distalis is composed of acidophils (white arrows), basophils (none shown here), and chromophobes (white arrowheads). Hematoxylin and eosin (H&E) stain. (Courtesy Dr. J.F. Zachary, College of Veterinary Medicine, University of Illinois.) 769.e1 770 SECTION II Pathology of Organ Systems Hypothalamus (CNS) Releasing hormones Inhibitory factors Adenohypophysis (anterior pituitary gland) Target gland hormones A Trophic hormones Target endocrine glands Trophic (stimulatory) actions Inhibitory actions Figure 12.3 Hypothalamic-Pituitary-Target Gland Axis. Releasing hormones (or inhibitory factors) produced in the hypothalamus act on the adenohypophysis to stimulate (or inhibit) release of trophic hormones. Trophic hormones act on specific endocrine glands, stimulating them in turn to produce hormones that exert their ultimate actions on downstream tissues and also provide negative feedback to the adenohypophysis and hypothalamus. (Courtesy Dr. M.A. Miller, College of Veterinary Medicine, Purdue University; and Dr. J.F. Zachary, College of Veterinary Medicine, University of Illinois.) corresponding adenohypophyseal cells. For example, corticotrophs respond to corticotrophin-releasing hormone with increased production of POMC, which undergoes posttranslational proteolysis to form ACTH, β-lipotropin, and β-endorphin, among other products. Corticotrophs express receptors for glucocorticoids; therefore, they respond to negative feedback from cortisol production by adrenocortical cells. Neurohypophysis The neurohypophysis contains the pars nervosa, which is connected to the hypothalamus by its infundibular stalk and consists mainly of axonal projections from hypothalamic neurons. Hypothalamic neurons in the supraoptic and paraventricular nuclei synthesize the neurohypophyseal hormones oxytocin and antidiuretic hormone (ADH). These nonapeptide hormones are packaged with a corresponding binding protein into membrane-bound neurosecretory granules and transported by axons to the pars nervosa for storage and secretion into the blood (see Fig. 12.2, B). Thyroid Gland In most domestic mammals, the thyroid gland has two distinct lobes, connected by a narrow or almost imperceptible isthmus, and is closely associated with the sides of the trachea, just caudal to the larynx. The word thyroid comes from the Greek word for shieldlike, but among the domestic mammals, the bovine thyroid gland comes B Figure 12.4 Acidophils, Basophils, and Chromophobes in the Pituitary Pars Distalis, Normal Dogs. A, In this dog, the pars distalis is composed of acidophils (arrows) and basophils (arrowheads). Hematoxylin and eosin (H&E) stain. B, In a different dog, the pars distalis is composed of acidophils (arrows) and sparsely granulated chromophobes (arrowheads). H&E stain. (Courtesy Dr. M.A. Miller, College of Veterinary Medicine, Purdue University.) closest to a shield shape with its more prominent isthmus and flattened lobes. The porcine thyroid gland lacks distinct lobes and is centered over the ventral aspect of the trachea, closer to the thoracic inlet than to the larynx. Depending on its blood supply, the thyroid gland is brown (low vascularity) to dark red (high vascularity). Its color can match that of skeletal muscle, with which it can be confused at surgery, autopsy (syn: necropsy) (E-Appendix 12.1), or indeed during carcass trimming at the slaughterhouse, where inadvertent inclusion of bovine thyroid gland in ground beef has resulted in elevated blood thyroxine concentrations in those who consumed even well-cooked hamburgers. The thyroid gland has a unique, among endocrine glands, follicular organization and contains two types of endocrine cells (Fig. 12.5, A and B): (1) follicular cells of endodermal origin that produce the thyroid hormones and (2) parafollicular medullary or C cells of neural crest origin that produce calcitonin. The thyroid gland originates early in gestation from a ventral midline proliferation of endodermal cells between the first and second pharyngeal pouches. Initially, it is connected to the developing tongue by the thyroglossal duct, which mostly disappears later in gestation. Thyroid medullary or C cells are derived from neural crest cells that populate the ultimobranchial body, which fuses with the thyroid gland. Later in gestation, the thyroid gland descends to its adult location near the larynx in most species. CHAPTER 12 Endocrine System E-Appendix 12.1 Autopsy (Syn: Necropsy) Techniques Postmortem Examination of the Endocrine System At autopsy, evaluation of the endocrine system generally includes gross examination of the pituitary gland, pineal gland (especially in horses, in which it is prominent), thyroid gland, parathyroid glands, and adrenal glands. Because many of the endocrine glands are relatively small, it is wise to collect them early in the dissection of the pertinent part of the body. For example, the thyroid and parathyroid glands should be identified before the larynx and trachea are removed from the neck. Likewise, the adrenal glands should be identified before the kidneys have been removed or the vena cava has been cut. Because of the small size of most endocrine organs, the entire organ is often collected for formalin fixation; depending on the diameter or thickness of the organ, it can be fixed with or without prior sectioning. Pancreatic islets are seldom grossly visible, so they are evaluated in histologic sections of exocrine pancreas (see Chapter 8, Hepatobiliary System and Exocrine Pancreas). Likewise, the dispersed endocrine system is not grossly visible and often needs immunohistochemistry (e.g., for generic neuroendocrine markers such as protein gene product 9.5) for histologic identification. Chemoreceptor organs are typically evaluated at autopsy only if they are enlarged. Gonads are another endocrine gland that, if present, should be examined at autopsy, but the ovaries and testes are covered separately under the Reproductive System (see Chapter 18, Female Reproductive System and Mammae and Chapter 19, Male Reproductive System). Endocrine tissues can be fixed routinely (as for other tissues) by immersion in 10% neutral-buffered formalin for histopathologic evaluation. A formalin to tissue volume ratio of 10 to 1 or greater is recommended. Pituitary Gland The pituitary gland can be removed along with the brain (i.e., still attached to the hypothalamus by the infundibular stalk) to avoid damage to the neurohypophysis; this is easier to accomplish in dogs and cats than in large animals, in which the dura mater extends across the dorsal aspect of the hypophysis as a tough fibrous sheet (sellar diaphragm) separating the hypophysis from the hypothalamus except at the infundibular stalk. See Chapter 14, Nervous System for the technique to remove the brain, in which (once the calvaria has been removed) the brain is freed from the cranial vault by severing cranial nerve roots. The optic chiasm (second cranial nerves) can be removed together with the pituitary gland. Alternatively, if the brain is removed separately, leaving the pituitary gland in the sella turcica, it is then practical to remove the pituitary gland together with the hypophyseal venous plexus and adjacent trigeminal nerve roots and ganglia. This is accomplished by incising the sellar diaphragm at the perimeter of the pituitary fossa, grasping the diaphragm with forceps where it joins the dura mater caudal to the pituitary fossa, and then lifting the pituitary gland from its fossa by sharp dissection. The pituitary gland should be dissected free from the trigeminal nerve roots, optic chiasm, and venous plexus for weighing or gross examination. If the brain is exposed by band-sawing the skull longitudinally, care should be taken to saw in a paramedian plane to avoid damaging the pituitary gland. In small animals, the pituitary gland can be fixed by immersion in formalin without sectioning. In large animals or in any animal with a large pituitary mass, sagittal section in the median longitudinal plane facilitates formalin fixation. Pineal Gland Lesions are seldom encountered in the pineal gland, but it can be quite prominent in the horse and other animals with seasonal 770.e1 reproductive activity and so should be recognized grossly as a normal structure. See the text for a description of its location. For formalin fixation, the pineal gland can be left attached to the brain or, if separated, should be placed in a tissue cassette before immersion in formalin. Thyroid Gland In puppies or kittens, the thyroid gland is small and can be examined grossly and then fixed for histologic processing without removing it from the trachea. In larger animals, the thyroid gland is usually removed from the trachea for gross examination and formalin fixation. The right and left lobes can be removed individually or together with the isthmus in animals with a more prominent thyroid isthmus. Remember that the porcine thyroid gland is closer to the thoracic inlet than to the larynx and is not divided into bilateral lobes. Small or flat thyroid glands can be fixed by immersion without sectioning. If the thyroid lobes are thicker than 4 or 5 mm, however, slicing them with a knife or scalpel blade allows more thorough gross examination and facilitates formalin fixation. Parathyroid Glands Feline and canine parathyroid glands have little variation in anatomic location and are closely associated with the thyroid gland. Thus, they usually are not dissected away from the thyroid gland for gross examination or formalin fixation. Parathyroid glands in ruminants, horses, and pigs vary in location and can be difficult to find, so they may not be evaluated in every case in busy diagnostic laboratories in which respiratory and enteric diseases are more often the focus of postmortem examination. If renal or skeletal disease is evident at autopsy, however, an effort should be made to collect parathyroid glands for gross and histologic examination. In most species, the close association of at least one pair of parathyroid glands with the thyroid gland often results in their detection in histologic sections of thyroid gland, either in adjacent adipose or in loose fibrovascular tissue (often just dorsal and medial to each lobe) or within the thyroid gland itself. Porcine parathyroid glands are not closely associated with the thyroid gland and must be sought in loose connective or adipose tissue near the carotid artery bifurcation. If parathyroid glands are collected separately from the thyroid gland, they should be placed in tissue cassettes before immersion in formalin to avoid their loss. Adrenal Glands Abundant adipose tissue can obscure adrenal gland location. The left adrenal gland is usually the easier one to find beside the vena cava and near the cranial pole of the left kidney. The right adrenal gland is typically more cranially located (nearer the liver, just as is the right kidney) and closely associated with the right dorsal aspect of the vena cava. In fact, it can be obscured by the vena cava in the typical ventral abdominal approach to autopsy, so it may be easier to palpate than to see. The adrenal gland should be cut in longitudinal or cross section to evaluate the corticomedullary ratio, look for nodules or other lesions, and facilitate formalin fixation. Pancreatic Islets Because pancreatic islets are typically not visible grossly, they are evaluated as part of the histologic examination of the exocrine pancreas. When pancreatic islets are of interest, at least one complete cross section of the pancreas (fixed by immersion in formalin) should be evaluated histologically. CHAPTER 12 Endocrine System 771 Follicular epithelium C-cells Colloid in follicle Vascular endothelium Nerve fiber A B Capillary Figure 12.5 Thyroid Follicular and Parafollicular (Medullary or C) Cells. A, Colloid-filled follicles are lined by a single layer of follicular epithelium. Individual or clustered C cells are beside the follicular cells or within the thyroid medulla. Note the close proximity of both follicular and C cells to capillaries. B, The cytoplasm of medullary C cells, but not that of follicular cells, in a canine thyroid gland is labeled brown (diaminobenzidine chromogen) with immunohistochemistry for calcitonin. (A courtesy Dr. M.A. Miller, College of Veterinary Medicine, Purdue University; and Dr. J.F. Zachary, College of Veterinary Medicine, University of Illinois. B courtesy Dr. M.A. Miller, College of Veterinary Medicine, Purdue University.) Thyroid Follicular Cells Cuboidal to columnar thyroid follicular cells are arranged in a single layer around follicles filled with colloid.b The follicular cells are equipped for protein (thyroglobulin) production and packaging with their abundant rough ER and well-developed Golgi apparatus. Apical microvilli increase the surface area for interaction with the colloid in the follicular lumen (E-Fig. 12.3). Thyroid hormone production is regulated through the hypothalamic-pituitary-thyroid axis (Figs. 12.6 and 12.7). Low plasma concentrations of thyroxine (tetraiodothyronine or T4) and triiodothyronine (T3) stimulate secretion of thyrotropin-releasing hormone (TRH) by the hypothalamus and thyroid-stimulating hormone (TSH) by pituitary thyrotrophs. When TSH binds with its receptor on follicular cells, the transmembrane receptor associates with G proteins, activating a cAMP-mediated protein kinase signaling cascade that causes hypertrophy and hyperplasia of follicular epithelium (Fig. 12.8, A), and upregulates thyroid hormone production by increasing intracellular calcium concentration and activating phosphokinase C (PKC). Calcium and PKC synergistically activate the dual oxidase (DUOX) complex in the apical plasma membrane to generate the H2O2 needed by thyroid peroxidase as the ultimate electron acceptor. Thyroxine and triiodothyronine are peptide hormones derived from iodinated thyroglobulin, a glycoprotein with numerous tyrosyl residues (see Fig. 12.7). Thyroglobulin is synthesized in the rough ER of the follicular cell, with glycosylation and packaging in the Golgi apparatus for secretion into the follicular lumen. Iodide from the blood is concentrated in follicular cells by the Na+/I- symporter (NIS) in the basolateral plasma membrane and secreted through the apical plasma membrane into the follicular colloid by the Na+independent chloride/iodide transporter pendrin. Human placental trophoblasts have both NIS and pendrin, so they can concentrate iodide from maternal blood, but such transfer would be inefficient bA proteinaceous fluid containing thyroglobulin in the lumen of the thyroid follicle. with the anatomic separation of trophoblasts from maternal blood in the placenta of ruminants, horses, and pigs. The mammary gland also concentrates iodide, making milk another source of iodine for the neonate. In the follicular lumen, iodide is oxidized by thyroid peroxidase to iodine, which then attaches to tyrosyl residues of thyroglobulin. Two iodinated tyrosyl residues, either monoiodotyrosine (MIT) or diiodotyrosine (DIT), are conjugated to form either T4 (two DITs) or T3 (one DIT and one MIT). The concentration of T3 formed is less than that of T4. Thyroid peroxidase catalyzes both the iodination of thyroglobulin and the subsequent linking of the iodinated tyrosyl residues to iodothyronines. Under the influence of TSH, the microvilli of follicular cells elongate and form pseudopodia that resorb colloid by endocytosis. The resorbed colloid droplets fuse with lysosomes, where T4 and T3 are cleaved from the thyroglobulin molecule by proteases. The availability of iodine contributes to the regulation of thyroid function. Excess iodide tends to decrease the responsiveness of follicular cells to TSH, reduce iodide trapping from the blood by downregulating the NIS, inhibit oxidation by thyroid peroxidase, and (at high concentration) inhibit thyroid hormone secretion. Depending on the individual animal, however, excess iodine can result in hyperthyroidism or hypothyroidism. Thyroid hormones act on nearly every cell or tissue in the body with the general effect of increasing the metabolic rate of the targeted cell. Almost all the circulating T4 and T3 is bound to thyroxine-binding globulin, transthyretin, or other carrier proteins. At the target cells, most of the free T4 is deiodinated to T3, which has much higher affinity for nuclear thyroid hormone receptors. The position of the three iodine molecules in T3 (3,5,3′-triiodothyronine) is critical because reverse T3 (3,3′,5′-triiodothyronine), formed in certain disease states such as neonatal protein deficiency, hepatic disease, or renal disease, lacks biologic activity. Elevated circulating T4 and T3 concentration suppresses TSH release through negative feedback on the hypothalamus and adenohypophysis, resulting in atrophy of the follicular cells (see Fig. 12.8, B). CHAPTER 12 Endocrine System L L C V CD E-Figure 12.3 Thyroid Follicular Cells, Thyroid Gland, Normal Dog. Thyroid follicular cells with long microvilli (V) that extend into the colloid (C) within the follicular lumen. Numerous lysosomes (L) and colloid droplets (CD) are present in the apical portion of the follicular cells. An interfollicular capillary (arrow) is present at the base of the follicle. Transmission electron microscopy (TEM). Uranyl acetate and lead citrate stain. (Courtesy Dr. C. Capen, College of Veterinary Medicine, The Ohio State University.) 771.e1 772 SECTION II Pathology of Organ Systems See Fig. 12.2, C for greater detail Capillary lumen Endothelium Hypothalamus Hypothalamic neuronal transport route of thyrotrophin releasing hormone (TRH) Optic chiasm Vesicle with TG Colloid in a follicular lumen Nucleus rER 3 Superior hypophyseal artery Anterior pituitary gland (adenohypophysis) Circulatory system 2 1 Posterior pituitary gland Adenohypophyseal thyrotrophs Capillaries of the anterior pituitary (portal vascular system) Thyroid stimulating hormone (TSH) in circulatory system TSH T3 10 (see microscopic image in Fig. 12.6) 4 Thyroid follicular cell 8 5 Iodination of TG 6 T3 T4 9 7 Conjugation of TG T4 Trachea Thyroglobulin (TG) Thyroglobulin with tyrosyl moieties - Iodide (I ) Oxidized iodine Thyroid gland Thyroid hormones (T3 and T4) Circulatory system Colloid in follicular lumina Thyroid hormones (T3 and T4) Systemic organs and tissues Figure 12.6 Hypothalamic-Pituitary-Thyroid Axis. Thyrotropin-releasing hormone (TRH), synthesized and released from hypothalamic neurons, stimulates adenohypophyseal thyrotrophs to release thyroid-stimulating hormone (TSH), which acts on thyroid follicular cells to promote synthesis and secretion of triiodothyronine (T3) and thyroxine (T4) into the circulation. These thyroid hormones have a positive effect (green arrows) on development, growth, and metabolism in organs and tissues throughout the body. Thyroid hormones exert negative feedback (red arrows) on the adenohypophysis and hypothalamus to regulate their own production. (Courtesy Dr. J.F. Zachary, College of Veterinary Medicine, University of Illinois and Dr. M.A. Miller, College of Veterinary Medicine, Purdue University.) Thyroid C Cells Thyroid C cells (also known as parafollicular or medullary cells) are the second type of thyroid endocrine cell. The C cells are situated beside follicular cells or within the thyroid medulla (between follicles) and in close proximity to blood vessels (see Fig. 12.5; E-Fig. 12.4). C Iodinated TG Iodinated and Triiodothyronine (T3) Thyroxine (T4) conjugated TG Iodine transporters (Na+/I- symporter, pendrin) Figure 12.7 Hormone Synthesis in the Thyroid Follicle. Blood iodide enters the follicular cell (1) through the Na+/I− symporter (basolateral plasma membrane) and is transported into the luminal colloid (right side of drawing) by pendrin (apical plasma membrane). Thyroglobulin (TG) is synthesized from tyrosine and other amino acids in the rough endoplasmic reticulum (rER), glycosylated and packaged into vesicles in the Golgi (2), and secreted into the colloid (3). In the colloid, iodide (4) is oxidized to iodine (5), which then attaches to tyrosyl residues of TG (6). Iodinated tyrosyl residues are conjugated (7) to form T4 and T3 side chains. Colloid that contains conjugated iodinated TG is resorbed into the follicular cell by endocytosis (8). The intracellular colloid droplets fuse with lysosomes where T4 and T3 are enzymatically cleaved from thyroglobulin (9) and then released into the circulation (10). (Courtesy Dr. M.A. Miller, College of Veterinary Medicine, Purdue University; and Dr. J.F. Zachary, College of Veterinary Medicine, University of Illinois.) cells produce calcitonin, a polypeptide hormone that is stored in secretory granules. Calcitonin acts to reduce the blood calcium (Ca2+) concentration, and it generally opposes the effects of parathyroid hormone (see section on Structure and Function, Parathyroid Glands). C cells can be distinguished from follicular cells with immunohistochemistry, using antibody to calcitonin (see Fig. 12.5, B), or with less specific neuroendocrine markers, such as chromogranin or PGP 9.5. Unlike thyroid follicular cells, C cells are not under the control of TSH. Instead, they respond to plasma concentration of Ca2+. When blood calcium concentration is low, numerous secretory granules accumulate in quiescent C cells (Fig. 12.9). Nevertheless, C cells rapidly secrete calcitonin in response to hypercalcemia. Thus, the secretory granules are depleted, and C cells undergo hypertrophy with amplification of rough ER and Golgi apparatus. Long-term hypercalcemia causes hyperplasia of C cells. Calcitonin acts mainly in bone and kidney to downregulate blood calcium concentration. Calcitonin inhibits resorption of mineral from bone by binding and inhibiting osteoclasts, thereby having the opposite effect of parathyroid hormone (see Structure and Function, CHAPTER 12 Endocrine System S C E-Figure 12.4 Thyroid C Cell, Thyroid Gland, Normal Dog. Thyroid C (parafollicular) cell with numerous secretory granules (S) and moderate development of Golgi apparatus and rough endoplasmic reticulum. Microvilli from follicular cells (arrow) extend into the colloid of the follicular lumen (C). The secretory polarity of the C cell is directed toward an interfollicular capillary (arrowhead) with fenestrae. Transmission electron microscopy (TEM). Uranyl acetate and lead citrate stain. (Courtesy Dr. C. Capen, College of Veterinary Medicine, The Ohio State University.) 772.e1 CHAPTER 12 Endocrine System A 773 B Figure 12.8 Regulation of Thyroid Follicular Epithelium. A, Follicular hyperplasia, thyroid gland, horse. Follicular cells under the influence of thyroid-stimulating hormone (TSH) are hypertrophied (tall columnar) and crowded, impinging on the follicular lumen. In follicles with an open lumen, the colloid is pale eosinophilic with resorption vacuoles at the apical surface of follicular cells. Hematoxylin and eosin (H&E) stain. B, Follicular atrophy, thyroid gland, dog. Thyroid follicular cells (arrow) after long-term administration of exogenous thyroxine (T4)—and therefore minimal secretion of TSH by adenohypophyseal thyrotrophs— are low cuboidal. The follicles are distended with densely stained colloid. Note the lack of apparent resorption vacuoles. Periodic acid-Schiff reaction. (A courtesy Dr. B. Harmon, College of Veterinary Medicine, The University of Georgia; and Noah’s Arkive, College of Veterinary Medicine, The University of Georgia. B courtesy Dr. C. Capen, College of Veterinary Medicine, The Ohio State University.) Hypercalcemia Thyroid C cell Serum calcium (10 mg/100 mL) Parathyroid chief cell Hypocalcemia Figure 12.9 Response of Thyroid C Cells and Parathyroid Chief Cells to Hypercalcemia and Hypocalcemia. In response to hypocalcemia, C cells become quiescent and accumulate secretory granules, whereas chief cells are nearly degranulated but have hypertrophied rough endoplasmic reticulum and Golgi apparatus for synthesis and packaging of parathyroid hormone. The opposite occurs in response to hypercalcemia—that is, C cells degranulate and undergo hypertrophy, and chief cells return to a quiescent stage. (Redrawn with permission from Dr. C. Capen, College of Veterinary Medicine, The Ohio State University.) Parathyroid Glands and Fig. 12.9). In the kidney, however, calcitonin and parathyroid hormone act synergistically to decrease renal tubular reabsorption of phosphorus. Parathyroid Glands Most domestic mammals have two pairs of parathyroid glands, so named because of their location beside the thyroid gland. The dog and cat have bilateral external and internal parathyroid glands that truly are beside or within the thyroid gland. The pig has only one pair of parathyroid glands that are located cranial to the thyroid gland, embedded either in the thymus in young pigs or in adipose tissue in adults. In cattle and sheep, the larger external parathyroid gland is cranial to the thyroid gland in loose connective tissue along Figure 12.10 Parathyroid Gland, Normal Dog. Numerous chief cells are separated and supported by a fine fibrovascular stroma. Hematoxylin and eosin (H&E) stain. (Courtesy Dr. J.F. Zachary, College of Veterinary Medicine, University of Illinois.) the common carotid artery. The smaller internal parathyroid glands are situated on the dorsal and medial surface of the thyroid lobes. In horses, the larger (“lower”) parathyroid gland is at a considerable distance from the thyroid gland in the caudal cervical region, whereas the smaller (“upper”) parathyroid gland is near the thyroid gland. The parathyroid glands are composed mainly of cords of chief cells in a fine fibrovascular stroma (Fig. 12.10). Chief cells produce and release parathyroid hormone (PTH) in response to decreased ionized calcium in peripheral blood. They can respond rapidly to changes in blood Ca2+ concentration with “bypass secretion”c (E-Fig. 12.5) of newly synthesized PTH. Unneeded PTH is stored in the cytosol as secretory granules. Oxyphil cells, often in clusters, are a second type of parathyroid cell that may be conspicuous by virtue cNewly synthesized PTH is rapidly released at the plasma membrane from small vesicles into extracellular fluid without interacting with (“bypass”) mature secretory granules in the cytosolic storage pool of PTH. CHAPTER 12 Endocrine System Chief cells Time (min) Cytosol RER GA Cytosol 0 1 10 20 Pro-PTH "New" PTH Amino acids Pre-pro PTH 773.e1 "New" t = 3 hr "Old" 1/2 secretory secretory granules granules Degradation (lysosomal "By-pass" enzymes) secretion Ca2+ Ca2+ cAMP, β-agonist Secreted PTH E-Figure 12.5 Chief Cell Bypass Secretion of Parathyroid Hormone (PTH) in Response to Hypocalcemia. Newly synthesized and processed PTH can be released directly into the blood without entering the storage pool of “old” secretory granules. Whereas PTH from the storage pool is mobilized by cyclic adenosine monophosphate (cAMP), β-agonists (e.g., epinephrine, norepinephrine, and isoproterenol), and low blood Ca2+ concentration, newly synthesized PTH is secreted only in response to low Ca2+ concentration. GA, Golgi apparatus; RER, rough endoplasmic reticulum. (Redrawn with permission from Dr. C. Capen, College of Veterinary Medicine, The Ohio State University.) 774 SECTION II Pathology of Organ Systems of their more abundant and more eosinophilic cytoplasm (because of numerous hypertrophied mitochondria) but few, if any, secretory granules; their function is poorly understood. The overall action of PTH is to mobilize calcium into extracellular fluid (Fig. 12.11). In the kidney, PTH acts within minutes to block reabsorption of phosphorus in proximal tubules and to enhance reabsorption of calcium in distal tubules. In bone, PTH activates osteoclasts indirectly by binding to its receptor on osteoblasts (see Chapter 16, Bones, Joints, Tendons, and Ligaments). PTH also promotes absorption of calcium from the intestine. Calcitonin, PTH, and cholecalciferol act in concert to regulate the calcium/phosphorus balance (see Fig. 12.11). From a functional standpoint, vitamin D brings about the retention of sufficient mineral ions for mineralization of bone matrix, whereas PTH maintains the proper calcium to phosphorus ratio in extracellular fluid. Cholecalciferol (vitamin D3) acts in the small intestine to promote the absorption of calcium from the orad and phosphorus from the aborad mucosa. Adrenal Gland The adrenal gland is so-named for its position just craniomedial to the kidney. Although classified anatomically as one gland, the adrenal cortex has mesodermal origin, and adrenocortical cells synthesize steroid hormones (corticosteroids) from cholesterol (E-Fig. 12.6), whereas the adrenal medulla is derived from neural crest ectoderm and its cells produce catecholamines from tyrosine (E-Fig. 12.7). The adrenal medulla is surrounded by the adrenal cortex (Fig. 12.12), so medullary cells are exposed to cortisol-rich blood. This close anatomic association between adrenal cortex and medulla is important because the phenylethanolamine-N-methyl transferase that converts norepinephrine to epinephrine is corticosteroid hormone-dependent. Interestingly, ACTH also contributes Gastrointestinal epithelium Osteoclast Dietary Ca2+ HPO4− Gastrointestinal lumen R 1,25(OH)2 VD3 ( PTH) ECF Ca2+ PTH 1,25(OH)2 VD3 Feces Renal tubular epithelium Bone fluid compartment CT (+) (–) PTH R (+) Ca2+ Bone mineral HPO4– PTH CT Glomerular filtrate Osteocyteosteoblast "pump" Osteoblast Urine Figure 12.11 Interaction of Parathyroid Hormone (PTH), Calcitonin (CT), and 1,25-Dihydroxycholecalciferol (1,25-[OH]2 VD3) in Hormonal Regulation of Calcium and Phosphorus in Extracellular Fluids (ECF). (Redrawn with permission from Dr. C. Capen, College of Veterinary Medicine, The Ohio State University.) zg zf zr m A B Figure 12.12 Adrenal Gland, Normal Dog. A, Cross section of adrenal gland. The cortex surrounds the medulla. Note prominent sinusoidal vasculature at the corticomedullary junction. The rectangle outlines an area of the corticomedullary junction like that depicted at higher magnification in Fig. 12.13. B, Interface between the cortical cells of the zona reticularis (top) and the finely granular chromaffin cells of the adrenal medulla (bottom). Hematoxylin and eosin (H&E) stain. m, Medulla; zf, zona fasciculata; zg, zona glomerulosa; zr, zona reticularis. (A courtesy Dr. M.A. Miller, College of Veterinary Medicine, Purdue University. B courtesy Dr. J.F. Zachary, College of Veterinary Medicine, University of Illinois.) CHAPTER 12 Endocrine System 774.e1 Steroid Hormones Produced by the Adrenal Cortex Cholesterol Zona glomerulosa Zona fasciculata Cortex Medulla Zona reticularis Adrenal gland 1 Pregnenolone 2 3 Pregnenolone Progesterone 17-OH Pregnenolone 11-deoxycorticosterone 17-OH Progesterone Corticosterone 11-deoxycortisol 11-deoxycorticosterone Aldosterone Cortisol Corticosterone Progesterone 4 Pregnenolone 17-OH Pregnenolone Dehydroepiandosterone Androstenedione E-Figure 12.6 Biosynthesis of Adrenocortical Steroid Hormones. Aldosterone (1) is the major mineralocorticoid produced by cortical cells of the zona glomerulosa. Cortisol is the major glucocorticoid (2, 3) produced by cortical cells in the zona fasciculata. Cells of the zona reticularis produce androgens (4) and lesser quantities of glucocorticoids. (Courtesy Dr. M.A. Miller, College of Veterinary Medicine, Purdue University; and Dr. J.F. Zachary, College of Veterinary Medicine, University of Illinois.) 774.e2 SECTION II Pathology of Organ Systems Catecholamines Produced by the Adrenal Medulla Tyrosine Cortex Medulla 3,4-dihydrophenylalanine (DOPA) Adrenal gland Dopamine Norepinephrine Epinephrine E-Figure 12.7 Biosynthesis of Adrenal Medullary Catecholamines from Tyrosine. (Courtesy Dr. M.A. Miller, College of Veterinary Medicine, Purdue University; and Dr. J.F. Zachary, College of Veterinary Medicine, University of Illinois.) 775 CHAPTER 12 Endocrine System produce mineralocorticoids, mainly aldosterone. Aldosterone controls blood pressure and extracellular fluid volume by acting at distal and collecting tubules of the kidney to promote sodium retention and potassium excretion (see Fig. 12.13, A). The zona glomerulosa has minimal response to ACTH (in contrast to the inner layers of the adrenal cortex) and is regulated mainly by the renin-angiotensin-aldosterone system with feedback from K+ concentration in the plasma. Renin, secreted by juxtaglomerular cells of the kidney (see Fig. 12.13, A) in response to lowered blood pressure (and other factors), converts angiotensinogen to angiotensin I, which, in turn, is to catecholamine synthesis by stimulating the activity of two key enzymes: tyrosine hydroxylase and dopamine-β-hydroxylase. Adrenal Cortex Adrenocortical cells, especially those of the inner layers (i.e., zonae), have vacuolated, lipid-laden cytoplasm typical of steroid hormone-producing cells; the cytoplasmic lipid imparts a yellowish cast to the cortex. The adrenal cortex is divided into zonae glomerulosa, fasciculata, and reticularis (Fig. 12.13). The zona glomerulosa is the outermost layer, in which cells arranged in arcuate formations 1 Decrease in renal perfusion Proximal convoluted tubule Juxtaglomerular complex E 2 3 Macula densa Renal glomerulus A Liver Juxtaglomerular cells A = Afferent arteriole E = Efferent arteriole 4 Renin Medulla Angiotensinogen 5 Angiotensin converting enzyme (ACE) 6 Adrenal gland Angiotensin I Angiotensin II Cortex 7 8 Systemic vasculature Renin Systemic vasoconstriction Action of aldosterone on the distal renal tubule H2CO3 ECF Osmotic Aldosterone 9 Glomerular filtrate Na+ Cl− gradient H2O Systemic vasculature H2O Na+ K+ H+ Cl− H+ K+ HCO3− 10 Epithelium of distal tubule Zona glomerulosa ECF K+ Figure 12.13 Regulation of Adrenocortical Function. A, Mineralocorticoid synthesis in the zona glomerulosa (hematoxylin and eosin [H&E] stain) is regulated through the renin-angiotensin-aldosterone system. A decrease in renal perfusion (1) activates the juxtaglomerular complex (2). Macula densa cells signal juxtaglomerular cells (3) to release renin (4) into the systemic vasculature. Renin converts angiotensinogen to angiotensin I (5), which is converted to angiotensin II by angiotensin-converting enzyme (6). Angiotensin II causes vasoconstriction (7) and stimulates aldosterone synthesis by adrenocortical cells of the zona glomerulosa (8). Aldosterone acts on renal distal and collecting tubules (9) to maintain extracellular fluid volume (ECF) by promoting excretion of K+ and resorption of Na+. Continued 776 SECTION II Pathology of Organ Systems See Fig. 12.2, C for greater detail Hypothalamus Corticotrophin releasing hormone (CRH) Optic chiasm Anterior pituitary gland C ZG Adenohypophyseal corticotrophs ZF Adrenocorticotrophic hormone (ACTH) Circulatory system Cortisol Systemic organs and tissues ZR Medulla M Adrenal gland B Cortex Circulatory system Cortisol Figure 12.13 cont’d B, The three layers of the adrenal cortex, from outer to inner, are the zona glomerulosa (ZG), zona fasciculata (ZF), and zona reticularis (ZR). Glucocorticoid synthesis in the ZF and ZR is regulated through the hypothalamic-pituitary-adrenocortical axis. Corticotrophin-releasing hormone (CRH) from the hypothalamus promotes synthesis and release of adrenocorticotrophic hormone (ACTH) from the adenohypophysis. ACTH acts on the ZF and ZR to increase production and secretion of cortisol and other glucocorticoids. Increased plasma concentration of cortisol provides negative feedback to the adenohypophysis and hypothalamus. Adrenal capsule (C) is at top; adrenal medulla (M) is at bottom. H&E stain. (A and B courtesy Dr. M.A. Miller, College of Veterinary Medicine, Purdue University; and Dr. J.F. Zachary, College of Veterinary Medicine, University of Illinois. Effect of aldosterone on distal nephron redrawn with permission from Dr. C. Capen, College of Veterinary Medicine, The Ohio State University.) converted in the lung to angiotensin II by an angiotensin-converting enzyme (ACE). Angiotensin II raises blood pressure by contracting vascular smooth muscle; it also acts on the zona glomerulosa to stimulate synthesis and release of aldosterone. The zona fasciculata is the middle and largest layer of the cortex. Its cells produce cortisol and other glucocorticoids; thus it responds to stimulation by ACTH (see Fig. 12.13, B) released into the systemic circulation by the adenohypophysis. Glucocorticoids have diverse actions on many organs and tissues throughout the body, but in general they tend to increase glucose production, decrease lipogenesis, suppress the immune response, and inhibit inflammation and its repair by fibroplasia (E-Fig. 12.8). Cells of the inner adrenocortical layer, the zona reticularis, produce sex hormones (androgens and estrogens), especially in castrated animals, and lesser concentrations of glucocorticoid hormones. Adrenal Medulla In contrast to the lipid-vacuolated cytoplasm of steroid-producing adrenocortical cells, the chromaffin cells of the adrenal medulla have finely granular basophilic to amphophilicd cytoplasm (see Fig. 12.12, B). Adrenal medullary cells produce the catecholamine dStains purple in histologic sections because of affinity for acidic (red) and basic (blue) dyes. hormones norepinephrine and epinephrine from tyrosine (see E-Fig. 12.7). Most of the epinephrine in circulation, but only a minor portion of circulating norepinephrine, is produced by the adrenal medulla. Epinephrine acts by nonselectively binding adrenergic receptors to exert a variety of effects in most tissues throughout the body. Stress, especially frightening stress that induces the “fight or flight” response, is the major trigger of epinephrine (also known as adrenaline) release. Norepinephrine is a major sympathetic neurotransmitter, with less importance as a hormone. Pancreatic Islets The bulk of the pancreas is an exocrine gland (see Chapter 8, Hepatobiliary System and Exocrine Pancreas). The endocrine component is contained in its islets of Langerhans (Fig. 12.14). Pancreatic islet cells have abundant rough ER and well-developed Golgi apparatus to produce and package their polypeptide hormones (E-Fig. 12.9). The islets contain a variety of hormone-producing cells, but this chapter is focused on the insulin-secreting β cells, which are confined to pancreatic islets and are the most common islet cell, and the glucagon-secreting α cells. Insulin and glucagon act in concert to control the glucose concentration in extracellular fluid. Insulin is secreted in response to elevated blood glucose and functions to transfer glucose from the blood into cells (especially hepatocytes, CHAPTER 12 Endocrine System 776.e1 A E-Figure 12.8 Dehiscence of Surgical Wound, Skin, Ventral Abdomen, Dog. Wounds heal slowly in dogs with cortisol excess because of an inhibition of fibroblastic proliferation. (Courtesy Dr. C. Capen, College of Veterinary Medicine, The Ohio State University.) B E-Figure 12.9 Pancreatic Islet, Normal Dog. Differences in secretion granules between β cells (B) and α cells (A); the internal cores of secretion granules in insulin-secreting β cells (arrowheads) are bar- or Y-shaped, with a prominent space between the limiting membrane and the internal core. Secretion granules of the glucagon-secreting α cells have an electron-dense, circular, internal core with a narrow submembranous space (arrow). Transmission electron microscopy (TEM). Uranyl acetate and lead citrate stain. (Courtesy Dr. C. Capen, College of Veterinary Medicine, The Ohio State University.) CHAPTER 12 Endocrine System 777 Endocrine Activity in Adipose Tissue In addition to its metabolic functions of synthesizing fatty acids and storing triglycerides, adipose tissue is a source of adipokines (i.e., chemical compounds produced by adipocytes that are secreted into the blood and act on distant target cells, such as in the hypothalamus, liver, and skeletal muscle). Thus, adipocytes are cells capable of endocrine signaling. Two adipokines have particular metabolic importance: leptin and adiponectin. Leptin is involved in appetite suppression and heat generation, and it is proinflammatory. In contrast, adiponectin enhances glucose uptake and metabolism, and it is antiinflammatory. See subsequent sections on Diseases Affecting Multiple Species of Domestic Animals, Obesity, and Diseases of Horses and Diseases of Pigs, Metabolic Syndrome. Dysfunction/Responses to Injury Figure 12.14 Pancreatic Islet, Normal Dog. The islet is surrounded by the exocrine pancreas. Hematoxylin and eosin (H&E) stain. (Courtesy Dr. J.F. Zachary, College of Veterinary Medicine, University of Illinois.) adipocytes, and skeletal myocytes) and to enhance glucose oxidation, glycogenesis, lipogenesis, and formation of ATP and nucleic acids. Glucagon, secreted in response to decreased blood glucose concentration, works in opposition to insulin and promotes glycogenolysis, gluconeogenesis, and lipolysis. Islet α cells, like the other non-β cell types (e.g., somatostatin-secreting δ cells and pancreatic polypeptide-secreting PP cells), are not restricted to pancreatic islets and can be found in other, mainly gastrointestinal, sites. Pineal Gland The pineal gland, situated between the cerebral hemispheres just dorsal and caudal to the thalamus, is seldom associated with disease in domestic mammals. The pineal gland is derived from the ependymal lining of the third ventricle in the developing diencephalon and is first observed at 30 to 40 days of gestation in the bovine fetus. Its importance in adult animals lies in its ability to relay information about photoperiod length to the hypothalamic-pituitary axis and thereby regulate circadian rhythm and seasonal reproduction. Pinealocytes secrete several neurotransmitters in addition to the hormone melatonin, a polypeptide derivative of tryptophan, in response to decreasing daylight hours. Melatonin binds receptors in the pituitary pars tuberalis and blocks the action of gonadotrophin-releasing hormone on the adenohypophysis. Lengthening of the photoperiod suppresses melatonin secretion, which explains increased reproductive activity in the spring, especially in seasonal breeders. Consequently, horses and small ruminants tend to have particularly prominent pineal glands compared with the other domestic species. Chemoreceptor Organs Chemoreceptor organs, such as the carotid bodies (near the bifurcation of the carotid arteries) and the aortic body (adjacent to the ascending aorta at the base of the heart), are clusters of glomus cells supported by glia-like cells. The glomus cells have numerous vesicles that contain various neurotransmitters (e.g., dopamine, catecholamines) used to relay their response to hypoxia to the nervous system. Lesions are not commonly encountered in the carotid or aortic bodies of domestic mammals, but the aortic body in particular is an occasional source of neuroendocrine neoplasia, known as chemodectoma (see Diseases Affecting Multiple Species of Domestic Animals, Disorders of the Chemoreceptor Organs), particularly in brachycephalic dogs. Mechanisms of Endocrine Diseases Endocrine organs are subject to all categories of injury, including degeneration and necrosis, vascular disturbances, inflammation from immune-mediated or infectious causes, and disturbances of growth (e.g., atrophy, hyperplasia, or neoplasia). Nevertheless, growth disturbances account for a disproportionate number of diagnoses. Disturbances of growth (whether atrophic or proliferative) in an endocrine organ can alter its function and have striking effects on distant and diverse target organs. These target organ injuries often account for the clinical presentation and major lesions. For example, cutaneous lesions can reflect hypothyroidism or hyperadrenocorticism; hyperinsulinemia can manifest as seizures. The clinical signs of endocrine disease reflect hypofunction or hyperfunction. Hypofunction of endocrine tissue usually indicates insufficient production or release of hormone(s); hyperfunction is usually the result of excessive hormone production. Endocrine dysfunction can also result from (1) inability of target cells to respond to hormones, (2) systemic disease or metabolic disturbances, or (3) administration of exogenous hormones. Hypofunction of an Endocrine Gland Primary Hypofunction. Hypofunction is considered primary if the hormonal deficiency is the result of a biochemical defect in synthesis (e.g., dyshormonogenetic goiter; see Diseases Affecting Multiple Species of Domestic Animals, Disorders of the Thyroid Gland, Follicular Hyperplasia and Goiter, Congenital Dyshormonogenetic Goiter) or the result of either failure of glandular development (e.g., from aplasia or hypoplasia) or destruction of the secretory cells of the gland. An example of primary hypofunction caused by anomalous development is canine panhypopituitarism that results from failure of oropharyngeal ectoderm to differentiate into the adenohypophysis (see Diseases of Dogs). Most endocrine organs are susceptible to immune-mediated injury in which autoreactive T lymphocytes and autoantibodies selectively destroy endocrine cells. Unlike immune-mediated diseases, infectious diseases seldom selectively attack a particular endocrine organ; however, endocrine glands, especially the adrenal glands, are vulnerable to inflammation and necrosis in systemic infections and to metastatic neoplasia. Secondary Hypofunction. Hypofunction is considered secondary if the cause arises outside the hypofunctioning gland. Often, this outcome involves injury of the pituitary gland with resultant trophic hormone deficiency. In other words, primary hypofunction of the pituitary gland causes secondary hypofunction of those endocrine glands that depend on its trophic hormones. In addition to the 778 SECTION II Pathology of Organ Systems Table 12.1 n Primary Hyperfunction of Endocrine Glands Neoplasia Hormone Lesion/Sign Somatotroph adenoma (pituitary gland) Thyroid follicular cell adenoma C-cell adenoma/ carcinoma (thyroid gland) Adrenocortical adenoma/carcinoma Pheochromocytoma (adrenal medulla) Parathyroid chief cell adenoma Pancreatic β-cell adenoma/carcinoma Growth hormone T4, T3 Acromegaly Calcitonin Cortisol Norepinephrine Parathyroid hormone Insulin ↑Basal metabolic rate Osteosclerosis Alopecia, polyuria/ polydipsia Hypertension Hypercalcemia Hypoglycemia m Figure 12.15 Secondary Adrenocortical Hypofunction; Brain with Neoplasm and Left (Longitudinal Section) and Right (Cross Section) Adrenal Glands, Dog. The neoplasm (n), centered around the third ventricle, has invaded and destroyed the pituitary gland, hypothalamus, and most of the thalamus. Destruction of the adenohypophysis caused a lack of adrenocorticotrophic hormone (ACTH) and other trophic hormones, resulting in bilateral adrenocortical atrophy (arrowheads), especially in the ACTH-dependent zonae fasciculata and reticularis, and (consequently) a relatively more prominent adrenal medulla (m). (Courtesy Dr. C. Capen, College of Veterinary Medicine, The Ohio State University.) previously mentioned causes of (primary) pituitary hypofunction, nonfunctional (hormonally inactive) pituitary adenomas or even nearby neoplasms of nonpituitary origin can become large enough to destroy the adenohypophysis. Because neither the pituitary neoplasm nor the residual adenohypophyseal cells would then produce sufficient trophic hormones, atrophy with secondary hypofunction develops in target tissues, such as the adrenal cortex (Fig. 12.15), thyroid follicles, or gonads. Hyperfunction of an Endocrine Gland Primary Hyperfunction. In primary hyperfunction, the cells of the affected endocrine gland autonomously (i.e., without dependence on trophic hormone stimulation) synthesize and secrete excess hormone. This outcome is usually the result of a functional (i.e., hormone-producing) neoplasm. Examples and consequences of primary endocrine gland hyperfunction caused by neoplasms are listed in Table 12.1. Although primary neoplasia is the major cause of primary hyperfunction of an endocrine gland, hyperfunction is not the inevitable result of endocrine neoplasia. Nonfunctional endocrine neoplasms (and even once-functional neoplasms that lose their capacity to produce or secrete bioactive hormone) can, as they increase in size, destroy surrounding glandular tissue and result in hypofunction. Secondary Hyperfunction. In secondary hyperfunction, excessive hormone production is a response to a signal (e.g., a trophic hormone) from outside the hyperfunctioning gland. For example, a functional neoplasm of adenohypophyseal (anterior pituitary gland) corticotrophs, with prolonged and excessive secretion of ACTH, would cause diffuse adrenocortical hyperplasia of the zonae fasciculata and reticularis (Fig. 12.16; E-Fig. 12.10) with resulting increased synthesis and secretion of cortisol. Theoretically, secondary hyperplasia and hyperfunction of an endocrine gland should subside when the stimulus of excessive trophic hormone is removed; however, chronic and severe hyperplasia is not always reversible. Endocrine cell proliferation can also be nodular or even clonal rather than diffuse. With long-term trophic hormone stimulation, there seems to be a continuum between focal or nodular hyperplasia and neoplasia. Secondary hyperfunction can also develop in endocrine glands or cells that are not under the control of pituitary trophic hormones. In renal secondary hyperparathyroidism and nutritional secondary hyperparathyroidism (see Diseases Affecting Multiple Species of Domestic Animals, Disorders of the Parathyroid Glands, Chief Cell Proliferation and Hyperparathyroidism), the parathyroid glands respond to decreased blood calcium concentrations with hyperplasia and increased production and secretion of PTH. Hypersecretion of Hormones or Hormone-Like Factors by Nonendocrine Neoplasms Some nonendocrine neoplasms secrete biologically active humoral substances. Most of these hormone-like chemicals produced by neoplastic cells are peptides because nonpeptide hormones (steroids, iodothyronines, or catecholamines) have more complex synthetic pathways. Pseudohyperparathyroidism or humoral hypercalcemia of malignancy (HHM) is a paraneoplastic syndrome caused by the autonomous hypersecretion of PTH-related peptide (PTHrP) by cancer cells. A well-characterized example is the canine apocrine carcinoma of the anal sac glands (see Diseases of Dogs). PTHrP acts as an agonist of the PTH receptor in target cells (e.g., in bone and kidney), leading to persistent hypercalcemia. Serum PTH concentration is decreased in response to the hypercalcemia, and PTH is not detectable in the neoplastic tissue. Endocrine Dysfunction Caused by Failure of Target Cell Response Endocrine dysfunction can be the result of failure of the target organ or tissue to respond to a hormone because of defective cell surface receptors or second messenger systems. For example, downregulation of insulin receptors on target cells (especially adipocytes, hepatocytes, or myocytes) can result in insulin resistance in obese animals. Iatrogenic Syndromes of Hormone Excess The administration of exogenous hormones has far-reaching effects on diverse populations of target cells and can result in clinically CHAPTER 12 Endocrine System E-Figure 12.10 Corticotroph Adenoma, Adenohypophysis, Dog. The corticotroph adenoma consists of a sheet of monotonous chromophobic cells with abundant pale amphophilic cytoplasm. Note the lack of acidophils. Hematoxylin and eosin (H&E) stain. (Courtesy Dr. M.A. Miller, College of Veterinary Medicine, Purdue University.) 778.e1 CHAPTER 12 Endocrine System 779 c m a A B zg Figure 12.17 Iatrogenic Hyperadrenocorticism, Left and Right Adrenal Glands, Dog. Hyperadrenocorticism, caused by long-term administration of exogenous glucocorticosteroids, has resulted in trophic atrophy of the adrenocorticotrophic hormone (ACTH)-dependent zonae fasciculata and reticularis of the adrenal cortex (c). Consequentially, the adrenal medulla (m) makes up a relatively greater proportion of the cross-sectional area. (Courtesy Dr. C. Capen, College of Veterinary Medicine, The Ohio State University.) important functional disturbances. The long-term administration of glucocorticoids, used to treat a variety of conditions, can reproduce most of the abnormalities of spontaneous adrenocortical hyperfunction. Nevertheless, although naturally occurring adrenocortical hyperfunction is usually the result of a disrupted hypothalamicpituitary-adrenocortical axis with hyperplasia or neoplasia of endocrine cells, iatrogenic glucocorticoid excess tends to cause profound atrophy of the adrenal cortex (especially in the ACTH-dependent zonae fasciculata and reticularis) via negative feedback through an intact hypothalamic-pituitary-adrenocortical axis (Fig. 12.17). Likewise, long-term administration of exogenous thyroid hormones results in negative feedback on the hypothalamus and on adenohypophyseal thyrotrophs with diminished TSH secretion and thyroid follicular atrophy (see Fig. 12.8, B). Exogenous sex hormones can also result in endocrine imbalances. For example, the administration of synthetic progestins, such as medroxyprogesterone acetate (also known as megestrol acetate), can induce mammary hypertrophy and hyperplasia in both male and female cats and dogs (see Chapter 18, Female Reproductive System and Mammae). Because hyperplastic mammary epithelial cells can be an extrapituitary source of GH, some progestin-treated dogs also develop acromegaly (E-Fig. 12.11). Portals of Entry/Pathways of Spread zf C Figure 12.16 Secondary Adrenocortical Hyperfunction (PituitaryDependent Hyperadrenocorticism); Brain, Pituitary Gland, and Adrenal Glands, Dog. A, A functional corticotroph (adrenocorticotrophic hormone [ACTH]-secreting) adenoma (a) in the pituitary gland caused diffuse and bilateral adrenocortical hyperplasia (arrows) leading to excessive secretion of cortisol (hyperadrenocorticism) by the zonae fasciculata and reticularis. B, Adenohypophysis. The corticotroph adenoma consists of packets of hypertrophied basophils with densely granulated cytoplasm. Note the lack of acidophils. Hematoxylin and eosin (H&E) stain. C, Adrenal cortex. Diffuse adrenocortical hyperplasia. Cells in the zona fasciculata (zf) are enlarged with abundant lipid-vacuolated cytoplasm. Zona glomerulosa (zg, top) is unaffected. H&E stain. (A courtesy Dr. C. Capen, College of Veterinary Medicine, The Ohio State University. B and C courtesy Dr. M.A. Miller, College of Veterinary Medicine, Purdue University.) Endocrine tissue is well vascularized, so the most common route of entry of a potentially injurious agent—be it microorganisms, leukocytes, trophic hormones, or an exogenous endocrine disruptor—is hematogenous. In addition, inflammation or other disease processes in adjacent tissues can extend to endocrine organs. The pituitary gland, with its neurohypophyseal connection to the hypothalamus and third ventricle of the brain and its adenohypophyseal origin from the craniopharyngeal duct, is particularly susceptible to extension of inflammation or neoplasia from the brain or from the pharynx. Defense Mechanisms/Barrier Systems Because of their ample blood supply, endocrine tissues are subjected to the benefits and potential harm of inflammation and innate and/ or adaptive immune responses. The pituitary gland gains some protection from pharyngeal microbiota by its bony encasement in the sella turcica but, at the same time, is more susceptible than the brain to hematogenous insults because of its lack of a blood-brain barrier. In general, because endocrine cells need ready access to the bloodstream to secrete their hormones, they are vulnerable to injury CHAPTER 12 Endocrine System E-Figure 12.11 Iatrogenic Acromegaly, Beagle (Center) Compared with Unaffected Littermates (Left and Right). Note the coarseness of the facial features and the markedly thick folds of the skin of the face. These characteristic changes are the result of the protein anabolic effects of somatotropin (produced by hyperplastic mammary ductular epithelial cells), which have been stimulated by the administration of exogenous medroxyprogesterone acetate. (Courtesy Dr. P. Concannon, College of Veterinary Medicine, Cornell University.) 779.e1 780 SECTION II Pathology of Organ Systems by chemical or infectious agents that arrive hematogenously. Most endocrine glands are protected from the external environment by their deep-seated location and from neighboring tissues or body cavities by a thin capsule of fibrous tissue. Diseases Affecting Multiple Species of Domestic Animalse Disorders of the Adenohypophysis Adenohypophyseal proliferation (hyperplasia or pituitary adenoma) is the most common postmortem lesion in canine pituitary glands. Secondary neoplasia, inflammation, or degeneration and necrosis unassociated with a pituitary tumor are rare. This predominance of proliferations among adenohypophyseal lesions seems to be true for the other domestic species as well. The increasing use of transsphenoidal hypophysectomy in the diagnosis and treatment of pituitary tumors in dogs and cats has changed adenohypophyseal pathology from a strictly postmortem endeavor to an important surgical biopsy practice. A Proliferative Disorders of the Adenohypophysis Hyperplasia and neoplasia are important lesions of the adenohypophysis. Physiologic hyperplasia is the result of stimulation through the hypothalamic-pituitary-target organ axis and tends to affect the trophic hormone-producing cells of interest throughout the adenohypophysis. In contrast, pathologic proliferation tends to be focal or multifocal. Nodular proliferations of cells in the pars distalis or pars intermedia that are multiple and smaller than 2 mm in diameter are typical of hyperplasia. Hyperplastic nodules do not disrupt the reticulin scaffolding of the adenohypophysis, but they can be recognized histologically because the nodule is composed mainly of one cell type (e.g., corticotrophs) (Fig. 12.18, A and E-Fig. 12.12), instead of the normal mixture of corticotrophs, somatotrophs, and other trophic hormone-producing cells. Immunohistochemistry can be used to identify the trophic hormone produced. Hyperplastic nodules are seldom large enough to be evident on clinical diagnostic imaging or gross examination; nevertheless, they can secrete excessive bioactive trophic hormones and thus be responsible for hyperplasia or even secondary hyperfunction of the targeted endocrine organ, depending on which trophic hormone is produced. Adenohypophyseal neoplasms are usually solitary and almost always classified as adenomas rather than carcinomas. It is plausible that hyperplastic nodules of adenohypophyseal cells could become clonal or that multiple nodules could coalesce to form an adenoma. Importantly, smaller adenohypophyseal lesions (whether hyperplastic or neoplastic [microadenomas]) are likely to be functional—that is, to produce and release trophic hormones into the peripheral blood—whereas large macroadenomas (see Fig. 12.18, B) may exert their effect mainly through destruction of adjacent pituitary parenchyma, often resulting in insufficient (rather than excessive) trophic hormone(s) or through compression of surrounding tissue, such as optic nerves or overlying hypothalamus. In addition to classifying nonphysiologic pituitary proliferations as hyperplastic nodules, microadenomas, or macroadenomas, it is useful to determine the type of trophic hormone produced by the proliferating cells. In veterinary medicine, this goal is generally accomplished by a combination of biochemical analysis of serum, clinical signs, and/ or immunohistochemistry on surgical biopsy or autopsy (syn: necropsy; see E-Appendix 12.1) specimens of affected pituitary gland. ePostmortem examination of the endocrine system is discussed in E-Appendix 12.1. Disorders that are known or thought to have a genetic basis are listed in E-Tables 12.1 and 1.2. a B Figure 12.18 Adenohypophyseal Hyperplasia and Neoplasia, Pituitary Gland. A, Corticotroph hyperplasia, dog. A hyperplastic nodule (lower twothirds), about 1 mm in diameter, consists of corticotrophs with basophilic granular cytoplasm that are hypertrophied, but retain the normal acinar pattern. Adjacent normal adenohypophyseal tissue (upper one-third) is a mixture of acidophils and (smaller) basophils or chromophobes. Note a few acidophils scattered through the hyperplastic nodule (arrow). Hematoxylin and eosin (H&E) stain. B, Pituitary macroadenoma, dog. The large adenoma (a) compresses the brain and optic chiasm (arrow). The adenohypophysis, neurohypophysis, and hypothalamus have been destroyed by the neoplasm. (A courtesy Dr. M.A. Miller, College of Veterinary Medicine, Purdue University. B courtesy Dr. C. Capen, College of Veterinary Medicine, The Ohio State University.) Immunohistochemical expression of trophic hormone in the cytoplasm of adenohypophyseal cells does not necessarily correlate with increased plasma concentrations of bioactive hormone. In poorly differentiated pituitary neoplasms that do not express detectable immunoreactive hormone, polymerase chain reaction (PCR) testing for mRNA of trophic hormones can be used to determine the cell of origin. Pituitary Carcinomas. Pituitary carcinomas are exceedingly rare, but that is at least partly because of stringent classification criteria. By convention, to be classified as malignant, a pituitary neoplasm must metastasize, not merely invade, either within the central nervous system or systemically. Adenomas of the Pars Distalis. Adenomas can arise from any of the trophic hormone-producing cells of the pars distalis. Depending on the cell lineage, the neoplastic cells may produce more than one type of trophic hormone. Of the domestic animal species, pars distalis adenomas are most commonly diagnosed in dogs and cats. CHAPTER 12 Endocrine System E-Table 12.1 780.e1 Inherited Endocrine Diseases of Animals Condition Species/Breed Pattern of Inheritance Adenohypophyseal aplasia Pituitary dwarfism Dyshormonogenetic goiter Jersey and Guernsey cattle German shepherd dogs, Karelian bear dogs, Spitz, toy poodles Abyssinian cats, Afrikaner cattle, rat and toy fox terriers, Saanen dwarf goats, sheep Beagles, Doberman pinschers, golden retrievers, Labrador retrievers Miniature schnauzers Bearded collies, Nova Scotia duck tolling retriever, Portuguese water dogs, standard poodles Chow Chows, Pomeranians, poodles, Samoyeds Keeshond Miniature horses and ponies, miniature swine, Ossabaw pigs Guernsey cattle Unknown Autosomal recessive Autosomal recessive Hypothyroidism Hypoparathyroidism Hypoadrenocorticism Adrenal hyperplasia-like syndrome Diabetes mellitus Metabolic syndrome Concurrent pheochromocytomas and thyroid medullary neoplasms E-Figure 12.12 Corticotroph Hyperplasia, Cat. A hyperplastic nodule (lower right two-thirds), less than 1 mm in diameter, is composed of packets of monomorphic chromophobes with abundant amphophilic cytoplasm. Adjacent normal adenohypophyseal tissue (upper left one-third) is a mixture of acidophils and (smaller) chromophobes. Hematoxylin and eosin (H&E) stain. (Courtesy Dr. M.A. Miller, College of Veterinary Medicine, Purdue University.) Unknown Unknown Unknown or autosomal recessive Unknown Unknown Unknown Unknown CHAPTER 12 Endocrine System Most canine pars distalis adenomas are derived from corticotrophs. Feline pars distalis adenomas in one case series were derived mainly from somatotrophs or gonadotrophs. Pars distalis adenomas that are less than 5 mm in diameter are classified as microadenomas; those adenomas that are larger are classified as macroadenomas (see Fig. 12.18, B). Somewhat surprisingly, the smaller microadenomas may be more likely to secrete bioactive trophic hormone, whereas large macroadenomas may have more of a compressive mass effectf rather than a trophic hormone effect. In fact, very large pituitary neoplasms are likely to result in decreased trophic hormone secretion with panhypopituitarism and atrophy (secondary hypofunction) of the targeted endocrine glands. Histologically, pituitary adenomas are composed of polyhedral or piriform cells that tend to be larger, with more abundant cytoplasm, than nonneoplastic pars distalis cells, but they usually have only mild nuclear atypia and few mitotic figures. Because corticotroph adenomas, somatotroph adenomas, and gonadotroph adenomas are the more common pars distalis neoplasms, they are discussed in the following sections. Lactotroph adenomas are reported mainly in sheep but are not common. Neoplasms of thyrotrophs have been reported in cats, but seem to be rare in all species. Although melanotrophs are scattered lightly through the pars distalis, most neoplasms of melanotrophs arise in the pars intermedia, where they are the predominant cell type (see next section). Corticotroph Adenomas. Functional (ACTH-secreting) corticotroph adenomas are an important cause of canine hypercortisolism (secondary adrenocortical hyperfunction or pituitary-dependent hyperadrenocorticism). Corticotroph adenomas are rare in the other domestic species. The larger macroadenomas can obliterate most of the pituitary gland, resulting in panhypopituitarism with secondary hypofunction of target endocrine organs, and compress the neurohypophysis, optic chiasm, hypothalamus, and thalamus. Histologically, corticotroph adenomas are composed of basophilic (densely granulated) to chromophobic (sparsely granulated) cells arranged in sinusoidal or diffuse patterns (see Fig. 12.16, B and E-Fig. 12.10); the granules are periodic acid-Schiff (PAS)-positive. The sinusoidal pattern consists of packets of polyhedral neoplastic cells surrounded by thin fibrovascular septa and more elongated neoplastic cells that palisade around sinusoids. In the diffuse pattern, neoplastic cells are arranged in sheets. Neoplastic cells in both patterns are often larger than nonneoplastic corticotrophs, with a large hypochromatic nucleus, one or two distinct nucleoli, few mitotic figures, and ample pale eosinophilic to amphophilic cytoplasm with distinct cell boundaries. Secretory granules may be dense and basophilic or sparse and inconspicuous in more chromophobic cells. The neoplastic cells are immunohistochemically positive for ACTH. Ultrastructurally, the neoplastic cells have well-developed rough ER and Golgi apparatus and numerous small (∼170 nm in diameter) dense-core secretory granules. Somatotroph Adenomas. Somatotroph adenoma is the most common pituitary adenoma in cats (also see Diseases of Cats, Hypersomatotropism). It is rare in the other domestic species. Neoplastic somatotrophs are usually larger than their nonneoplastic counterparts and have numerous eosinophilic secretory granules; however, large neoplastic cells with few cytoplasmic granules or only faintly eosinophilic granules are the predominant cell type in some somatotroph adenomas. Hypersecretion of GH, also known as STH, can lead to acromegaly (see Diseases of Cats, Hypersomatotropism); it also promotes hepatocellular synthesis and secretion of insulin-like growth factor-1 (IGF-1), so serum assay for IGF-1 concentration can be used diagnostically. fCompression of normal tissues adjacent to the mass resulting in atrophy and/ or necrosis of affected cells and reduced blood supply to the tissue. 781 Gonadotroph Adenomas. Immunohistochemistry to detect follicle stimulating hormone (FSH) or luteinizing hormone (LH) is not routine in most diagnostic laboratories, so gonadotroph adenomas may be underdiagnosed. Nevertheless, gonadotroph adenoma was the second most common tumor type (after somatotroph adenoma) in a series of feline pituitary adenomas. Histologically, the neoplastic cells were chromophobic and PAS-negative. No clinical evidence of hypergonadotropism or other endocrinopathy was observed in any of the cats; however, all affected cats had been spayed or castrated before diagnosis of the pituitary adenoma. Adenomas of the Pars Intermedia. Adenomas in the pars intermedia are usually derived from melanotrophs. Equine pituitary adenomas (Fig. 12.19) almost always develop in the pars intermedia. In addition, the pars intermedia is a major site (along with the pars distalis) for canine pituitary adenomas. The pars intermedia is also an important site of feline pituitary adenomas. Interestingly, pars intermedia adenomas are practically nonexistent in human beings because the pars intermedia shrinks after fetal life and is only vestigial in adults. Melanotroph Adenomas. Melanotroph adenomas, derived from cells that, like corticotrophs, produce POMC-derived peptides, are the major pituitary neoplasm in horses (see Diseases of Horses) and account for about half of canine pituitary adenomas and a smaller proportion of feline pituitary adenomas. Like corticotroph adenomas, they typically are composed of hypertrophied cells with basophilic to chromophobic, densely to sparsely granulated cytoplasm. The granules are PAS-positive. The presence of colloid-filled follicles in the neoplastic tissue can help distinguish the pars intermedia adenoma from one that originated in the pars distalis, but in large adenomas or in fragmented surgical biopsy specimens, the precise location of the tumor is not always apparent. Immunohistochemically, the neoplastic cells express both α-MSH and ACTH. Functional adenomas that produce and secrete bioactive ACTH can result in hypercortisolism in 50% or more of the canine and feline cases. Nonfunctional adenomas can cause hypopituitarism secondary to destruction of the adenohypophysis (anterior pituitary a Figure 12.19 Adenoma, Brain, Pituitary Gland, Horse. The pituitary gland is enlarged by an adenoma (a) in the pars intermedia. (Courtesy College of Veterinary Medicine, University of Illinois.) 782 SECTION II Pathology of Organ Systems (hypophysitis). The term pituitary apoplexy is used for acute hemorrhagic infarction of the hypophysis, usually in association with a pituitary neoplasm. This condition is diagnosed mainly in human beings but is also observed in dogs and horses. o Inflammation. The pituitary gland can become inflamed as part of a systemic infection, but there are few, if any, infectious agents that target the hypophysis. In a systemic infection, the microbial agent reaches the hypophysis hematogenously. (The blood-brain barrier does not protect the hypophysis.) Pituitary inflammation can also develop as an extension from adjacent tissue (e.g., meninges, brain, pharynx). Lymphoplasmacytic hypophysitis is thought to be an immune-mediated (probably autoimmune) disease and has been described in the canine pituitary gland, but it is less common than similar inflammatory processes in the thyroid or adrenal glands. Disorders of the Neurohypophysis Diabetes Insipidus Figure 12.20 Iatrogenic Adenohypophyseal Atrophy, Dog. The adenohypophysis in this dog, treated with the somatostatin analog pasireotide for a corticotroph macroadenoma, was so small that it was detectable only histologically. o, Optic chiasm. (Courtesy Dr. D. Bruyette, VCA West Los Angeles Animal Hospital and Dr. M.A. Miller, College of Veterinary Medicine, Purdue University.) gland) or diabetes insipidus secondary to destruction of the neurohypophysis (posterior pituitary gland) or compression of the hypothalamus (i.e., mass effect). Miscellaneous Disorders of the Adenohypophysis Cellular Atrophy, Degeneration, and Death. Physiologic hypophyseal atrophy is the result of negative feedback from targeted endocrine organs through the hypothalamic-pituitary-target organ axis. This results in selective atrophy of a specific type of trophic hormone-producing cell. For example, increased plasma concentration of thyroxine causes atrophy of thyrotrophs without affecting other adenohypophyseal cells. Therefore, physiologic atrophy seldom causes grossly appreciable shrinkage of the adenohypophysis. In contrast, profound and generalized adenohypophyseal atrophy can be induced by treatment with the somatostatin analogue pasireotide (Fig. 12.20).g Somatostatin is so named because it inhibits the release of GH from somatotrophs. Injectable pasireotide has been used as treatment of canine and feline pituitary adenomas, and it works by binding somatostatin receptors, which are present on somatotrophs, corticotrophs, and perhaps other trophic-hormone producing cells of the adenohypophysis. In addition to the hypothalamus, other organs and tissues, notably the δ cells of the pancreatic islets, produce somatostatin. Somatostatin inhibits not only the release of GH from somatotrophs but also that of insulin and glucagon from pancreatic islet cells and that of ACTH from corticotrophs. Hypophyseal degeneration or necrosis can also result from compression by a mass within the pituitary gland or in adjacent tissue (see Fig. 12.15). Alternatively, degeneration can be secondary to vascular disturbances or to inflammation in the pituitary gland gPasireotide is a somatostatin analogue that has a 40-fold increased affinity for somatostatin receptor type 5 (SSTR5). Its trade name is Signifor (Novartis Pharmaceuticals Corporation). Diabetes insipidus is a form of polyuria caused by an inability to concentrate urine. It is the result of inadequate synthesis and release of ADH in the central or hypophyseal form of the disorder or the result of the failure of renal tubular epithelial cells to respond to ADH in the nephrogenic form. In either form, hypotonic urine (with osmolality equivalent to or less than that of plasma) is produced, even in the face of water deprivation. The hypophyseal form of diabetes insipidus can be caused by any process that compresses or destroys the pars nervosa, infundibular stalk, or the supraoptic nucleus (so named for its hypothalamic location just dorsal to the optic chiasm). The hypophyseal form can be distinguished from the nephrogenic form of diabetes insipidus by the ability of the patient to concentrate urine after administration of ADH. Neoplasms Most neoplasms of the pars nervosa are extensions of adenohypophyseal adenomas. Brain tumors, particularly ependymomas (see Chapter 14, Nervous System) of the third ventricle, also can extend through the infundibular stalk into the pars nervosa. The pituicytoma is a primary neoplasm of the pituicyte, the glial cell of the pars nervosa. The pituicyte is considered a variant of astrocytes and expresses glial fibrillary acidic protein immunohistochemically. Pituicytomas are rare but have been observed in the domestic species, often as incidental findings in animals with an adenohypophyseal adenoma. Other Neoplastic Disorders of the Hypophysis Secondary Neoplasms Unprotected by the blood-brain barrier, the pituitary gland is vulnerable to metastatic neoplasia. The pars nervosa may be particularly susceptible because of its direct blood supply from the carotid artery. The more common secondary neoplasms of the hypophysis are lymphoma in various species, equine and canine melanoma, and canine thyroid and mammary carcinomas. Secondary neoplasms can also be extensions from adjacent tumors, such as osteosarcoma of the sella turcica or ependymoma of the third ventricle. Suprasellar Neoplasms Although most tumors arising in or above the sella turcica (i.e., suprasellar) originate in the pituitary gland, less common suprasellar neoplasms include meningioma, craniopharyngioma, and germ cell tumors. Suprasellar meningiomas resemble those at other locations (see Chapter 14, Nervous System). Whereas pituitary neoplasms are more common in older animals, craniopharyngiomas and germ cell tumors tend to arise in young adult animals. CHAPTER 12 Endocrine System Craniopharyngiomas (Fig. 12.21, A and B) are rare epithelial tumors that are thought to be derived from the craniopharyngeal duct and Rathke’s pouch remnants. Two histologic patterns are recognized in human beings: the adamantinomatous form, which is more common in childhood and resembles odontogenic tumors such as ameloblastoma, and the squamous (pseudo)papillary form, which c m t A 783 occurs mainly in adults and is thought to develop from metaplasia of adenohypophyseal cells in the pars tuberalis. Both histologic patterns are recognized in domestic mammals, in which craniopharyngioma has been reported only in dogs and cats, and usually in young animals. The neoplastic tissue consists of nests of polyhedral epithelial cells in fibrous stroma. The neoplastic cells have eosinophilic cytoplasm, distinct cell borders, and faint intercellular bridges. The cells express cytokeratins immunohistochemically. Tubular formations lined by ciliated cells and goblet cells in some areas of the tumor reflect its association with the craniopharyngeal duct. Reported veterinary cases have been expansile or infiltrative, but the mitotic index is typically low. Although malignant craniopharyngioma was diagnosed in two cats, metastasis was not reported. Suprasellar neoplasms with a population of germ cells, teratomatous features, or immunoreactivity for α-fetoprotein should be classified as suprasellar germ cell tumors (see Fig. 12.21, C). Intracranial germ cell tumors have been described only in the suprasellar location and only in dogs of the domestic animal species. These infiltrative neoplasms obliterate the pituitary gland and compress the hypothalamus. The predominant cell type is a germ cell that resembles the neoplastic cells of testicular seminoma. As in seminoma, aggregates of small lymphocytes are commonly scattered through the neoplastic stroma. Suprasellar germ cell tumors also include nests of lipid-laden hepatoid cells and may have scattered tubular formations lined by endodermal cells or other teratomatous features. Disorders of the Thyroid Gland Disorders of the thyroid gland are clinically important if they result in thyroid hormone deficiency (hypothyroidism) or excess (hyperthyroidism) or if they produce a mass effect. Other disorders of the thyroid gland may be subclinical and of little importance to the animal. Developmental Malformations B Ectopic Thyroid Tissue. Ectopic thyroid tissue is usually encountered from the base of the tongue along the path of descent of the developing gland, but it can migrate as far caudally as the diaphragm. In dogs, functional nodules of thyroid tissue are common near the ascending aorta at the base of the heart. Functional ectopic thyroid tissue is a source of hormone production after thyroidectomy. It also can be a site of thyroid carcinoma, mainly in dogs, and usually in a mediastinal location (see Diseases of Dogs). Accessory Thyroid Tissue and Thyroglossal Duct Cysts. C Figure 12.21 Non-Pituitary Suprasellar Neoplasms, Dogs. A, Craniopharyngioma (c), left and right adrenal glands and thyroid lobes. The neoplasm has extended dorsally through the hypothalamus and compressed the thalamus (black arrows). Destruction of the pituitary gland resulted in trophic atrophy of the adrenal cortices (white arrows), leaving a prominent medulla (m) surrounded by remaining cortical tissue (mainly zona glomerulosa). Thyroid follicles were also atrophied, but colloid involution maintained the overall thyroid (t) size within normal limits. B, The craniopharyngioma is composed of interconnecting nests of keratinizing epithelial cells in fibrous stroma. Hematoxylin and eosin (H&E) stain. C, Suprasellar germ cell tumors consist predominantly of seminoma-like germ cells with fewer lipid-laden hepatoid cells and scattered aggregates of small lymphocytes. H&E stain. (A courtesy Dr. C. Capen, College of Veterinary Medicine, The Ohio State University. B and C courtesy Dr. M.A. Miller, College of Veterinary Medicine, Purdue University.) Accessory thyroid tissue is derived from remnants of the thyroglossal duct. Thyroglossal duct remnants can also form cysts or sinus tracts along the ventral midline of the neck. The cysts that develop near the base of the tongue are typically lined by stratified squamous epithelium, whereas those nearer the thyroid cartilage of the larynx are more likely to be lined by epithelium that resembles that of thyroid follicles. Grossly, the cysts contain watery to mucoid secretions. They seldom exceed 1 cm in diameter, but they can become inflamed, rupture, and form a fistulous tract to the skin. Rarely, thyroglossal duct cysts undergo neoplastic transformation to carcinoma. Follicular Hyperplasia and Goiter The term goiter denotes a nonneoplastic enlargement of the thyroid gland (Fig. 12.22, A) as a result of follicular cell hyperplasia (see Fig. 12.22, B; also see Fig. 12.8, A), although follicular hyperplasia does not always cause grossly appreciable enlargement. The causes for follicular hyperplasia include iodine deficiency, iodine excess, goitrogens, and defects in the synthesis of thyroid hormones. Goiter can be 784 SECTION II Pathology of Organ Systems diffuse (throughout the gland) or multinodular. Diffuse goiter is typically a compensatory, thyroid stimulating hormone (TSH)-induced response to hypothyroidism (decreased plasma concentrations of T4 A B Figure 12.22 Hyperplastic Goiter, Thyroid Gland, Goat. A, Deficiency of maternal dietary iodine during pregnancy resulted in hyperplasia (and hypertrophy) of thyroid follicular cells in this perinatal goat with symmetric enlargement of both lobes (goiter). B, Follicular cells are increased in number and size, impinging on the follicular lumen. Hematoxylin and eosin (H&E) stain. (A courtesy Dr. O. Hedstrom, College of Veterinary Medicine, Oregon State University; and Noah’s Arkive, College of Veterinary Medicine, The University of Georgia. B courtesy Dr. B. Harmon, College of Veterinary Medicine, The University of Georgia; and Noah’s Arkive, College of Veterinary Medicine, The University of Georgia.) and T3). In contrast, multinodular goiter in old cats (Fig. 12.23, A-B and E-Fig. 12.13) consists of hyperplastic follicular cells that function autonomously (independent of TSH) with resultant hyperthyroidism. Unaffected follicular cells (those outside the hyperplastic nodules) undergo atrophy because of the low plasma concentration of TSH (Fig. 12.23, C). Iodine Deficiency. Iodine deficiency, especially during the fetal and neonatal period when the need for thyroid hormones is greatest, is the major cause of diffuse goiter in certain geographic regions—for example, the Pacific Northwest and the Great Lakes region—especially in horses, cattle, small ruminants, and pigs without dietary iodine supplementation. Without sufficient iodine, deficient synthesis of T4 and T3 results in TSH-induced hyperplastic goiter (see Fig. 12.8, A). Grossly, the thyroid gland in hyperplastic goiter is diffusely enlarged and reddened (Fig. 12.24, A; also see Fig. 12.22, A). Histologically, increased vascularity explains the reddening of the gland. Follicles are irregularly enlarged in hyperplastic goiter, but their luminal diameter is diminished because the crowded and hypertrophied (tall columnar) follicular cells form papillary projections into the follicular lumen (E-Fig. 12.14; also see Fig. 12.22, B). Colloid is paler (less eosinophilic) than normal. The periphery of the colloid has a scalloped appearance because of the formation of endocytic resorption vacuoles at the apical surface of the follicular cells (see Fig. 12.8, A). Some hyperplastic follicles may lack apparent colloid or have a collapsed lumen. Despite a robust response to TSH, many iodine-deficient fetuse