Earthquake Engineering PDF
Document Details
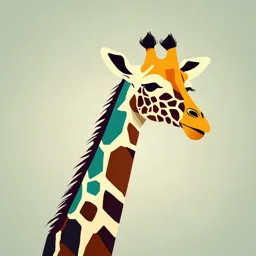
Uploaded by ImmenseCarbon
SMIT
Tags
Summary
This document is a presentation on earthquake engineering covering plate tectonics, seismic waves, and earthquake-resistant design. It discusses various aspects of earthquakes, including their causes, effects, and the design principles for earthquake-resistant structures.
Full Transcript
Elements of earthquake Engineering Department of Civil Engineering, SMIT Engineering Seismology Seismology is the study of the generation, propagation and measurement of seismic waves through earth and the sources that generate them. Fig. Earth’s Interio...
Elements of earthquake Engineering Department of Civil Engineering, SMIT Engineering Seismology Seismology is the study of the generation, propagation and measurement of seismic waves through earth and the sources that generate them. Fig. Earth’s Interior Fig. Earth’s Interior Engineering Seismology Plate Tectonics The lithosphere/ Crust is broken into seven large (and several smaller) segments called Plates Engineering Seismology Plate Tectonics The Earth’s crust is divided into 12 major plates which are moved in various directions. This plate motion causes them to collide, pull apart, or scrape against each other. Each type of interaction causes a characteristic set of Earth structures or “tectonic” features. The word, tectonic, refers to the deformation of the crust because of plate interaction. Plate Tectonics The Earth's crust is divided into 12 major tectonic plates, which move in different directions due to the forces of plate tectonics. The names of these major plates are: 1. African Plate 7. Pacific Plate 2. Antarctic Plate 8. Nazca Plate 9. Cocos Plate 3. Eurasian Plate 10. Caribbean Plate 4. Indo-Australian Plate (sometimes 11. Arabian Plate considered as two plates: the Indian 12. Philippine Sea Plate Plate and the Australian Plate) 5. North American Plate 6. South American Plate World Plates Types of plate boundary Divergent Convergent Transform Plate Boundaries 1. Spreading Ridges Spreading ridges or divergent boundaries are areas along the edges of plates move apart from each other. This is the location where the less dense molten rock from the mantle rises upwards and becomes part of crust after cooling. Highest rate of spreading or expansion between plates is found to occur near Pacific Ocean ridges and the lowest rate of spreading occurs along mid-Atlantic ridges. Generally, spreading ridges are located beneath the oceans. A few areas where the spreading occurs along the continental mass are East African rift valley and Iceland. Plate Boundaries Key Features of Spreading Ridges Mid-Ocean Ridges: The most prominent feature of spreading ridges is the mid-ocean ridge system, the largest continuous mountain range on Earth, extending over 65,000 kilometers (40,000 miles) through all major oceans. Example: The Mid-Atlantic Ridge, which separates the Eurasian Plate from the North American Plate and the African Plate from the South American Plate. Seafloor Spreading: This process of creating new oceanic crust and pushing older crust away is called seafloor spreading. It helps explain the movement of tectonic plates and the gradual widening of ocean basins. Rift Valleys: At the center of the spreading ridge, a deep rift valley can form due to the pulling apart of the plates. These valleys are often the site of volcanic activity, as magma rises to fill the gap. Example: The East African Rift, a continental example of a rift valley, though most rift valleys are found at mid-ocean ridges. Hydrothermal Vents: Along spreading ridges, seawater seeps into the cracks in the newly formed crust, gets heated by magma, and is expelled through hydrothermal vents. These vents support unique ecosystems, with organisms relying on chemosynthesis rather than photosynthesis for energy. Divergent boundary Plate Boundaries 2. Subduction Boundary A subduction boundary is a type of convergent plate boundary where one tectonic plate moves under another and is forced to sink into the Earth's mantle. This process typically involves an oceanic plate being subducted beneath a continental plate or another oceanic plate. The sinking plate is usually denser than the overriding plate, causing it to dive beneath. Subduction is a critical part of the Earth's tectonic cycle, leading to various geological phenomena such as earthquakes, volcanic activity, and the formation of deep ocean trenches. Plate Boundaries Fig. Subduction Boundary Plate Boundaries Key Features of Subduction Boundaries Deep Ocean Trenches: The deepest parts of the ocean, formed where the subducting plate bends and sinks into the mantle. Example: Mariana Trench. Volcanic Activity: As the subducted plate melts, magma rises and forms volcanic arcs, either as volcanic mountains (on continental crust) or volcanic islands (on oceanic crust). Example: Cascade Range, Japan. Earthquakes: Subduction zones are highly seismically active due to the immense pressure and friction caused by the plates interacting. Deep and powerful earthquakes, known as megathrust earthquakes, often occur along these zones. Example: 2011 Tōhoku Earthquake in Japan. Mountain Building: In cases where continental and oceanic plates converge, subduction can push up mountain ranges. Example: The Andes Mountains. Plate Boundaries Plate Boundaries 3. Collision Boundaries When two plates with continental lithosphere collide, subduction ceases, and a mountain range is formed by squeezing together and uplifting the continental crust on both plates. The Himalayan Mountains between India and China were formed in this way. Convergent Boundary Plate Boundaries Key Features of Collision Boundaries Mountain Building (Orogeny): The most significant result of a collision boundary is the formation of mountain ranges. When two continental plates collide, the crust buckles and is pushed upward, forming towering mountains. This process is called orogeny. Example: The Himalayas, the highest mountain range in the world, formed by the ongoing collision between the Indian Plate and the Eurasian Plate. Earthquakes: The collision of massive continental plates produces large amounts of stress and pressure, resulting in powerful earthquakes. These earthquakes are usually shallow but can be highly destructive due to the enormous energy released. Example: The Himalayas and surrounding regions frequently experience earthquakes due to the ongoing collision of the Indian and Eurasian plates. Thrust Faults and Folds: As the plates collide, the crust may fracture and fold, creating thrust faults (where one section of the Earth's crust is pushed over another) and large-scale folds in the rock layers. This results in complex and often deformed geological structures. Example: The folds and thrust faults in the Appalachian Mountains, which were formed during the ancient collision of North America with another landmass. No Volcanic Activity: Unlike subduction zones, collision boundaries do not typically result in volcanic activity because there is no sinking of plates into the mantle to generate magma. Plate Boundaries 4. Transform Ridge Transform boundaries occur along the plate margins where two plate moves past each other without destroying or creating new crust. Transform Boundary Fig. View of the San Andreas transform fault Plate Boundaries Key Geological Features of Transform Boundaries Fault Lines: The key feature of a transform boundary is the presence of fault lines where the plates slide past each other. These fault lines can be several kilometers wide and are often the site of repeated earthquakes. No Crust Creation or Destruction: Unlike at divergent boundaries (where new crust is formed) or convergent boundaries (where crust is destroyed), transform boundaries neither create nor destroy Earth's crust. The movement is purely lateral, with plates grinding past each other. Offset Geological Features: Over time, the movement along transform faults can offset natural features like rivers, valleys, and mountain ranges. The offset of these features provides evidence for the horizontal movement at these boundaries. Earthquake Activity: Transform boundaries are known for producing powerful, shallow earthquakes. Since the plates slide past each other, they often become locked due to friction, and when they finally release, the result is a large seismic event. CAUSES OF EARTHQUAKE Here are the primary causes of earthquakes: 1. Tectonic Plate Movements Faulting: Earthquakes often occur along faults, which are fractures in the Earth's crust where blocks of rock have moved relative to each other. When stress builds up along a fault line, it can be released suddenly, causing an earthquake. Plate Boundaries: The Earth's lithosphere is divided into tectonic plates that float on the semi-fluid asthenosphere. Interactions at plate boundaries—such as convergent (colliding), divergent (moving apart), and transform (sliding past each other)—are primary causes of earthquakes. 2. Volcanic Activity Magma Movement: Earthquakes can occur due to the movement of magma within the Earth's crust. As magma rises towards the surface, it can create pressure and fractures, causing volcanic earthquakes. Volcanic Eruptions: The buildup of pressure from magma and gases within a volcano can trigger seismic activity before, during, and after an eruption. CAUSES OF EARTHQUAKE 3. Human Activities Mining Operations: Extracting minerals, coal, or other resources from the Earth can cause changes in stress and lead to seismic activity, including induced earthquakes. Reservoir-Induced Seismicity: The filling of large reservoirs behind dams can increase pressure on faults and induce earthquakes. The weight of the water can affect the stress along geological faults. Geothermal Energy Extraction: The extraction of geothermal fluids from underground reservoirs can alter pressure conditions and induce seismic activity. 4. Stress Accumulation and Release Elastic Rebound Theory: According to this theory, stress accumulates along faults due to tectonic forces. When the stress exceeds the strength of the rocks, it is released suddenly, causing an earthquake. This release results in the rocks snapping back to their original shape, leading to seismic waves. CAUSES OF EARTHQUAKE 5. Isostatic Rebound Post-Glacial Rebound: After the melting of large ice sheets (such as those from the last Ice Age), the Earth's crust slowly adjusts to the reduced weight. This process, known as isostatic rebound, can induce earthquakes as the crust rebalances. 6. Collapse Earthquakes Subsurface Caves and Caverns: The collapse of underground caves or large voids can cause surface earthquakes. When the support structures beneath the Earth's surface fail, it can result in a sudden ground shake. 7. Fault Slippage Fault Movement: Sudden slippage along geological faults due to accumulated stress causes the ground to shake. This can occur along pre-existing fault lines or new faults that develop due to tectonic forces. Magnitude and Intensity Magnitude Intensity Magnitude represents the size Intensity represents the effects of the earthquake, but not of an earthquake: the shaking necessarily the damage or and damage at different shaking level locations Decimal value (e.g., 6.7). Only Determined from observations one value is used for a single of shaking and damage earthquake Roman numerals from I to X Described by the “Richter are used. The value varies scale”, though “energy” depending on location magnitude is now generally The Modified Mercalli used. Intensity Scale is used Depends on the energy released. Magnitude and Intensity Seismic Zones in India Seismic Zoning of India India is divided into the following four seismic zones: 1. Zone II (Low Seismic Risk) 2. Zone III (Moderate Seismic Risk) 3. Zone IV (High Seismic Risk) 4. Zone V (Very High Seismic Risk) Seismic Zonation Map Fig. Seismic Map IS1893-2016 1. Zone II – Low Seismic Risk Description: This zone experiences the least seismic activity and is considered the safest in terms of earthquake risks. It is unlikely to experience severe earthquakes, and the probability of significant damage is low. Areas Covered: Parts of central and southern India, such as Karnataka, Tamil Nadu, Andhra Pradesh, and parts of Madhya Pradesh and Maharashtra. Maximum Expected Intensity: Earthquakes in Zone II typically have an intensity of less than 6 on the Modified Mercalli Intensity (MMI) scale, meaning minor or no structural damage. 2. Zone III – Moderate Seismic Risk Description : This zone has a moderate risk of earthquakes, and seismic activity is more frequent compared to Zone II. Structures in these areas should be designed to withstand moderate earthquakes. Areas Covered: Regions such as Kerala, parts of Uttar Pradesh, Punjab, Rajasthan, Maharashtra, and Gujarat. Major cities like Mumbai, Chennai, and Kolkata also fall in this zone. Maximum Expected Intensity: Intensity ranges from 6 to 7 on the MMI scale, which could cause slight to moderate damage to well-constructed buildings and more significant damage to poorly constructed structures. 3. Zone IV – High Seismic Risk Description: Areas in Zone IV experience significant seismic activity and are prone to major earthquakes. Strict building codes are enforced to reduce the impact of potential seismic events. Areas Covered: The Himalayan region, parts of Delhi, Jammu & Kashmir, Himachal Pradesh, the northern parts of Punjab, Haryana, and parts of Bihar and West Bengal (including Delhi, Amritsar, Ludhiana, Patna). Maximum Expected Intensity: Earthquakes in this zone can reach intensities of 7 to 8 on the MMI scale, capable of causing serious structural damage, collapsing poorly constructed buildings, and affecting infrastructure. 4. Zone V – Very High Seismic Risk Description: Zone V is the most seismically active region of India, experiencing the highest intensity of earthquakes. The potential for devastating earthquakes is significant, and the region requires the strictest adherence to seismic-resistant construction standards. Areas Covered: The northeastern states (Assam, Arunachal Pradesh, Manipur, Meghalaya, Mizoram, Nagaland, and Tripura), the northern parts of Bihar, parts of Jammu & Kashmir, and Andaman and Nicobar Islands. This zone also includes parts of Uttarakhand and the western Himalayas. Maximum Expected Intensity: Intensity levels in Zone V can exceed 8 on the MMI scale, leading to catastrophic damage, with large-scale structural collapse and loss of life, especially in poorly designed or unreinforced buildings. Terminology Hypocenter or Focus: The place of origin of the earthquake in the interior of the earth is known as focus or origin or centre or hypocenter. Epicenter: The place on the earth's surface, which lies exactly above the centre of the earthquake, is known as the 'epicenter’. Focal Depth of an earthquake refers to the depth at which the earthquake originates below the Earth's surface. It is the vertical distance between the Earth's surface and the focus (hypocenter), which is the point Terminology 1. Hypocentral Distance: The hypocentral distance refers to the distance between the hypocenter (also known as the focus) of an earthquake and a specific point on the Earth's surface. The hypocenter is the point inside the Earth where the earthquake originates. The hypocentral distance takes into account both the depth of the earthquake and the horizontal distance from the hypocenter to the observation point. 2. Epicentral Distance: The epicentral distance is the horizontal distance between the epicenter of an earthquake and a specific location on the Earth's surface. The epicenter is the point directly above the hypocenter on the Earth's surface. Key Difference: Hypocentral Distance includes the vertical depth of the earthquake, whereas Epicentral Distance only refers to the horizontal surface distance from the epicenter to a point on the surface. Faults The term fault is used to describe a discontinuity within rock mass, along which movement had happened in the past. Plate boundary is also a type of fault. Lineaments are mappable linear surface features and may reflect subsurface phenomena. A lineament could be a fault, a joint or any other linear geological phenomena. Most faults produce repeated displacements over geologic time. Movement along a fault may be gradual or sometimes sudden thus, generating an earthquake. Faults Earthquake Terminology Seismic Waves Seismic Waves P-Type Wave These are known as primary waves, push-pull waves, longitudinal waves, compressional waves, etc. These waves propagate by longitudinal or compressive action, which mean that the ground is alternately compressed and dilated in the direction of propagation. P waves are the fastest among the seismic waves and travel as fast as 8 to 13 km per second. Therefore, when an earthquake occurs, these are the first waves to reach any seismic station and hence the first to be recorded. The P waves resemble sound waves because these too are compressional or longitudinal waves in nature. Hence, the particles vibrate to and fro in the direction of propagation (i.e. longitudinal particle motion). These S-Type Waves Seismic Waves These are also called shear waves, secondary waves, transverse waves, etc. Compared to P waves, these are relatively slow. These are transverse or shear waves, which mean that the ground is displaced perpendicularly to the direction of propagation, Figure 1.16. In nature, these are like light waves, i.e., the waves move perpendicular to the direction of propagation. Hence, transverse particle motion is characteristic of these waves. These waves are capable of traveling only through solids. If the particle motion is parallel to prominent planes in the medium they are called SH waves. On the other hand, if the Seismic Waves Surface Waves Surface waves are the slowest among the seismic waves. Therefore, these are the last to be recorded in the seismic station at the time of occurrence of the earthquake. They travel at the rate of 4 to 5 km per second. Complex and elliptical particle motion is characteristic of these waves. These waves are capable of travelling through solids and liquids. They are complex in nature and are said to be of two kinds, namely, Raleigh waves and Love waves. The Rayleigh surface waves are tension- compression waves similar to the P- waves expect that their amplitude diminishes with distance below the surface of the ground. Similarly, the Love waves are the counterpart of the “S” body waves; they are shear waves that diminishes rapidly with distance Effects of Earthquake Effects of Earthquake Earthquakes induced Tsunamis Expressway collapse Bridge damage due to recent earthquakes Earthquakes induced landslide Effects of Earthquake Fig.: Damaged building in Singtam, East Sikkim Effects of Earthquakes on Structures Ground Shaking: The primary effect of earthquakes is ground shaking, which causes vibrations that travel through the structure. The intensity of shaking can lead to cracking, tilting, or collapse of buildings. Foundation Failure: The shaking can destabilize the foundation of structures, leading to settlement, cracking, or complete failure, especially in areas with poor soil conditions. Structural Cracking and Deformation: Earthquakes cause bending, stretching, and compression of structural elements (beams, columns, walls), leading to cracks, permanent deformations, or even structural failure. Resonance: If the frequency of seismic waves matches the natural frequency of the structure, it can amplify the motion (resonance), causing excessive swaying and severe damage. Collapse of Walls and Roofs: Non-reinforced masonry walls and roofs are particularly vulnerable to collapse under strong seismic forces, causing significant structural damage and potential fatalities. Effects of Earthquakes on Structures Torsional Movements: Asymmetric buildings can experience torsional motion (twisting), which can weaken the structure and cause severe localized damage, particularly to the corners and edges. Shear Failures: Seismic forces induce shear stress, which can cause walls, beams, and columns to fail by cracking or splitting horizontally, particularly in poorly designed or reinforced structures. Liquefaction: In areas with loose, water-saturated soils, seismic shaking can cause the soil to behave like a liquid, leading to the sinking or tilting of buildings as their foundations lose stability. Pounding between Adjacent Buildings: When closely spaced buildings sway during an earthquake, they can collide (pound) against each other, causing significant structural damage at points of contact. Buckling of Columns: Vertical support columns can buckle under the compressive forces generated by an earthquake, leading to partial or complete collapse of the structure. Effects of Earthquakes on Structures Failure of Building Facades: External cladding, glass, and decorative elements can detach and fall during strong shaking, leading to exterior damage and posing a risk to people below. Damaged Lifelines: Earthquakes can damage essential structural systems like elevators, staircases, and electrical or plumbing systems, reducing the functionality and safety of the building. Roof Displacement and Collapse: Large roofs, particularly flat or unreinforced ones, are susceptible to displacement or collapse due to uneven forces applied during an earthquake. Soft Story Collapse: Buildings with a "soft story" (such as parking garages or large windows on the ground floor) are highly vulnerable to collapse as these levels often lack the stiffness to withstand seismic forces. Failure of Bridges and Overpasses: Earthquakes can cause the collapse of bridges and overpasses due to shaking, displacement of supports, or soil liquefaction, disrupting transport networks. Damage to Dams and Reservoirs: Earthquakes can weaken or breach dams and reservoirs, causing flooding and further structural damage downstream. IS CODES FOR EARTHQUAKE RESISTANT DESIGN 1. IS 1893: Criteria for Earthquake Resistant Design of Structures IS 1893 (Part 1): 2016 - "General Provisions and Buildings": This part provides guidelines for the general provisions, seismic design parameters, and considerations for buildings. IS 1893 (Part 2): 2014 - "Industries Structures": This part addresses the seismic design requirements specific to industrial structures. IS 1893 (Part 3): 2014 - "Bridge and Retaining Walls": This part deals with the seismic design criteria for bridges and retaining walls. IS 1893 (Part 4): 2015 - "Foundations": This part covers the seismic considerations for foundation design. 2. IS 13920: 2016 - "Ductile Design and Detailing of Reinforced Concrete Structures Subjected to Seismic Forces" This code provides detailed guidelines for the ductile design and detailing of reinforced concrete structures to ensure they can withstand seismic forces and exhibit ductility. 3. IS 4326: 1993 - "Code of Practice for Earthquake Resistant Design and Construction of Buildings" This is an earlier standard that provides guidelines for earthquake-resistant design and construction practices, which have been updated and replaced by IS 1893. Earthquake Resistant Design We don’t need earthquake-proof buildings that will not get damaged even during the strong earthquake -Instead, earthquake resistant building. such buildings resist the effects of ground shaking, although they may get damaged severely but would not collapse during the strong earthquake. Thus, safety of people and contents is assured in earthquake- resistant buildings, and thereby a disaster is avoided. This is a major objective of seismic design codes throughout the world. DESIGN PHILOSOPHY a)Under minor shaking, structural members should not be damaged; however, building parts that do not carry load may sustain repairable damage. DESIGN PHILOSOPHY b) Moderate Shaking: Structure should be able to resist occasional moderate ground shaking without significant damage DESIGN PHILOSOPHY c) Major Shaking: Structure should be able to resist major earthquakes without collapse OBJECTIVES OF EQ RESISTANT DESIGN The primary aim of earthquake-resistant design is to ensure that structures can withstand seismic events with minimal damage and loss of life. Below are the main objectives: 1. Life Safety: The most crucial objective is to protect human life by preventing structural collapse during an earthquake. Even in severe seismic events, buildings should remain intact enough to allow safe evacuation. 2. Structural Integrity: Ensure that the building or structure maintains its overall stability during an earthquake, with minimal structural damage. 3. Damage Control: Minimize damage to non-structural components, such as partitions, windows, and mechanical systems, so that repair costs and downtime after the earthquake are reduced. OBJECTIVES OF EQ RESISTANT DESIGN 4. Resilience: Enable the structure to absorb and dissipate seismic energy through flexibility, reducing the risk of complete failure. 5. Functionality: In critical infrastructure (e.g., hospitals, emergency response centers), the design ensures the building remains operational after an earthquake. 6. Economic Feasibility: Design structures in a cost-effective manner that balances safety, performance, and cost, without excessive over-engineering. 7. Durability: Ensure that the materials and design can endure repeated or prolonged seismic activity over the building’s lifespan. Earthquake-Resistant Structures Earthquake-resistant structures are designed to withstand seismic forces and minimize damage during an earthquake. The goal of such structures is to ensure the safety of occupants and prevent the collapse of the building, even under intense shaking. Key Features of Earthquake-Resistant Structures:. Ductility: Materials and structural elements should have the ability to deform without breaking. This allows the building to absorb and dissipate seismic energy.. Base Isolation: A technique where the building is separated from the ground using bearings or isolators, reducing the transmission of seismic forces to the structure. Earthquake-Resistant Structures Figure: Conventional system VS base isolation system Fig.: Ductility of building structure Earthquake-Resistant Structures 3. Lateral Load Resistance: Structures are designed with components like shear walls, braced frames, and moment-resisting frames to resist horizontal forces generated by earthquakes. 4. Symmetry and Regularity: A symmetrical design distributes seismic forces more evenly, while irregularities can lead to uneven stress distribution and increased risk of damage. 5. Strong Foundation: A strong and flexible foundation helps in distributing seismic forces effectively, preventing settlement or tilting. 6. Cross Bracing: Diagonal braces are added to improve the stability of the structure under lateral seismic forces. Earthquake-Resistant Structures Shear Walls Moment Resisting Frames Braced Frames THANK YOU….