MCB 104 Historical Background of Microscopy PDF
Document Details
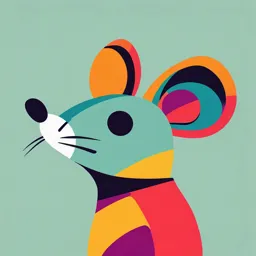
Uploaded by AwedBixbite9904
Kaduna State University
Tags
Summary
This document provides a historical overview of microscopy, starting with the simple magnifying glasses and progressing to the development of compound microscopes and the contributions of key figures like Leeuwenhoek and Hooke. It explores the concepts like chromatic aberration and spherical aberration and their resolution to image quality.
Full Transcript
MCB 104 HISTORICAL BACKGROUND OF MICROSCOPY The First Microscopes The early simple “microscopes” which were only magnifying glasses had one power, usually about 6X – 10X. One thing that was very common and interesting to look at, were fleas and other...
MCB 104 HISTORICAL BACKGROUND OF MICROSCOPY The First Microscopes The early simple “microscopes” which were only magnifying glasses had one power, usually about 6X – 10X. One thing that was very common and interesting to look at, were fleas and other tiny insects, hence these early magnifiers called “flea glasses”. In the year 1595, two Dutch spectacle makers, Zaccharias Janssen and his father Hans started experimenting with lenses. They put several lenses in a tube and made a very important discovery. The object near the end of the tube appeared to be greatly enlarged, much larger than any simple magnifying glass could achieve by itself! Their first microscopes were more of a novelty than a scientific tool since maximum magnification was only around 9X and the images were somewhat blurry. It was Antony Van Leeuwenhoek (1632-1723), a Dutch draper and scientist, and one of the pioneers of microscopy who in the late 17th century became the first man to make and use a real microscope. He is often referred to as the father of microscopy. In England, Robert Hooke re-confirmed Leeuwenhoek's discoveries of tiny living organisms in a drop of water. He replicated Leeuwenhoek's light microscope and proceeded to improve upon its design. Leeuwenhoek’s microscope used a single convex glass lens attached to a metal holder and was focused using screws. Compound Microscopes To increase the magnification power of a single-lens microscope, the focal length has to be reduced. However, a reduction in focal length necessitates a reduction of the lens diameter, and after a point, the lens becomes difficult to see through. To solve this problem, the compound microscope system was invented in the 17th century. This type of microscope incorporates more than one lens so that the image magnified by one lens can be further magnified by another. 1 Today, the term “microscope” is generally used to refer to this type of compound microscope. In the compound microscope, the lens closer to the object to be viewed is referred to as the “objective”, while the lens closer to the eye is called the “eyepiece”. Englishman Robert Hooke is credited with the microscopic milestone of discovering the basic unit of all life, the cell. In the mid 17th century, Hooke saw a structural mesh while studying a sample of cork that reminded him of the small monastic rooms called cells. Later Developments All the early microscopists saw quite distorted images due to the low quality of the glass and imperfect shape of their lenses. Little was done to improve the microscope until the middle of the 19th century when great strides were made and quality instruments like today’s microscope emerged. Companies in Germany like Zeiss and an American company founded by Charles Spencer began producing fine optical instruments. We can also mention Ernst Abbe, who carried out a theoretical study of optical principles, and Otto Schott, who conducted research on optical glass. In order for light microscopes to achieve better resolution, three basic problems had to be overcome: Chromatic aberration: the unequal bending of different colours of light that occur in a lens. This problem was first solved by Chester Hall in the 1730’s. He discovered that if he used a second lens of different shape and light bending properties he could realign the colours without losing all of the magnification of the first lens. Spherical aberration: the unequal bending of light that hits different parts of a lens. Joseph Jackson Lister solved this problem in 1830. He discovered that by putting lenses at precise distances from each other, the aberration from all but the first lens could be eliminated. Low power low curvature lenses could be made with minimal aberration and by using a lens of this type for the first in a series, the problem could be virtually eliminated. The third problem is that for a microscope, to be as good as physically possible, it must collect a cone of light that is as wide as possible. Ernst Abbe worked out the solution to this problem in the 1870’s. He determined the physical laws that govern the collection of light by an objective and maximized this collection by using water and oil immersion lenses. The maximum resolution that Abbe was able to achieve is about 10 times better than the resolution Leeuwenhoek had achieved about 100 years earlier. This resolution of 0.2 microns or 200 nanometers is a physical limit imposed by the wavelength of light. Carl Zeiss: Within the 1800s, a mechanic named Carl Zeiss began his own business in the German university town of Jena, Thuringia, with the goal of providing researchers with high-quality instruments. Between 1846 and 1866, microscopes of uniformly high quality were built in Zeiss' workshop in accordance with very strict rules of craftsmanship. In the beginning, these were very simple instruments that were used as dissection microscopes, but in 1857 the Zeiss workshop produced the first genuine compound microscope (equipped with an eyepiece and an objective). 2 The new instrument was called the Stativ 1, which combined practical functionality with the skilled optical refinement provided by a craftsman. After almost 20 years, Zeiss was employing about 20 qualified staff members and took great pride in what had become a prosperous business. He knew that his instruments were good, but he refused to accept the trial and error method used at the time for the production of optics. Zeiss also was aware that competition from other microscope manufacturers would eventually bypass his accomplishments if he failed to continue to produce innovations. With the ultimate goal of creating reproducible products, Zeiss acknowledged that his manufacturing procedure had to be based on precise rules and strict guidelines, or as he once said: "The working hand should have no other function than to precisely implement the shapes and dimensions of all the design components determined beforehand by computation." Dr. Ernst Abbe: For assistance in this endeavor, Zeiss formed a partnership with Dr. Ernst Abbe, a brilliant physicist and mathematician. Abbe was appointed as the research director of Zeiss Optical Works in late 1866. For the next six years, the team worked intensively to lay the scientific foundations for the design and fabrication of advanced optical systems. In 1869, they introduced a new illumination apparatus that was designed to improve the performance of microscope illumination. Three years later, in 1872, Abbe formulated his wave theory of microscopic imaging and defined what would become known as the Abbe Sine Condition. Several years later, Zeiss was producing a line of 17 different objectives, including three immersion systems, all featuring a level of image quality unknown until then. The construction of microscopes on a sound theoretical basis was possible at last, and still is today. The original formula for the calculation of the possible resolution of the microscope is still utilized more than a century later. Resolutionx,y = λ / 2[η sin(α)] or d = 0.5 λ/n.sinθ The Zeiss enterprise continued to push onward in the late 1800s. Abbe became an equal partner, and forward-thinking intelligence became the inherent capital of the young company. Several problems still remained for Zeiss Optical Works however, since the quality of optical glass produced during the period was not sufficient to provide the theoretical resolution that was dictated by Abbe's sine condition. The glass used in the construction of microscope lenses was not homogeneous and it tended to undergo a phase separation during cooling, which led to a varying refractive index throughout the glass, and therefore, light waves passing through these lenses were refracted unpredictably. In short, first-rate resolution was unattainable with the poor quality glass. Otto Schott: Abbe first met Otto Schott, a glass chemist, in 1881. Over the next several years, Abbe and Schott developed several new glass formulas and made adjustments to the mixing and annealing process to eliminate internal defects and produce optical-grade glass with a uniform refractive index. In 1884, Schott, Abbe, and Zeiss formed a new company known as "Jenaer Glaswerk Schott und Genossen". Continued experimentation with glass recipes and preparation techniques yielded highly successful results, and in 1886, they introduced a new type of objective, the apochromat. By this time, an incredible 44 different types of optical glass were being produced. The creation of the apochromat objectives (with and without immersion media) eliminated color aberrations, which greatly assists bacteriologists in identifying infectious bacteria, and brought the resolving power of the microscope to the limit we know today. The progress made in the 3 development of objectives led to fields of view larger than anything ever achieved before. In the course of time, it also became evident that more attention would have to be paid to illumination. PROPERTIES OF LIGHT AND GLASS IN RELATION TO MICROSCOPY Visible light consists of electromagnetic waves that behave like other waves. Hence, many of the properties of light that are relevant to microscopy can be understood in terms of light’s behavior as a wave. An important property of light waves is the wavelength, or the distance between one peak of a wave and the next peak. The height of each peak (or depth of each trough) is called the amplitude. In contrast, the frequency of the wave is the rate of vibration of the wave, or the number of wavelengths within a specified time period (Figure 1). Figure 1. (a) The amplitude is the height of a wave, whereas the wavelength is the distance between one peak and the next. (b) These waves have different frequencies, or rates of vibration. The wave at the top has the lowest frequency, since it has the fewest peaks per unit time. The wave at the bottom has the highest frequency. Interactions of Light Light waves interact with materials by being reflected, absorbed, or transmitted. Reflection occurs when a wave bounces off of a material. For example, a red piece of cloth may reflect red light to our eyes while absorbing other colors of light. Absorbance occurs when a material captures the energy of a light wave. In the case of glow-in-the-dark plastics, the energy from light can be absorbed and then later re-emitted as another form of phosphorescence. Transmission occurs when a wave travels through a material, like light through glass (the process of transmission is called transmittance). When a material allows a large proportion of light to be transmitted, it may do so because it is thinner, or more transparent (having more transparency and less opacity). Light waves can also interact with each other by interference, creating complex patterns of motion. Dropping two pebbles into a puddle causes the waves on the puddle’s surface to interact, creating complex interference patterns. Light waves can interact in the same way. 4 In addition to interfering with each other, light waves can also interact with small objects or openings by bending or scattering. This is called diffraction. Diffraction is larger when the object is smaller relative to the wavelength of the light. Often, when waves diffract in different directions around an obstacle or opening, they will interfere with each other. Refraction In the context of microscopy, refraction is perhaps the most important behavior exhibited by light waves. Refraction occurs when light waves change direction as they enter a new medium. Different transparent materials transmit light at different speeds; thus, light can change speed when passing from one material to another. This change in speed usually also causes a change in direction (refraction), with the degree of change dependent on the angle of the incoming light. Figure 2. (a) Refraction occurs when light passes from one medium, such as air, to another, such as glass, changing the direction of the light rays. (b) As shown in this diagram, light rays passing from one medium to another may be either refracted or reflected. Figure 3. This straight pole appears to bend at an angle as it enters the water. This optical illusion is due to the large difference between the refractive indices of air and water. 5 The extent to which a material slows transmission speed relative to empty space is called the refractive index of that material. Large differences between the refractive indices of two materials will result in a large amount of refraction when light passes from one material to the other. For example, light moves much more slowly through water than through air, so light entering water from air can change direction greatly. We say that the water has a higher refractive index than air. White light can be separated into its component colors using refraction. If we pass white light through a prism, different colors will be refracted in different directions, creating a rainbow-like spectrum on a screen behind the prism. This separation of colors is called dispersion, and it occurs because, for a given material, the refractive index is different for different frequencies of light. Certain materials can refract nonvisible forms of electromagnetic radiation (EMR) and, in effect, transform them into visible light. Certain fluorescent dyes, for instance, absorb ultraviolet or blue light and then use the energy to emit photons of a different color, giving off light rather than simply vibrating. This occurs because the energy absorption causes electrons to jump to higher energy states, after which they then almost immediately fall back down to their ground states, emitting specific amounts of energy as photons. Not all of the energy is emitted in a given photon, so the emitted photons will be of lower energy and, thus, of lower frequency than the absorbed ones. Thus, a dye such as Texas red may be excited by blue light, but emit red light; or a dye such as fluorescein isothiocyanate (FITC) may absorb (invisible) high-energy ultraviolet light and emit green light. In some materials, the photons may be emitted following a delay after absorption; in this case, the process is called phosphorescence. Glow-in-the-dark plastic works by using phosphorescent material. Magnification and Resolution Microscopes magnify images and use the properties of light to create useful images of small objects. Magnification is defined as the ability of a lens to enlarge the image of an object when compared to the real object. For example, a magnification of 10⨯ means that the image appears 10 times the size of the object as viewed with the naked eye. Greater magnification typically improves our ability to see details of small objects, but magnification alone is not sufficient to make the most useful images. It is often useful to enhance the resolution of objects: the ability to tell that two separate points or objects are separate. A low- resolution image appears fuzzy, whereas a high-resolution image appears sharp. Two factors affect resolution. The first is wavelength. Shorter wavelengths are able to resolve smaller objects; thus, an electron microscope has a much higher resolution than a light microscope, since it uses an electron beam with a very short wavelength, as opposed to the long-wavelength visible light used by a light microscope. The second factor that affects resolution is numerical aperture, which is a measure of a lens’s ability to gather light. The higher the numerical aperture, the better the resolution. FACTORS THAT AFFECT IMAGE CHARACTERISTIC Not to be confused with magnification, microscope resolution is the shortest distance between two separate points in a microscope’s field of view that can still be distinguished as distinct entities. 6 If the two points are closer together than your resolution then they will appear ill-defined and their positions will be inexact. A microscope may offer high magnification, but if the lenses are of poor quality the resulting poor resolution will degrade the image quality. Numerical Aperture Microscope resolution is affected by several elements. An optical microscope set on a high magnification may produce an image that is blurred and yet it is still at the maximum resolution of the objective lens. The numerical aperture of the objective lens affects the resolution. It indicates the ability of the lens to gather light and resolve a point at a fixed distance from the lens. Aberration and Diffraction Aberration is another factor related to lens performance that impacts resolution. Simply stated, microscope’s lenses are designed to focus light rays on a single point. More light rays straying from this focal point will occasion a greater amount of aberration and a greater amount of diffraction. However, if all light rays are focused on one infinite pinpoint this will also cause diffraction. Diffraction in microscopy is nothing more than interference or noise caused by the light rays passing through and around the specimen being viewed, passing through the small aperture of a lens, or bending at the edges of the objective. Without diffraction the specimen would not be visible. However, too much diffraction limits the resolution of a microscope. Lens manufacturers work to design lenses with the highest aberration correction possible for a particular class of objective lens. Mathematical computations have proven that the smallest point of focus for light rays without causing diffraction is 200 nanometers. This is the ideal resolution for an optical microscope. Light Wavelength and Refractive Index Microscope resolution is also impacted by the wavelength of light being used to illuminate the specimen. Longer wavelengths of light offer less resolution than short wavelength illumination. The range in nanometers of the wavelength of the visible light is from 380nm to 750nm. Another method of improving microscope resolution is to increase the refractive index between the objective lens and the specimen. The refractive index is merely a ratio expression of the relative speed of light passing through a substance as a proportion of the speed of light in a vacuum. As the refractive index increases the speed of the light passing through a medium is slower. As light slows down the wavelength gets shorter and yields better resolution. Objective lenses are manufactured that allow imaging in immersion oil which has a refractive index of 1.51 and substantially shortens light waves. 7 Microscope resolution is the most important determinant of how well a microscope will perform and is determined by the numerical aperture and light wavelength. It is not impacted by magnification but does determine the useful magnification of a microscope. Even though 200 nanometers is considered the optimal resolution for optical microscopes, higher resolutions can be obtained using fluorescence microscopy. When combined with a laser light source, focal plane resolution of 15-20 nanometers can be achieved. However, in routine microscopic observations the highest resolution possible is usually unnecessary. STAINING TECHNIQUES Stain A stain is a substance that adheres to a cell, giving the cell colour. The presence of colour gives the cells significant contrast so they are much more visible. Different stains have affinities for different organisms, or different parts of organisms. They are used to differentiate different types of organisms or to view specific parts of organisms Staining Staining is an auxiliary technique used in microscopy to enhance contrast in the microscopic image. Stains and dyes are frequently used in biology and medicine to highlight structures in biological tissues for viewing, often with the aid of different microscopes. Fixation Fixation is used to preserve the shape of the cells or tissue involved as much as possible. Sometimes heat fixation is used to kill, adhere, coagulate the protoplasm and makes cells or tissues permeable so they will accept stains. Hanging drop technique This technique is meant for microscopic observation of living bacteria. Hanging drop technique enables viewing of size, shape, arrangement and motility of live microorganisms in fluid media. It requires the use of special ground slides. In this technique a loopful of bacterial suspension is placed in the center of a cover slip. In the four corners tiny droplets of mineral oil are placed. The hollow ground slide is placed over the cover slip with the depression side down and the slide is inverted quickly so that the water cannot run off to one side. However, the lack of contrast yields limited though valuable information. Microbial Staining Microbiologists commonly stain bacterial cells before viewing them because the cytoplasm lacks colour, making it hard to see the cells on the bright background of the microscope field. Several staining techniques have been developed to provide contrast for bright-field microscopy. The main advantages of staining are that it 8