Electric Forces PDF
Document Details
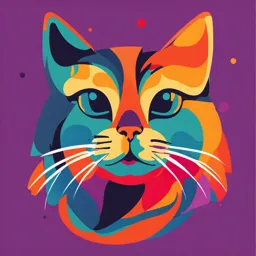
Uploaded by FieryBodhran
European University Cyprus
Dr Irene Polycarpou
Tags
Summary
These lecture notes cover the fundamentals of electric forces, focusing on concepts like electric fields, charges, and polarization. The document also includes examples to illustrate these principles.
Full Transcript
Electric fields Physics for Biomedical Sciences Dr Irene Polycarpou What is the Origin of Electric Charge? Each atom contains a nucleus (protons and neutrons) and electrons. Among them, the electrons are the ones that could be transferred from one atom to another. The SI unit of electric c...
Electric fields Physics for Biomedical Sciences Dr Irene Polycarpou What is the Origin of Electric Charge? Each atom contains a nucleus (protons and neutrons) and electrons. Among them, the electrons are the ones that could be transferred from one atom to another. The SI unit of electric charge is 1 Coulomb [C]. Electric charge is a scalar, and a fundamental quantity of a particle. 𝑄 =𝑛×𝑒 Transfer of electrons during a redox reaction. Bohr’s vs. Electron Cloud Model The electron cloud model uses the basic idea of Bohr’s model, except that the electrons are not found in distinct orbitals but their position can be thought of as in a cloud that has a particular energy. Bohr’s model depicts electrons as particles, while the electron cloud model depicts electrons as both particles and waves, following the principles of quantum mechanics. Figure 1. Two Models of Atomic Structure. (a) In the planetary model, the electrons of helium are shown in fixed orbits, depicted as rings, at a precise distance from the nucleus, somewhat like planets orbiting the sun. (b) In the electron cloud model, the electrons of carbon are shown in the variety of locations they would have at different distances from the nucleus over time. Electric Charges Atoms has charged particles: the protons and the electrons. The charge on proton is positive (+) The charge on electron is negative (-) Charge comes in two types, positive and negative. Electric charge: ✓LIKE charges repel each other. ✓OPOSSITE charges attract each other. Electric Charges Charge in an object comes from an imbalance of protons and electrons in atoms that make up the object. Charge Balance Neutral atoms are made of equal number of positive and negative charges. ✓Neutral carbon has 6 protons, 6 electrons, (& neutrons) Electrons can be stripped off of atoms. ✓Electrons occupy the “vulnerable outskirts” of atoms, aka. the electron cloud Charge usually flows in such a way as to maintain neutrality. ✓Excess positive charge attracts excess negative charge Conservation of Electric Charge Electric charge is conserved — the arithmetic sum of the total charge cannot change in any interaction. Charge is neither created, nor destroyed; it only transfers from one place to another. In physics, charge conservation is the principle that the total electric charge in an isolated system never changes. Example 1 A cat’s fur acts like your hair when you rub a balloon on it. A balloon has picked up -150 μC of charge from Albert, the cat. The resulting opposite charges on the balloon and Albert, cause the balloon to stick. a) How many electrons have been transferred? b) What is the charge on Albert? Example 1 A cat’s fur acts like your hair when you rub a balloon on it. A balloon has picked up -150 μC of charge from Albert, the cat. The resulting opposite charges on the balloon and Albert, cause the balloon to stick. a) How many electrons have been transferred? b) What is the charge on Albert? 𝑒 = −1.60 × 10−19 𝐶 𝑄 −150 × 10−6 𝐶 14 𝐶 a) 𝑛= = = 9.4 × 10 𝑒 −1.60 × 10−19 𝐶 a) Conservation of charge tells us that 𝑄𝐴𝑙𝑏𝑒𝑟𝑡 = +150 μC Formation of Ions The presence of different atoms in objects provides different objects with different electrical properties. An atom may gain or loose free electrons and become charged. Ions – electrically charged particles formed either by loss or gain of electrons. Conductors, isolators & semiconductors Conductors are materials that let electricity flow easily through them. They have low resistance and high conductivity. Metals are examples of conductors. Insulators are materials that do not let electricity flow through them. They have high resistance and low conductivity. Rubber and glass are examples of insulators. Semiconductors are materials that have conductivity between conductors and insulators. They can be used to control the flow of electricity. Silicon and germanium are examples of semiconductors. Conductors, isolators & semiconductors A conduction band is a band of energy levels in a solid that is partially filled with electrons. These electrons are highly mobile and are responsible for electrical conductivity. The conduction band is the band of electron orbitals that electrons can bounce up into from the valence band when energised. At the point when the electrons are in these orbitals, they have enough energy to move freely in the material. This movement of electrons makes an electric current flow. The valence band is the furthest electron orbital of a particle of a particular material in which electrons are involved. Electric Polarization Some materials can become polarized – this means that their atoms rotate in response to an external charge. Electric polarization is a slight relative shift of positive and negative electric charge in opposite directions – separating opposite charges within an object Polarization occurs when an electric field distorts the negative cloud of electrons around positive atomic nuclei in a direction opposite the field. This slight separation of charge makes one side of the atom While there is a separation of charge, somewhat positive and the opposite side somewhat there is NOT an imbalance of charge. negative. When neutral objects become polarized, they are still neutral objects. Electric Polarization IN AN INSULATOR When bringing a charged object near an insulator, electrons are not free to migrate through the material. Instead, they redistribute within the atoms/molecules themselves → their “centers of charge” move. Atom is electrically polarized. Normal atom with the center of the electron When a negative cloud at the charge is brought near positive nucleus. the right, the electron cloud shifts to the left. Centers of positive and Surfaces of materials usually negative charges no look like this, even though longer coincide. there is still zero net charge on the whole object. Electric Polarization Free electrons are free to move CONDUCTORS vs. INSULATORS in a conductor. Neighboring positive wall induces negative charge. Free electrons move towards the wall. Electrons stay with their atom in an insulator, but atoms become polarized. Neighboring positive wall incudes negative charge. Molecules are polarized. Most materials are in-between perfect conductors and insulators. Charging an Object Charging an Object CHARGING BY FRICTION Rubbing two different materials together is the simplest way to give charge. When two uncharged objects are rubbed together, some electrons from one object can move onto the other object. The object that gains electrons becomes negatively charged. Since two objects are made of different materials, their atoms will hold onto electrons with different strengths. As they, the electrons with weaker bonds are “ripped” off one material and collect on the other. Example 2 CHARGING BY FRICTION Materials: Rod of ebonite (very hard, black rubber) Rod of glass Piece of animal fur. Rub a piece of ebonite (very hard, black rubber) across a piece of animal fur. Explain what happens. Example 2 CHARGING BY FRICTION The fur does not hold on to its electrons as strongly as the ebonite. At least some electrons will be ripped off of the fur and stay on the ebonite. Now the fur has a slightly positive charge (it lost some electrons) and the ebonite is slightly negative (it gained some electrons). The net charge is still zero between the two. Remember the conservation of charge. No charges have been created or destroyed, just moved around. Example 3 CHARGING BY FRICTION Rub a piece of glass rod across a piece of silk cloth. Explain what happens. The glass does not hold on to its electrons as strongly as the silk cloth. At least some of the electrons will be ripped off of the glass and stay on the silk cloth. Now the glass has a slightly positive charge (it lost some electrons) and the silk cloth is slightly negative (it gained some electrons). The net charge is still zero between the two. Remember the conservation of charge. No charges have been created or destroyed, just moved around. Example 4 CHARGING BY FRICTION When you exit and re-enter your vehicle while refuelling, there is the potential for sufficient static electricity to build up that a spark can discharge between your body and the fuel nozzle. In rare circumstances, the spark can ignite gasoline vapors around the fill spout, causing a brief flash fire. Example 5 CHARGING BY FRICTION If you are wearing certain shoes and walking on a certain type of carpet you will become charged. In this example electrons move from the shoe to the carpet. When we touch a conducting object that is earthed, electrons flow onto our bodies to neutralise the charge. Charging an Object CHARGING BY FRICTION Friction results in two objects with opposite charges, one negatively charged and one positively charged. The triboelectric series, sometimes called the electrostatic series, is a list that places materials in order according to their tendency to receive or give up negative electrical charges (electrons). By comparing the position of two materials on this list, it can be determined which object becomes negatively charged and which object becomes positively charged. Example 6 CHARGING BY FRICTION A person rubs a rubber balloon on their hair. Referring to the The Triboelectric Series of Common Materials, determine which type of charge is obtained by the balloon and which type of charge is obtained by the hair. Example 6 CHARGING BY FRICTION Rubbing a balloon transfers electrons from the hair to the balloon by friction, resulting in a negative charge on the balloon and a positive charge on the hair. Charging an Object CHARGING BY FRICTION What will determine which material will give electrons? The material that steals electrons has the larger affinity. The electron affinity of an atom or molecule is defined as the amount of energy released when an electron is attached to a neutral atom or molecule in the gaseous state to form a negative ion. In other words, the neutral atom's likelihood of gaining an electron. Charging an Object CHARGING BY FRICTION Insulators can be easily charged by friction as the extra electrons gained CANNOT easily escape. Conductors CANNOT be easily charged by friction as the extra electrons gained can easily escape. Charging an Object CHARGING BY CONDUCTION Charging by conduction allows a neutral object to be charged from another object that is already charged. When the two objects touch, negative charges move from one object to the other until they are distributed evenly between the two objects. Conduction results in two objects that are charged with the same sign. Example 7 CHARGING BY CONDUCTION In the laboratory, an aluminum rod and a styrofoam ball are used. The rod is negatively charged and the ball is neutral. At the moment of contact, the excess negative charges in the rod seek to distribute themselves evenly in each object, because the negative charges repel each other. Some of the excess negative charges move from the rod to the ball. Now, both the rod and the ball are negatively charged. Charging an Object CHARGING BY INDUCTION Charging by induction allows a section of a neutral conducting object to be temporarily charged by placing it in close proximity to a charged object without direct contact. Induction results in a temporarily charged object with a negatively charged section and a positively charged section. Generally, once the charged object is moved away from the neutral object, the latter returns to its original state. The uncharged object does not actual gain charges. Charging by Induction Charging by Induction Example 8 CHARGING BY INDUCTION Charging an Object CHARGING BY INDUCTION Can you charge an object by induction PERMANENTLY? ✓ Yes by grounding! An object is grounded when it is connected to the earth through a conductor. Example 9 CHARGING BY INDUCTION Charging an Object Electric Field An electric field is a region where electric charges experience a force due to the presence of other charges. The direction and strength of an electric field can be represented by electric field lines, which point in the direction that a positive test charge would move. The closer the lines are, the stronger the field. Electric Field Strength The electric field at a point is defined as the force per unit charge exerted on a small positive test charge placed at that point. The unit of electric field is newton per coulomb (N/C) or volt per meter (V/m). Electric Field Strength FOR A POINT CHARGE The electric field due to a point charge is given by the formula, where k is the Coulomb’s constant, q is the charge of the source, and r is the distance from the source. The electric field is inversely proportional to the square of the distance and points radially away from a positive charge and towards a negative charge. Electric Field Strength The electric field due to a collection of charges can be found by vectorially adding the electric fields due to each individual charge. This is known as the superposition principle. The electric field inside a conductor is zero, because any excess charge on a conductor distributes itself on the surface to minimize the repulsive forces. The electric field outside a conductor is perpendicular to the surface and proportional to the surface charge density. Charge tends to accumulate in greater numbers at locations of greatest curvature. Electric Field If we know the electric field, we can calculate the force on any charge: The direction of the force depends on the sign of the charge – in the direction of the field for a positive charge, opposite to it for a negative one. Example 10 A −4 × 10−12 𝐶 charge Q is placed at the origin. What is the magnitude and direction of the electric field produced by Q if a test charge were placed at 𝑥 = −0.2 𝑚 ? Example 10 A −4 × 10−12 𝐶 charge Q is placed at the origin. What is the magnitude and direction of the electric field produced by Q if a test charge were placed at 𝑥 = −0.2 𝑚 ? −12 kQ ( 4 x10 ) E = 2 = 8.99 x10 9 r.2 2 Emag = 0.899 N/C Edir = Towards Q to the right. Remember, our equations will only give us MAGNITUDE. And the electric field LEAVES POSITIVE and ENTERS NEGATIVE. Electric Field Lines The electric field can be represented by field lines. These lines start on a positive charge and end on a negative charge. Electric field lines are a convenient way of visualizing the electric field. Electric field lines: I. Point in the direction of the field vector at every point II. Start at positive charges or infinity III. End at negative charges or infinity IV. Are more dense where the field is stronger. The number of field lines starting (ending) on a positive (negative) charge is proportional to the magnitude of the charge. The electric field is stronger where the field lines are closer together. Electric Field Lines The charge on the right is twice the magnitude of the charge on the left (and opposite in sign), so there are twice as many field lines, and they point towards the charge rather than away from it. Electric Field Lines An electric dipole is a pair of equal and opposite electric charges that are separated by a small distance, creating a net polarity and an electric field. The electric dipole moment is a vector quantity that measures the strength and direction of the dipole, pointing from the negative charge to the positive charge. Electric Field Lines Note that, while the lines are less dense where the field is weaker, the field is not necessarily zero where there are no lines. In fact, there is only one point within the figures below where the field is zero – can you find it? Electric Field Lines Along the line connecting the charges, the left charge contributes a rightward electric field, and the right charge contributes a leftward electric field. They are equidistant so they both have magnitude Electric field is zero in that point because the sum of electric field vectors have same intensity and direction, but are opposite. That point is halfway between two like charges. Coulomb’s Law Experiment shows that the electric force between two charges is proportional to the product of the charges and inversely proportional to the distance between them. Coulomb’s law gives the force between two point charges: The force is along the line connecting the charges, and is attractive if the charges are opposite, and repulsive if the charges are like. Coulomb’s Law The forces on the two charges are action-reaction forces. 1. This means that the electric force that one charge exerts on another charge is equal in magnitude and opposite in direction to the electric force that the other charge exerts on the first charge. This is consistent with Newton’s third law of motion, which states that for every action, there is an equal and opposite reaction. 2. This also means that electric force is a conservative force, which means that the work done by the electric force on a charge depends only on the initial and final positions of the charge, not on the path taken. This means that the electric potential energy of a system of charges is well-defined and conserved. Coulomb’s Law If there are multiple charges, the forces are added by superposition. Note: Coulomb’s law is stated in terms of point charges, but it is also valid for spherically symmetric charge distributions, as long as the distance is measured from the center of the sphere. Coulomb’s Law ILLUSTRATED Like charges repel Unlike charges attract Solving Problems with Coulomb’s Law & Vectors Coulomb’s law applies to point charges (and also spherically symmetric charge distributions). Superposition: for multiple point charges, the forces on each charge from every other charge can be calculated and then added as vectors. © 2014 Pearson Education, Inc. Solving Problems with Coulomb’s Law & Vectors The net force on a charge is the vector sum of all the forces acting on it. © 2014 Pearson Education, Inc. Example 11 Two point charges, 𝑞1 = 2.0 𝜇𝐶 and 𝑞2 = −4.0 𝜇𝐶 , are placed 0.5 𝑚 apart. What is the magnitude and direction of the electrostatic force between them? Example 11 Two point charges, 𝑞1 = 2.0 𝜇𝐶 and 𝑞2 = −4.0 𝜇𝐶 , are placed 0.5 𝑚 apart. What is the magnitude and direction of the electrostatic force between them? 2.0 × 10 −6 𝐶 × −4.0 × 10−6 𝐶 𝐹 = 8.99 × 109 𝑁𝑚2 /𝐶 2 (0.5𝑚)2 𝐹 = −7.19 × 10−2 𝑁 The negative sign indicates that the force is attractive, since the charges have opposite signs. Therefore, the magnitude of the force is 7.19 × 10−2 𝑁, and the direction is towards the other charge. Electric Current Electric current is simply the flow of charge. Electrons flowing in a wire constitute a current. Measured in Coulombs per second, or Amperes Colloquially, Amp (A) Refers to amount of charge crossing through cross-sectional area per unit time. Electrons have a charge of – 1.6 × 10−19 Coulombs So (negative) one Coulomb is 6 × 1018 electrons One amp is 6 × 1018 electrons per second Subtle gotcha: electrons flow in direction opposite to current, since current is implicitly positive charge flow, but electrons are negative. Voltage, Current and Power One Volt is a Joule per Coulomb (J/C). One Amp of current is one Coulomb per second (C/s). If I have one volt (J/C) and one amp (C/s), then multiplying gives Joules per second (J/s) → This is power. J/s = Watts So the formula for electrical power is just: 𝑃 = 𝑉 × 𝐼 = 𝑣𝑜𝑙𝑡𝑎𝑔𝑒 × 𝑐𝑢𝑟𝑟𝑒𝑛𝑡 Example 13 A patient has a cardiac pacemaker that delivers a 120 V pulse to the heart. The resistance of the heart tissue is 100 Ω. How much power does the pacemaker consume? Example 13 A patient has a cardiac pacemaker that delivers a 120 V pulse to the heart. The resistance of the heart tissue is 100 Ω. How much power does the pacemaker consume? 𝑉 𝑃 =𝑉×𝐼 𝐼= 𝑅 𝑉 2 (120 𝑉)2 𝑃= = 𝑅 100 𝛺 𝑃 = 144 𝑊 The pacemaker consumes 144 watts of power. Electric Field and Conductors What would happen if I put a very big charge inside an ungrounded hollow conducting sphere like this image? The charges inside the conductor are supposed to rearrange. So in that case, the electrons will move towards the inner surface and it creates a field in the opposite direction that somewhat cancels the existing field. The net charge on a conductor is on its surface. Electric Field and Conductors The electric field is always perpendicular to the surface of a conductor – if it weren’t, the charges would move along the surface. The electric field is stronger where the surface is more sharply curved. 67 Electric Forces in Molecular Biology: DNA Structure The DNA molecule is a double helix: The A-T and G-C nucleotide bases attract each other through electrostatic forces. © 2014 Pearson Education, Inc. Electric Forces in Molecular Biology: DNA Structure In DNA, there are four different bases: adenine (A) and guanine (G) are the larger purines. Cytosine (C) and thymine (T) are the smaller pyrimidines. How do they match up? - Thymine and adenine - Cytosine and guanine Electric Forces in Molecular Biology: DNA Structure The importance of electric forces in human cell Electric forces are non-contact forces that are a result of electric charges as protons and electrons within atoms, and molecules and compounds. Electric forces are able to act over distance. These forces are arguably the most important forces as they direct all the processes that take place within the living biological cell. The importance of electric forces in human cell Electric forces are important forces as they direct many processes that take place within the living biological cell. The human cell is composed of many types of molecules and compounds. The majority of the human cell is composed of cytoplasm. Cytoplasma is a fluid that contains mostly water. The biological cell is composed mostly of water. In a way we can think of the human cell as a sea of water molecules which are moving with random and rapid motion. They collide with each other and they are exchanging energy. Source: AkLectures, 2019, Available at: http://www.aklectures.com One thing that organizes this motion of molecules within the human cells are electrical forces. Inside the cell all the different types of processes that takes place, occur as a result of two principles: 1) Random and rapid motion of the molecules and atoms found inside the plasma 2) Electrical forces which act to organize, arrange and ultimately carry out the processes. Electric forces govern all the different types of process such as: - Cell Respiration - DNA structure and replication - Translation Source: AkLectures, 2019, Available at: http://www.aklectures.com Example --- Cell Respiration Cell Respiration: process within the mitochondria During Cell Respiration ATP molecules are produced ATP molecules store energy which is used to carry out mechanical functions (e.g. moving our hands) The production of ATP is a result of oxygen. Oxygen is able to accept electrons. This is due to the number of protons that makes: Oxygen to be highly electronegative. Therefore as a result of electric forces THE OXYGEN is able to accept electrons. 74 Electric Forces in Molecular Biology: DNA Structure The DNA molecule is a double helix: The A-T and G-C nucleotide bases attract each other through electrostatic forces. Electric Forces in Molecular Biology: DNA Structure In DNA, there are four different bases: adenine (A) and guanine (G) are the larger purines. Cytosine (C) and thymine (T) are the smaller pyrimidines. How do they match up? - Thymine and adenine - Cytosine and guanine Electric Forces in Molecular Biology: DNA Structure The importance of electric forces in human cell Electric forces are non-contact forces that are a result of electric charges as protons and electrons within atoms, and molecules and compounds. Electric forces are able to act over distance. These forces are arguably the most important forces as they direct all the processes that take place within the living biological cell. Remember that: Electronegativity is a chemical property that describes the tendency of an atom to attract a shared pair of electrons towards itself. Example --- DNA structure Genetic information is passed down from parent to child in the form of DNA, which is composed of genes that code for proteins DNA is a double-helix structure that is held together by electric forces between pairs of nucleotides: - Thymine and adenine - Cytosine and guanine On these molecules we have different atoms and these different atoms have different electronegativity. Oxygen is more electronegative than carbon and that means it pulls electrons closer to the oxygen and that develops a small negative charge on this oxygen. Nitrogen is more electronegative than the H atom that is attached to. Therefore, the Nitrogen takes electrons more rapidly than the H atom will and that means a small positive charge will developed on the H atom. Source: AkLectures, 2019, Available at: http://www.aklectures.com Because Thymine and Adenine can get close enough the electric forces between these two nucleotides will be high enough for them to be bond. Remember that Coulomb force is related with distance: Since the Coulomb force drops with distance, the distances between the base pairs must be small enough that the electrostatic force is sufficient to hold them together The distance separating the two strands that make up the DNA structure is about 1 nm, while the distance separating the individual atoms within each base is about The reason that Cytosine and guanine can 0.3 nm. not get close enough is because the shapes are not correct. The Thymine and adenine have the correct shape to get close enough. So that the distance is small and the ELECTRICAL force is high. Adenine is able to fit close enough only to thymine. As a result of this proximity the electrical force is high enough to hold them. Example --- DNA structure All these electrical forces (hydrogen bonds) hold the double helix together. it is the electrostatic force that not only holds the molecule together but gives the molecule structure and strength. 82 DNA Replication Replication: DNA is in a “soup” of A, C, G, and T in the cell. During random collisions, A and T will be attracted to each other, as will G and C; other combinations will not. 83 Electrocardiogram (ECG or EKG) The electrocardiogram detects heart defects by measuring changes in potential on the surface of the heart. Electrocardiogram (ECG or EKG) The heart is a muscle that contracts and pumps blood. It consists of specialized muscle cells called cardiac myocytes. The contraction of these cells is initiated by electrical impulses, known as action potentials. Unlike skeletal muscles which have to be stimulated by the nervous system, the heart generates its OWN ELECTRICAL STIMULATION. The nervous system can make the heartbeats go faster or slower, but cannot generate them. A heart can keep on beating even when taken out of the body. The impulses start from a small group of myocytes called the PACEMAKER cells which constitute the cardiac conduction system. The SA node is the primary pacemaker of the heart. It initiates all heartbeats and controls heart rate. The SA generates action potentials that spread through the contractile myocytes of the atria. The myocytes are connected by gap junctions, which form channels that allow ions to flow. This enables electrical coupling of neighboring cells. An action potential in one cell triggers an action potential in its neighbor cell and the signals propagate. The impulse reach the AV node, slow down a little to allow the atria to contract and then follow the conduction pathway and spread through the ventricular myocytes. Cells are polarized, meaning that there there is an electrical voltage across the cell membrane. In a resting cell the cell is more negative on the inside that the outside. At the resting state: More Potassium (K+) ions inside the cell An action potential is a brief REVERSAL of electric polarity of the cell. An action potential is a brief REVERSAL of electric polarity of the cell. When membrane voltage increases and becomes less negative, the cell is LESS POLARIZED (depolarized). When membrane voltage decreases and becomes more negative, the cell is RE-POLARIZED 93 94 Electrocardiogram (ECG or EKG) Because a large number of cells in the heart rhythmically depolarize, with each contraction, we can record the electrical changes on our body surface through. This electrical trace is known as an electrocardiogram. ECG: Works by measuring changes in electric field as heart pumps Electrodes are placed at several positions on the body and the change in voltage measured with time 95 Electrocardiogram (ECG or EKG) Interior of Heart muscle cells negatively charged at rest Called “polarisation” Potassium (K+) ions leak out, leaving interior –ve Depolarisation occurs just prior to contraction: Sodium (Na+) ions enter cells Occurs in waves across the heart Re-polarisation restores –ve charge in interior -- ++ ++++ ----- -- ++ Polarisation Depolarisation 96 DIASTOLE - filling SYSTOLE - ejection Depolarisation occurs just prior to contraction: Sodium (Na+) ions enter cells 97 Typical wave of an Electrocardiogram An ECG has a typical shape which contains a few remarkable peaks. The peaks (positive as well as negative) are marked by the letters P, Q, R, S and T, each corresponding to a certain mechanic action of the heart. The letters peaks of P, Q and R are often referred to as the PQR-complex. 98 Typical wave of an Electrocardiogram https://www.youtube.com/watch?v=v7Q9BrNfIpQ 3 distinct waves are produced during cardiac cycle P wave caused by atrial depolarization QRS complex caused by ventricular depolarization T wave results from ventricular repolarization DNA itself is highly negatively charged All these electrical forces (hydrogen bonds) hold the double helix together. it is the electrostatic force that not only holds the molecule together but gives the molecule structure and strength. Phosphate groups in the DNA backbone carry negatively-charged oxygen molecules giving the phosphate-sugar backbone of DNA an overall negative charge. ELECTROENCEPHALOGRAM (EEG) Electroencephalography, technique for recording and interpreting the electrical activity of the brain. The nerve cells of the brain generate electrical impulses that fluctuate rhythmically in distinct patterns. EEG is a medical device for analyzing the electrical activity of the brain and detect abnormalities such as (epilepsy or Alzheimer) It was used in humans by Hans Berger. In 1929 German scientist Hans Berger published the results of the first study to employ an electroencephalograph, an instrument that measures and records these brain-wave patterns. The recording produced by such an instrument is called an electroencephalogram, commonly abbreviated EEG. EEG: The basic unit of communication in the nervous system is the nerve cell (neuron). There are billions of nerve cells in the brain that produce electric signals (small electric signals). The neurons are negative when they are at rest and become positive when they synapse. EEG can record this change by electrodes The general mechanism of EEG is picking up the charge of electric potentials The signals are transferred from the electrodes and processed to be appeared in a screen. EEG waves are categorized according their characteristics (e.g. frequency, amplitude) Revision Revision Revision Revision Revision Revision