The Science and Engineering of Materials PDF
Document Details
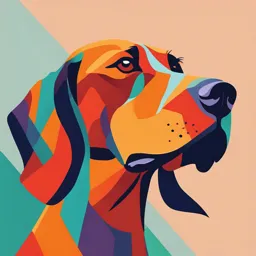
Uploaded by StainlessAlien
Tags
Related
- Chemistry of Engineering Materials Engineering Chemistry PDF
- Materials Science and Engineering: An Introduction (10th Edition) PDF
- Materials Science and Engineering: An Introduction PDF
- Introduction to Materials Science & Engineering PDF
- Properties of Engineering Materials Lecture Notes PDF
- The Importance of Engineering Materials in Present World PDF
Summary
This textbook provides an introduction to materials science and engineering. It covers the composition, microstructure, and processing of various materials, their properties, and applications. It also illustrates how material properties can be tailored to meet specific design requirements.
Full Transcript
The Science and Engineering of Materials Sixth Edition Copyright 2010 Cengage Learning, Inc. All Rights Reserved. May not be copied, scanned, or duplicated, in whole or in part. The principal...
The Science and Engineering of Materials Sixth Edition Copyright 2010 Cengage Learning, Inc. All Rights Reserved. May not be copied, scanned, or duplicated, in whole or in part. The principal goals of a materials scientist and engineer are to (1) make existing materials better and (2) invent or discover new phenomena, materials, devices, and applications. Breakthroughs in the materi- als science and engineering field are applied to many other fields of study such as biomedical engineering, physics, chemistry, environmental engineering, and information technology. The materials science and engi- neering tetrahedron shown here represents the heart and soul of this field. As shown in this diagram, a materials scientist and engineer’s main objective is to develop materials or devices that have the best per- formance for a particular application. In most cases, the performance-to-cost ratio, as opposed to the per- formance alone, is of utmost importance. This concept is shown as the apex of the tetrahedron and the three corners are representative of A—the composition, B—the microstructure, and C—the synthesis and pro- cessing of materials. These are all interconnected and ultimately affect the performance-to-cost ratio of a material or a device. The accompanying micrograph shows the microstructure of stainless steel. For materials scientists and engineers, materials are like a palette of colors to an artist. Just as an artist can create different paintings using different colors, materials scientists create and improve upon different materials using different elements of the periodic table, and different synthesis and processing routes. (Car image courtesy of Ford Motor Company. Steel manufacturing image and car chassis image courtesy of Digital Vision/Getty Images. Micrograph courtesy of Dr. A.J. Deardo, Dr. M. Hua, and Dr. J. Garcia.) Copyright 2010 Cengage Learning, Inc. All Rights Reserved. May not be copied, scanned, or duplicated, in whole or in part. Introduction to Materials Chapter 1 Science and Engineering Have You Ever Wondered? What do materials scientists and engineers study? How can steel sheet metal be processed to produce a high strength, lightweight, energy absorbing, malleable material used in the manufacture of car chassis? Can we make flexible and lightweight electronic circuits using plastics? What is a “smart material?” I n this chapter, we will first introduce you to the field of materials science and engi- neering (MSE) using different real-world examples. We will then provide an introduc- tion to the classification of materials. Although most engineering programs require students to take a materials science course, you should approach your study of materi- als science as more than a mere requirement. A thorough knowledge of materials sci- ence and engineering will make you a better engineer and designer. Materials science underlies all technological advances and an understanding of the basics of materials and their applications will not only make you a better engineer, but will help you during the design process. In order to be a good designer, you must learn what materials will be appropriate to use in different applications. You need to be capable of choosing the right material for your application based on its properties, and you must recognize how and why these properties might change over time and due to processing. Any engineer can look up materials properties in a book or search databases for a material that meets design specifications, but the ability to innovate and to incorporate materials safely in a design is rooted in an understanding of how to manipulate materials properties and functionality through the control of the material’s structure and processing techniques. The most important aspect of materials is that they are enabling; materials make things happen. For example, in the history of civilization, materials such as stone, iron, and bronze played a key role in mankind’s development. In today’s fast-paced world, the discovery of silicon single crystals and an understanding of their properties have enabled the information age. 3 Copyright 2010 Cengage Learning, Inc. All Rights Reserved. May not be copied, scanned, or duplicated, in whole or in part. 4 CHAPTER 1 Introduction to Materials Science and Engineering In this book, we provide compelling examples of real-world applications of engineered materials. The diversity of applications and the unique uses of materials illustrate why a good engineer needs to understand and know how to apply the prin- ciples of materials science and engineering. 1-1 What is Materials Science and Engineering? Materials science and engineering (MSE) is an interdisciplinary field of science and engi- neering that studies and manipulates the composition and structure of materials across length scales to control materials properties through synthesis and processing. The term composition means the chemical make-up of a material. The term structure means a description of the arrangement of atoms, as seen at different levels of detail. Materials sci- entists and engineers not only deal with the development of materials, but also with the synthesis and processing of materials and manufacturing processes related to the produc- tion of components. The term “synthesis” refers to how materials are made from naturally occurring or man-made chemicals. The term “processing” means how materials are shaped into useful components to cause changes in the properties of different materials. One of the most important functions of materials scientists and engineers is to establish the rela- tionships between a material or a device’s properties and performance and the microstruc- ture of that material, its composition, and the way the material or the device was synthesized and processed. In materials science, the emphasis is on the underlying relation- ships between the synthesis and processing, structure, and properties of materials. In materials engineering, the focus is on how to translate or transform materials into useful devices or structures. One of the most fascinating aspects of materials science involves the investiga- tion of a material’s structure. The structure of materials has a profound influence on many properties of materials, even if the overall composition does not change! For exam- ple, if you take a pure copper wire and bend it repeatedly, the wire not only becomes harder but also becomes increasingly brittle! Eventually, the pure copper wire becomes so hard and brittle that it will break! The electrical resistivity of the wire will also increase as we bend it repeatedly. In this simple example, take note that we did not change the material’s composition (i.e., its chemical make-up). The changes in the material’s prop- erties are due to a change in its internal structure. If you look at the wire after bending, it will look the same as before; however, its structure has been changed at the microscopic scale. The structure at the microscopic scale is known as the microstructure. If we can understand what has changed microscopically, we can begin to discover ways to control the material’s properties. Let’s examine one example using the materials science and engineering tetrahe- dron presented on the chapter opening page. Let’s look at “sheet steels” used in the manufacture of car chassis (Figure 1-1). Steels, as you may know, have been used in man- ufacturing for more than a hundred years, but they probably existed in a crude form dur- ing the Iron Age, thousands of years ago. In the manufacture of automobile chassis, a material is needed that possesses extremely high strength but is formed easily into aero- dynamic contours. Another consideration is fuel efficiency, so the sheet steel must also be thin and lightweight. The sheet steels also should be able to absorb significant amounts of energy in the event of a crash, thereby increasing vehicle safety. These are somewhat contradictory requirements. Copyright 2010 Cengage Learning, Inc. All Rights Reserved. May not be copied, scanned, or duplicated, in whole or in part. 1 - 1 What is Materials Science and Engineering? 5 Figure 1-1 Application of the tetrahedron of materials science and engineering to sheet steels for automotive chassis. Note that the composition, microstructure, and synthesis-processing are all interconnected and affect the performance-to-cost ratio. (Car image courtesy of Ford Motor Company. Steel manufacturing image and car chassis image courtesy of Digital Vision/Getty Images. Micrograph courtesy of Dr. A.J. Deardo, Dr. M. Hua, and Dr. J. Garcia.) Thus, in this case, materials scientists are concerned with the sheet steel’s composition; strength; weight; energy absorption properties; and malleability (formability). Materials scientists would examine steel at a microscopic level to determine if its properties can be altered to meet all of these requirements. They also would have to Copyright 2010 Cengage Learning, Inc. All Rights Reserved. May not be copied, scanned, or duplicated, in whole or in part. 6 CHAPTER 1 Introduction to Materials Science and Engineering consider the cost of processing this steel along with other considerations. How can we shape such steel into a car chassis in a cost-effective way? Will the shaping process itself affect the mechanical properties of the steel? What kind of coatings can be developed to make the steel corrosion resistant? In some applications, we need to know if these steels could be welded easily. From this discussion, you can see that many issues need to be con- sidered during the design and materials selection for any product. Let’s look at one more example of a class of materials known as semiconducting polymers (Figure 1-2). Many semiconducting polymers have been processed into light emit- ting diodes (LEDs). You have seen LEDs in alarm clocks, watches, and other displays. These displays often use inorganic compounds based on gallium arsenide (GaAs) and other materials. The advantage of using plastics for microelectronics is that they are lightweight and flexible. The questions materials scientists and engineers must answer with applica- tions of semiconducting polymers are What are the relationships between the structure of polymers and their electrical properties? How can devices be made using these plastics? Will these devices be compatible with existing silicon chip technology? Figure 1-2 Application of the tetrahedron of materials science and engineering to semiconducting polymers for microelectronics. Copyright 2010 Cengage Learning, Inc. All Rights Reserved. May not be copied, scanned, or duplicated, in whole or in part. 1 - 2 Classification of Materials 7 How robust are these devices? How will the performance and cost of these devices compare with traditional devices? These are just a few of the factors that engineers and scientists must consider during the development, design, and manufacture of semiconducting polymer devices. 1-2 Classification of Materials There are different ways of classifying materials. One way is to describe five groups (Table 1-1): TABLE 1-1 Representative examples, applications, and properties for each category of materials Examples of Applications Properties Metals and Alloys Copper Electrical conductor wire High electrical conductivity, good formability Gray cast iron Automobile engine blocks Castable, machinable, vibration-damping Alloy steels Wrenches, automobile chassis Significantly strengthened by heat treatment Ceramics and Glasses SiO2–Na2O–CaO Window glass Optically transparent, thermally insulating Al2O3, MgO, SiO2 Refractories (i.e., heat-resistant lining Thermally insulating, withstand of furnaces) for containing molten high temperatures, relatively metal inert to molten metal Barium titanate Capacitors for microelectronics High ability to store charge Silica Optical fibers for information Refractive index, low optical technology losses Polymers Polyethylene Food packaging Easily formed into thin, flexible, airtight film Epoxy Encapsulation of integrated circuits Electrically insulating and moisture-resistant Phenolics Adhesives for joining plies in plywood Strong, moisture resistant Semiconductors Silicon Transistors and integrated circuits Unique electrical behavior GaAs Optoelectronic systems Converts electrical signals to light, lasers, laser diodes, etc. Composites Graphite-epoxy Aircraft components High strength-to-weight ratio Tungsten carbide-cobalt Carbide cutting tools for machining High hardness, yet good (WC-Co) shock resistance Titanium-clad steel Reactor vessels Low cost and high strength of steel with the corrosion resistance of titanium Copyright 2010 Cengage Learning, Inc. All Rights Reserved. May not be copied, scanned, or duplicated, in whole or in part. 1 - 3 Functional Classification of Materials 11 1-3 Functional Classification of Materials We can classify materials based on whether the most important function they perform is mechanical (structural), biological, electrical, magnetic, or optical. This classification of materials is shown in Figure 1-6. Some examples of each category are shown. These cat- egories can be broken down further into subcategories. Figure 1-6 Functional classification of materials. Notice that metals, plastics, and ceramics occur in different categories. A limited number of examples in each category are provided. Copyright 2010 Cengage Learning, Inc. All Rights Reserved. May not be copied, scanned, or duplicated, in whole or in part. 12 CHAPTER 1 Introduction to Materials Science and Engineering Aerospace Light materials such as wood and an aluminum alloy (that acci- dentally strengthened the engine even more by picking up copper from the mold used for casting) were used in the Wright brothers’ historic flight. Today, NASA’s space shuttle makes use of aluminum powder for booster rockets. Aluminum alloys, plastics, silica for space shuttle tiles, and many other materials belong to this category. Biomedical Our bones and teeth are made, in part, from a naturally formed ceramic known as hydroxyapatite. A number of artificial organs, bone replacement parts, cardiovascular stents, orthodontic braces, and other components are made using differ- ent plastics, titanium alloys, and nonmagnetic stainless steels. Ultrasonic imaging systems make use of ceramics known as PZT (lead zirconium titanate). Magnets used for magnetic resonance imaging make use of metallic niobium tin-based superconductors. Electronic Materials As mentioned before, semiconductors, such as those made from silicon, are used to make integrated circuits for computer chips. Barium titanate (BaTiO3), tantalum oxide (Ta2O5), and many other dielectric materials are used to make ceramic capacitors and other devices. Superconductors are used in making pow- erful magnets. Copper, aluminum, and other metals are used as conductors in power trans- mission and in microelectronics. Energy Technology and Environmental Technology The nuclear industry uses materials such as uranium dioxide and plutonium as fuel. Numerous other materials, such as glasses and stainless steels, are used in handling nuclear materials and managing radioactive waste. New technologies related to batteries and fuel cells make use of many ceramic materials such as zirconia (ZrO2) and polymers. Battery technology has gained significant importance owing to the need for many electronic devices that require longer lasting and portable power. Fuel cells will also be used in elec- tric cars. The oil and petroleum industry widely uses zeolites, alumina, and other materi- als as catalyst substrates. They use Pt, Pt/Rh and many other metals as catalysts. Many membrane technologies for purification of liquids and gases make use of ceramics and plastics. Solar power is generated using materials such as amorphous silicon (a:Si:H). Magnetic Materials Computer hard disks make use of many ceramic, metallic, and polymeric materials. Computer hard disks are made using alloys based on cobalt-platinum-tantalum-chromium (Co-Pt-Ta-Cr) alloys. Many magnetic ferrites are used to make inductors and components for wireless communications. Steels based on iron and silicon are used to make transformer cores. Photonic or Optical Materials Silica is used widely for making opti- cal fibers. More than ten million kilometers of optical fiber have been installed around the world. Optical materials are used for making semiconductor detectors and lasers used in fiber optic communications systems and other applications. Similarly, alumina (Al2O3) and yttrium aluminum garnets (YAG) are used for making lasers. Amorphous silicon is used to make solar cells and photovoltaic modules. Polymers are used to make liquid crystal displays (LCDs). Smart Materials A smart material can sense and respond to an external stimulus such as a change in temperature, the application of a stress, or a change in humid- ity or chemical environment. Usually a smart material-based system consists of sensors Copyright 2010 Cengage Learning, Inc. All Rights Reserved. May not be copied, scanned, or duplicated, in whole or in part. 1 - 5 Environmental and Other Effects 13 and actuators that read changes and initiate an action. An example of a passively smart material is lead zirconium titanate (PZT) and shape-memory alloys. When properly processed, PZT can be subjected to a stress, and a voltage is generated. This effect is used to make such devices as spark generators for gas grills and sensors that can detect under- water objects such as fish and submarines. Other examples of smart materials include magnetorheological or MR fluids. These are magnetic paints that respond to magnetic fields. These materials are being used in suspension systems of automobiles, including models by General Motors, Ferrari, and Audi. Still other examples of smart materials and systems are photochromic glasses and automatic dimming mirrors. Structural Materials These materials are designed for carrying some type of stress. Steels, concrete, and composites are used to make buildings and bridges. Steels, glasses, plastics, and composites also are used widely to make automotives. Often in these applications, combinations of strength, stiffness, and toughness are needed under different conditions of temperature and loading. 1-4 Classification of Materials Based on Structure As mentioned before, the term “structure” means the arrangement of a material’s atoms; the structure at a microscopic scale is known as “microstructure.” We can view these arrangements at different scales, ranging from a few angstrom units to a millimeter. We will learn in Chapter 3 that some materials may be crystalline (the material’s atoms are arranged in a periodic fashion) or they may be amorphous (the arrangement of the material’s atoms does not have long-range order). Some crystalline materials may be in the form of one crystal and are known as single crystals. Others consist of many crys- tals or grains and are known as polycrystalline. The characteristics of crystals or grains (size, shape, etc.) and that of the regions between them, known as the grain boundaries, also affect the properties of materials. We will further discuss these concepts in later chapters. A micrograph of stainless steel showing grains and grain boundaries is shown in Figure 1-1. 1-5 Environmental and Other Effects The structure-property relationships in materials fabricated into components are often influenced by the surroundings to which the material is subjected during use. This can include exposure to high or low temperatures, cyclical stresses, sudden impact, corrosion, or oxidation. These effects must be accounted for in design to ensure that components do not fail unexpectedly. Temperature Changes in temperature dramatically alter the properties of materials (Figure 1-7). Metals and alloys that have been strengthened by certain heat treatments or forming techniques may suddenly lose their strength when heated. A tragic reminder of this is the collapse of the World Trade Center towers on Copyright 2010 Cengage Learning, Inc. All Rights Reserved. May not be copied, scanned, or duplicated, in whole or in part. 14 CHAPTER 1 Introduction to Materials Science and Engineering Figure 1-7 Increasing temperature normally reduces the strength of a material. Polymers are suitable only at low temperatures. Some composites, such as carbon-carbon composites, special alloys, and ceramics, have excellent properties at high temperatures. September 11, 2001. Although the towers sustained the initial impact of the collisions, their steel structures were weakened by elevated temperatures caused by fire, ultimately leading to the collapse. High temperatures change the structure of ceramics and cause polymers to melt or char. Very low temperatures, at the other extreme, may cause a metal or polymer to fail in a brittle manner, even though the applied loads are low. This low-temperature embrit- tlement was a factor that caused the Titanic to fracture and sink. Similarly, the 1986 Challenger accident, in part, was due to embrittlement of rubber O-rings. The reasons why some polymers and metallic materials become brittle are different. We will discuss these concepts in later chapters. The design of materials with improved resistance to temperature extremes is essential in many technologies, as illustrated by the increase in operating temperatures of aircraft and aerospace vehicles (Figure 1-8). As higher speeds are attained, more heating of the vehicle skin occurs because of friction with the air. Also, engines operate more effi- ciently at higher temperatures. In order to achieve higher speed and better fuel economy, Figure 1-8 Skin operating temperatures for aircraft have increased with the development of improved materials. (After M. Steinberg, Scientific American, October 1986.) National aerospace plane Copyright 2010 Cengage Learning, Inc. All Rights Reserved. May not be copied, scanned, or duplicated, in whole or in part. 1 - 5 Environmental and Other Effects 15 Figure 1-9 NASA’s X-43A unmanned aircraft is an example of an advanced hypersonic vehicle. (Courtesy of NASA Dryden Flight Research Center (NASA-DFRC).) new materials have gradually increased allowable skin and engine temperatures. NASA’s X-43A unmanned aircraft is an example of an advanced hypersonic vehicle (Figure 1-9). It sustained a speed of approximately Mach 10 (7500 miles/h or 12,000 km/h) in 2004. Materials used include refractory tiles in a thermal protection system designed by Boeing and carbon-carbon composites. Corrosion Most metals and polymers react with oxygen or other gases, partic- ularly at elevated temperatures. Metals and ceramics may disintegrate and polymers and non-oxide ceramics may oxidize. Materials also are attacked by corrosive liquids, leading to premature failure. The engineer faces the challenge of selecting materials or coatings that prevent these reactions and permit operation in extreme environments. In space appli- cations, we may have to consider the effect of radiation. Fatigue In many applications, components must be designed such that the load on the material may not be enough to cause permanent deformation. When we load and unload the material thousands of times, even at low loads, small cracks may begin to develop, and materials fail as these cracks grow. This is known as fatigue failure. In design- ing load-bearing components, the possibility of fatigue must be accounted for. Strain Rate Many of you are aware of the fact that Silly Putty®, a silicone-(not silicon-) based plastic, can be stretched significantly if we pull it slowly (small rate of strain). If you pull it fast (higher rate of strain), it snaps. A similar behavior can occur with many metallic materials. Thus, in many applications, the level and rate of strain have to be considered. Copyright 2010 Cengage Learning, Inc. All Rights Reserved. May not be copied, scanned, or duplicated, in whole or in part. 16 CHAPTER 1 Introduction to Materials Science and Engineering In many cases, the effects of temperature, fatigue, stress, and corrosion may be interrelated, and other outside effects could affect the material’s performance. 1-6 Materials Design and Selection When a material is designed for a given application, a number of factors must be consid- ered. The material must acquire the desired physical and mechanical properties, must be capable of being processed or manufactured into the desired shape, and must provide an economical solution to the design problem. Satisfying these requirements in a manner that protects the environment—perhaps by encouraging recycling of the materials—is also essential. In meeting these design requirements, the engineer may have to make a number of trade-offs in order to produce a serviceable, yet marketable, product. As an example, material cost is normally calculated on a cost-per-pound basis. We must consider the density of the material, or its weight-per-unit volume, in our design and selection (Table 1-2). Aluminum may cost more than steel on a weight basis, but it is only one-third the density of steel. Although parts made from aluminum may have to be thicker, the aluminum part may be less expensive than the one made from steel because of the weight difference. In some instances, particularly in aerospace applications, weight is critical, since additional vehicle weight increases fuel consumption. By using materials that are light- weight but very strong, aerospace or automobile vehicles can be designed to improve fuel utilization. Many advanced aerospace vehicles use composite materials instead of alu- minum. These composites, such as carbon-epoxy, are more expensive than the traditional aluminum alloys; however, the fuel savings yielded by the higher strength-to-weight ratio of the composite (Table 1-2) may offset the higher initial cost of the aircraft. There are lit- erally thousands of applications in which similar considerations apply. Usually the selec- tion of materials involves trade-offs between many properties. By this point of our discussion, we hope that you can appreciate that the prop- erties of materials depend not only on composition, but also how the materials are made (synthesis and processing) and, most importantly, their internal structure. This is why it is not a good idea for an engineer to refer to a handbook and select a material for a given application. The handbooks may be a good starting point. A good engineer will con- sider: the effects of how the material was made, the exact composition of the candidate TABLE 1-2 Strength-to-weight ratios of various materials Strength-to-weight Material Strength (lb/in.2) Density (lb/in.3) ratio (in.) Polyethylene 1,000 0.030 0.03 ! 106 Pure aluminum 6,500 0.098 0.07 ! 106 Al2O3 30,000 0.114 0.26 ! 106 Epoxy 15,000 0.050 0.30 ! 106 Heat-treated alloy steel 240,000 0.280 0.86 ! 106 Heat-treated aluminum alloy 86,000 0.098 0.88 ! 106 Carbon-carbon composite 60,000 0.065 0.92 ! 106 Heat-treated titanium alloy 170,000 0.160 1.06 ! 106 Kevlar-epoxy composite 65,000 0.050 1.30 ! 106 Carbon-epoxy composite 80,000 0.050 1.60 ! 106 Copyright 2010 Cengage Learning, Inc. All Rights Reserved. May not be copied, scanned, or duplicated, in whole or in part. Summary 17 material for the application being considered, any processing that may have to be done for shaping the material or fabricating a component, the structure of the material after processing into a component or device, the environment in which the material will be used, and the cost-to-performance ratio. Earlier in this chapter, we had discussed the need for you to know the principles of materials science and engineering. If you are an engineer and you need to decide which materials you will choose to fabricate a component, the knowledge of principles of mate- rials science and engineering will empower you with the fundamental concepts. These will allow you to make technically sound decisions in designing with engineered materials. Summary Materials science and engineering (MSE) is an interdisciplinary field concerned with inventing new materials and devices and improving previously known materials by developing a deeper understanding of the microstructure-composition-synthesis- processing relationships. Engineered materials are materials designed and fabricated considering MSE principles. The properties of engineered materials depend upon their composition, structure, syn- thesis, and processing. An important performance index for materials or devices is their performance-to-cost ratio. The structure of a material refers to the arrangement of atoms or ions in the material. The structure at a microscopic level is known as the microstructure. Many properties of materials depend strongly on the structure, even if the composition of the material remains the same. This is why the structure-property or microstructure- property relationships in materials are extremely important. Materials are classified as metals and alloys, ceramics, glasses and glass-ceramics, com- posites, polymers, and semiconductors. Metals and alloys have good strength, good ductility, and good formability. Metals have good electrical and thermal conductivity. Metals and alloys play an indispensable role in many applications such as automotives, buildings, bridges, aerospace, and the like. Ceramics are inorganic crystalline materials. They are strong, serve as good electrical and thermal insulators, are often resistant to damage by high temperatures and corro- sive environments, but are mechanically brittle. Modern ceramics form the underpin- nings of many microelectronic and photonic technologies. Glasses are amorphous, inorganic solids that are typically derived from a molten liquid. Glasses can be tempered to increase strength. Glass-ceramics are formed by annealing glasses to nucleate small crystals that improve resistance to fracture and thermal shock. Polymers have relatively low strength; however, the strength-to-weight ratio is very favorable. Polymers are not suitable for use at high temperatures. They have very good corrosion resistance, and—like ceramics—provide good electrical and thermal insula- tion. Polymers may be either ductile or brittle, depending on structure, temperature, and strain rate. Semiconductors possess unique electrical and optical properties that make them essen- tial for manufacturing components in electronic and communication devices. Copyright 2010 Cengage Learning, Inc. All Rights Reserved. May not be copied, scanned, or duplicated, in whole or in part. 18 CHAPTER 1 Introduction to Materials Science and Engineering Composites are made from different types of materials. They provide unique combina- tions of mechanical and physical properties that cannot be found in any single material. Functional classification of materials includes aerospace, biomedical, electronic, energy and environmental, magnetic, optical (photonic), and structural materials. Materials can also be classified as crystalline or amorphous. Crystalline materials may be single crystal or polycrystalline. Properties of materials can depend upon the temperature, level and type of stress applied, strain rate, oxidation and corrosion, and other environmental factors. Selection of a material having the needed properties and the potential to be manufac- tured economically and safely into a useful product is a complicated process requiring the knowledge of the structure-property-processing-composition relationships. Glossary Alloy A metallic material that is obtained by chemical combinations of different elements (e.g., steel is made from iron and carbon). Typically, alloys have better mechanical properties than pure metals. Ceramics A group of crystalline inorganic materials characterized by good strength, especially in compression, and high melting temperatures. Many ceramics have very good electrical and thermal insulation behavior. Composites A group of materials formed from mixtures of metals, ceramics, or polymers in such a manner that unusual combinations of properties are obtained (e.g., fiberglass). Composition The chemical make-up of a material. Crystalline material A material composed of one or many crystals. In each crystal, atoms or ions show a long-range periodic arrangement. Density Mass per unit volume of a material, usually expressed in units of g/cm3 or lb/in.3 Fatigue failure Failure of a material due to repeated loading and unloading. Glass An amorphous material derived from the molten state, typically, but not always, based on silica. Glass-ceramics A special class of materials obtained by forming a glass and then heat treating it to form small crystals. Grain boundaries Regions between grains of a polycrystalline material. Grains Crystals in a polycrystalline material. Materials engineering An engineering oriented field that focuses on how to transform materi- als into a useful device or structure. Materials science A field of science that emphasizes studies of relationships between the microstructure, synthesis and processing, and properties of materials. Materials science and engineering (MSE) An interdisciplinary field concerned with invent- ing new materials and improving previously known materials by developing a deeper understanding of the microstructure-composition-synthesis-processing relationships between different materials. Materials science and engineering tetrahedron A tetrahedron diagram showing how the performance-to-cost ratio of materials depends upon the composition, microstructure, synthesis, and processing. Mechanical properties Properties of a material, such as strength, that describe how well a mate- rial withstands applied forces, including tensile or compressive forces, impact forces, cyclical or fatigue forces, or forces at high temperatures. Metal An element that has metallic bonding and generally good ductility, strength, and electrical conductivity. Copyright 2010 Cengage Learning, Inc. All Rights Reserved. May not be copied, scanned, or duplicated, in whole or in part. Problems 19 Microstructure The structure of a material at the microscopic length scale. Physical properties Characteristics such as color, elasticity, electrical or thermal conductivity, magnetism, and optical behavior that generally are not significantly influenced by forces acting on a material. Plastics Polymers containing other additives. Polycrystalline material A material composed of many crystals (as opposed to a single-crystal material that has only one crystal). Polymerization The process by which organic molecules are joined into giant molecules, or polymers. Polymers A group of materials normally obtained by joining organic molecules into giant molec- ular chains or networks. Polymers are characterized by low strengths, low melting temperatures, and poor electrical conductivity. Processing Different ways for shaping materials into useful components or changing their properties. Semiconductors A group of materials having electrical conductivity between metals and typical ceramics (e.g., Si, GaAs). Single crystal A crystalline material that is made of only one crystal (there are no grain boundaries). Smart material A material that can sense and respond to an external stimulus such as change in temperature, application of a stress, or change in humidity or chemical environment. Strength-to-weight ratio The strength of a material divided by its density; materials with a high strength-to-weight ratio are strong but lightweight. Structure Description of the arrangements of atoms or ions in a material. The structure of mate- rials has a profound influence on many properties of materials, even if the overall composition does not change. Synthesis The process by which materials are made from naturally occurring or other chemicals. Thermoplastics A special group of polymers in which molecular chains are entangled but not interconnected. They can be easily melted and formed into useful shapes. Normally, these polymers have a chainlike structure (e.g., polyethylene). Thermosets A special group of polymers that decompose rather than melt upon heating. They are normally quite brittle due to a relatively rigid, three-dimensional network structure (e.g., polyurethane). Problems Section 1-1 What is Materials Science and Section 1-4 Classification of Materials Engineering? Based on Structure 1-1 Define materials science and engineering Section 1-5 Environmental and Other (MSE). Effects 1-2 Define the following terms: (a) composition, (b) structure, (c) synthesis, (d) processing, 1-4 For each of the following classes of materi- and (e) microstructure. als, give two specific examples that are a reg- ular part of your life: 1-3 Explain the difference between the terms (a) metals; materials science and materials engineering. (b) ceramics; Section 1-2 Classification of Materials (c) polymers; and (d) semiconductors. Section 1-3 Functional Classification Specify the object that each material is of Materials found in and explain why the material is Copyright 2010 Cengage Learning, Inc. All Rights Reserved. May not be copied, scanned, or duplicated, in whole or in part. 20 CHAPTER 1 Introduction to Materials Science and Engineering used in each specific application. Hint: 1-10 Coiled springs ought to be very strong and One example answer for part (a) would be stiff. Si3N4 is a strong, stiff material. Would that aluminum is a metal used in the base you select this material for a spring? of some pots and pans for even heat distri- Explain. bution. It is also a lightweight metal that 1-11 Temperature indicators are sometimes pro- makes it useful in kitchen cookware. Note duced from a coiled metal strip that uncoils that in this partial answer to part (a), a a specific amount when the temperature specific metal is described for a specific increases. How does this work; from what application. kind of material would the indicator be made; 1-5 Describe the enabling materials property of and what are the important properties that the each of the following and why it is so: material in the indicator must possess? (a) silica tiles for the space shuttle; 1-12 You would like to design an aircraft that can (b) steel for I-beams in skyscrapers; be flown by human power nonstop for a dis- (c) a cobalt chrome molybdenum alloy for tance of 30 km. What types of material hip implants; properties would you recommend? What (d) polycarbonate for eyeglass lenses; and materials might be appropriate? (e) bronze for sculptures. 1-13 You would like to place a three foot diame- 1-6 Describe the enabling materials property of ter microsatellite into orbit. The satellite will each of the following and why it is so: contain delicate electronic equipment that (a) aluminum for airplane bodies; will send and receive radio signals from (b) polyurethane for teeth aligners (invisible earth. Design the outer shell within which braces); the electronic equipment is contained. What (c) steel for the ball bearings in a bicycle’s properties will be required, and what kind of wheel hub; materials might be considered? (d) polyethylene terephthalate for water 1-14 What properties should the head of a car- bottles; and penter’s hammer possess? How would you (e) glass for wine bottles. manufacture a hammer head? 1-7 Write one paragraph about why single- 1-15 The hull of the space shuttle consists of crystal silicon is currently the material of ceramic tiles bonded to an aluminum skin. choice for microelecronics applications. Discuss the design requirements of the Write a second paragraph about potential shuttle hull that led to the use of this combi- alternatives to single-crystal silicon for solar nation of materials. What problems in pro- cell applications. Provide a list of the refer- ducing the hull might the designers and ences or websites that you used. You must manufacturers have faced? use at least three references. 1-16 You would like to select a material for the 1-8 Steel is often coated with a thin layer of zinc electrical contacts in an electrical switching if it is to be used outside. What characteris- device that opens and closes frequently and tics do you think the zinc provides to this forcefully. What properties should the con- coated, or galvanized, steel? What precau- tact material possess? What type of material tions should be considered in producing this might you recommend? Would Al2O3 be a product? How will the recyclability of the good choice? Explain. product be affected? 1-17 Aluminum has a density of 2.7 g/cm3. 1-9 We would like to produce a transparent Suppose you would like to produce a compos- canopy for an aircraft. If we were to use a ite material based on aluminum having a den- traditional window glass canopy, rocks or sity of 1.5 g/cm3. Design a material that would birds might cause it to shatter. Design a have this density. Would introducing beads of material that would minimize damage or at polyethylene, with a density of 0.95 g/cm3, into least keep the canopy from breaking into the aluminum be a likely possibility? Explain. pieces. Copyright 2010 Cengage Learning, Inc. All Rights Reserved. May not be copied, scanned, or duplicated, in whole or in part. Problems 21 1-18 You would like to be able to identify dif- These three problems are designed to provide an ferent materials without resorting to chem- introduction to Knovel, its website, and the inter- ical analysis or lengthy testing procedures. active tools available on it. For a detailed introduc- Describe some possible testing and sorting tion describing the use of Knovel, please visit your techniques you might be able to use based textbook’s website at: http://www.cengage.com/ on the physical properties of materials. engineering/askeland and go to the Student 1-19 You would like to be able to physically sep- Companion site. arate different materials in a scrap recycling K1-1 Convert 7750 kg/m3 to lb/ft3 using the Unit plant. Describe some possible methods that Converter. might be used to separate materials such as polymers, aluminum alloys, and steels from Using the Periodic Table, determine the one another. atomic weight of magnesium. 1-20 Some pistons for automobile engines might What is the name of Section 4 in be produced from a composite material con- Perry’s Chemical Engineers’ Handbook taining small, hard silicon carbide particles (Seventh Edition)? in an aluminum alloy matrix. Explain what Find a book title that encompasses the fun- benefits each material in the composite may damentals of chemistry as well as contains provide to the overall part. What problems interactive tables of chemical data. might the different properties of the two K1-2 Using the basic search option in Knovel, materials cause in producing the part? find as much physical and thermodynamic 1-21 Investigate the origins and applications for a data associated with ammonium nitrate as material that has been invented or discov- possible. What applications does this chem- ered since you were born or investigate the ical have? development of a product or technology Using the Basic Search, find the formula for that has been invented since you were born the volume of both a sphere and a cylinder. that was made possible by the use of a novel Using the Data Search, produce a list of material. Write one paragraph about this five chemicals with a boiling point between material or product. Provide a list of the ref- 300 and 400 K. erences or websites that you used. You must K1-3 Using the Equation Plotter, determine the use at least three references. enthalpy of vaporization of pure acetic acid at 360 K. Problem What is the pressure (in atm) of air with a temperature of 200˚F and a water content of 10–2 lb water/lb air? All problems in the final section of each chapter require the use of the Knovel website Find three grades of polymers with a (http://www.knovel.com/web/portal/browse). melting point greater than 325˚C. Copyright 2010 Cengage Learning, Inc. All Rights Reserved. May not be copied, scanned, or duplicated, in whole or in part. Diamond and graphite both consist of pure carbon, but their materials properties vary considerably. These differences arise from differences in the arrangements of the atoms in the solids and differences in the bonding between atoms. Covalent bonding in diamond leads to high strength and stiffness, excellent ther- mal conductivity, and poor electrical conductivity. (Courtesy of Özer Öner/Shutterstock.) The atoms in graphite are arranged in sheets. Within the sheets, the bonding between atoms is covalent, but between the sheets, the bonds are less strong. Thus graphite can easily be sheared off in sheets as occurs when writing with a pencil. (Courtesy of Ronald van der Beek/Shutterstock.) Graphite’s thermal conductivity is much lower than that of diamond, and its electrical conductivity is much higher. Copyright 2010 Cengage Learning, Inc. All Rights Reserved. May not be copied, scanned, or duplicated, in whole or in part.