IB D1.3 Mutation and Gene Editing PDF 2025
Document Details
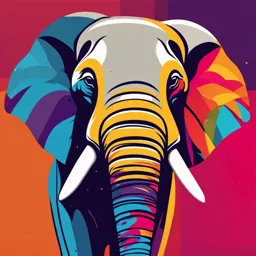
Uploaded by DesirousPraseodymium
2025
IB
Tags
Summary
This document is a set of lecture notes or study materials about D1.3 Mutation and Gene Editing for the IB program in 2025. It covers the different types of mutations, their consequences, and their role in genetic variation.
Full Transcript
First Exams 2025 D1.3 Mutation and Gene Editing Theme: Continuity and Change Level of Organisation: Molecules SL and HL Content IB Guiding Questions How do gene mutations occur? What are the consequences of gene mutation? SL and HL Content SL & HL Content: D1....
First Exams 2025 D1.3 Mutation and Gene Editing Theme: Continuity and Change Level of Organisation: Molecules SL and HL Content IB Guiding Questions How do gene mutations occur? What are the consequences of gene mutation? SL and HL Content SL & HL Content: D1.3: Mutation and Gene Editing D1.3.1: Gene mutations as structural changes to genes at the molecular level D1.3.2: Consequences of base substitutions D1.3.3: Consequences of insertions and deletions D1.3.4: Causes of gene mutation D1.3.5: Randomness in mutation SL and HL Content SL & HL Content: D1.3: Mutation and Gene Editing D1.3.6: Consequences of mutation in germ cells and somatic cells D1.3.7: Mutation as a source of genetic variation SL and HL Content SL & HL Key Terms Mutation Polymorphism Substitution Mutations Gene Insertion Mutations Allele Deletion Mutations Phenotype Point Mutations Codon Frameshift Mutations Mutagen Single-Nucleotide Radiation Polymorphisms Germ Cell SL and HL Content SL & HL Key Terms Somatic Cell Cancer Genetic Variation Natural Selection SL and HL Content D1.3.1: Gene mutations as structural changes to genes at the molecular level Distinguish between substitutions, insertions and deletions. SL and HL Content Gene Mutations Describe gene mutation Gene mutation involves changing the structure of genes at the molecular level. This is achieved by changing the nucleotide base sequence of the DNA. ❓ Distinguish between substitution, insertion and deletion mutations. Gene Mutations Distinguish between the three main types of mutation. Insertions and deletions affect one or more nucleotides whereas substitution usually affect a single base in the gene. Substitution mutations occur when one nucleotide in a DNA sequence is replaced by another nucleotide. Insertion mutations occur when one or more nucleotides is added to a DNA sequence. Deletion mutations occur when one or more nucleotides are removed from a DNA sequence. SL and HL Content D1.3.2: Consequences of base substitutions Students should understand that single-nucleotide polymorphisms (SNPs) are the result of base substitution mutations and that because of the degeneracy of the genetic code they may or may not change a single amino acid in a polypeptide. Base Substitution Mutations Base substitution mutation can result in one of three consequences; neutral, harmful or beneficial. Explain why Neutral mutations are mutations that have no effect. Base substitution mutations in non-coding DNA sequences usually do not have any effect so they are neutral. Non-coding sequences do not code for proteins Same-sense mutations are also considered to be silent mutations. This mutation occurs when a base substitution changes the codon but not the amino acid. This is possible because of the degeneracy of the genetic code. Base Substitution Mutations Harmful mutations are those that dramatically change protein structure, disrupting its normal biological activity. Nonsense mutations are considered harmful. This mutation occurs when a base substitution changes a codon for an amino acid into a stop codon, prematurely terminating translation. The resulting protein is unlikely to function properly. Missense mutations on the other hand can either be beneficial or harmful. This mutation occurs when a base substitution changes the codon for one amino acid into a codon for a different amino acid. Therefore one amino acid in the polypeptide is different. Many genetic diseases are due to mis-sent mutation, however a very small proportion of mis-sense mutations have beneficial effect SL and HL Content Single-Nucleotide Polymorphisms Single-nucleotide polymorphisms (SNPs) is a genetic variation that occurs when a single nucleotide is altered (base substitution) and kept through heredity such that more than 1% of the population has it. SL and HL Content D1.3.3: Consequences of insertions and deletions Include the likelihood of polypeptides ceasing to function, either through frameshift changes or through major insertions or deletions. Specific examples are not required. Insertion Mutations An insertion mutation adds one (or more) nucleotides to a gene. If multiples of 3 nucleotides are inserted into a gene, there will be extra codons in the mRNA leading to extra amino acids in the polypeptide. This can cause significant change to the structure of the polypeptide and prevent it from functioning properly. However, if the number of nucleotides inserted in a gene is not a multiple of 3, it results in a frame-shift mutation. Here, every codon is changed from the insertion point. This is because such mutations shift the reading frame for every codon beyond the mutation point. Therefore, the resulting protein is usually non-functional as many of its amino acids have been changed. DELETION Mutations A deletion mutation removes one (or more) nucleotides from a gene. If multiples of 3 nucleotides are deleted from a gene, there will be missing codons in the mRNA leading to missing amino acids in the polypeptide. This can cause significant change to the structure of the polypeptide and prevent it from functioning properly. However, if the number of nucleotides deleted from a gene is not a multiple of 3, it results in a frame-shift mutation. Here, every codon is changed from the deletion point. This is because such mutations shift the reading frame for every codon beyond the mutation. Therefore, the resulting protein is usually non-functional. Frameshift Mutations An insertion or deletion disrupts the reading frame of codons on a gene. All of the codons after the mutation will be changed. Polypeptides produced by frameshift mutations are unlikely to function, as many codon changes produce a different sequence of amino acids. Since the genetic code is read in codons, the addition of even one nucleotide can disturb the grouping of codons The polypeptide will have a different shape, and will not be able to carry out its function, as the amino acid sequence determines the shape and function of a polypeptide. SL and HL Content D1.3.4: Causes of gene mutation Students should understand that gene mutation can be caused by mutagens and by errors in DNA replication or repair. Include examples of chemical mutagens and mutagenic forms of radiation. Causes of Gene Mutations Mutations happen naturally through errors in DNA replication or during the repair of damaged DNA. However, Mutagens are agents that cause permanent, heritable changes to the DNA of cells..They increase the frequency of mutation above the natural level. Mutagens: High Energy Radiation Mutagens can be different forms of radiation. High energy electromagnetic radiation such as ultraviolet light, X-rays and gamma-rays are all mutagens. Radioactive isotopes of elements release alpha-particles and beta-particles which are also mutagens. These radiations damage the DNA by stimulating the breaking of the hydrogen bonds between the bases. This results in the separation of the DNA strands. This is dangerous because during reconnection, the DNA High energy radiation damages DNA and is a mutagen bases may connect to different bases. Also, radiation exposure can cause breaks in the DNA strands, leading to fragmentation, resulting in replication Mutagens: Chemicals Many chemicals are mutagens as they react with and damage DNA. Tobacco smoke contains many mutagens including polycyclic aromatic hydrocarbons formed during burning of tobacco. Alkylating agents which are used in chemotherapy are mutagens. Tobacco smoke contains many mutagens SL and HL Content D1.3.5: Randomness in mutation Students should understand that mutations can occur anywhere in the base sequences of a genome, although some bases have a higher probability of mutating than others. They should also understand that no natural mechanism is known for making a deliberate change to a particular base with the purpose of changing a trait. SL and HL Content Mutations are Random Mutations occur randomly in any location within a genome, though some bases have a faster mutation rate than others. Cytosine is the nitrogen base that has the highest probability of mutating. Cytosine can spontaneously lose an amino group, transforming it into uracil. Also changes between C and T (pyrimidines) and between A and G (purines) happen more readily than other base substitutions which require the number of rings in the base to change. There is no natural mechanism for making a deliberate mutation to create a new allele for a gene, and change a trait. Mutations occur at random locations Read the linked article on the randomness of mutations that result in cancer. SL and HL Content D1.3.6: Consequences of mutation in germ cells and somatic cells Include inheritance of mutated genes in germ cells and cancer in somatic cells. SL and HL Content Consequences of Mutations Mutations can be neutral, beneficial or harmful. Most mutations are neutral as they occur within non-coding sections of DNA, which does not code for proteins. Some substitution mutations are neutral due to the degeneracy of the genetic code. Many mutations to genes are harmful, though few mutations may be beneficial to survival and reproduction of the organism. Mutations to Germ Cells and Somatic Cells Germ cells develop into gametes such as sperm or eggs. If a gene mutates in a germ cell, gametes may be produced that carry the mutation. The mutation in the gamete can be passed on to the offspring after fertilization. Therefore mutated genes in germ cells are hereditary. Inherited conditions such as sickle cell are a result of mutations in germ cells. Somatic cells are all cells which are not reproductive cells. Mutations in somatic cells are not passed on to offspring, as somatic cells are not involved in sexual reproduction. Mutations of genes that control the cell cycle such as proto-oncogenes and tumour suppressor genes can result in uncontrollable cell division. Rapid repeated division of the cell leads to the formation of tumours or cancer. These mutations often occur in somatic cells. While germline mutations (mutations in germ cells) don't directly cause cancer in the individual carrying them, they can increase cancer risk in their offspring. SL and HL Content D1.3.7: Mutation as a source of genetic variation Students should appreciate that gene mutation is the original source of all genetic variation. Although most mutations are either harmful or neutral for an individual organism, in a species they are in the long term essential for evolution by natural selection. Nature of Science: Commercial genetic tests can yield information about potential future health and disease risk. One possible impact is that, without expert interpretation, this information could be problematic. SL and HL Content Mutations and Natural Selection Natural selection is the differential survival due to the inheritance of traits that make an individual more likely to survive and reproduce. Natural selection causes evolution of species over time as the alle frequency of the population changes. Mutations and Natural Selection Genetic variation is a requirement for evolution by natural selection. Most mutations are neutral or are harmful to the individual, however mutation is required for the long-term survival of a species. Mutations can lead to the formation of new alleles and are therefore a key source of variation for natural selection, although there are other sources of genetic variation such as; recombination of genes by crossing over, independent assortment of chromosomes during meiosis, and random fertilization. The environment changes over time and species need to evolve in response. When the environment changes, Individuals with mutations that are advantageous to the environmental conditions are able to survive and reproduce and pass on the mutated gene or advantageous alleles to the next generation through natural selection. With time, the allele frequency of the population changes , leading to Commercial Genetic Tests Nature of Science: There are many commercially available genetic tests which provide a range of information on a person’s genome, including information on potential health and disease risk. A possible unintended consequence is excessive anxiety in those who find they are carrying a mutation that might cause disease. Another unintended consequence is that life insurance could then be very expensive for someone with genes that increase the risk of disease. The interpretation of these results without professional assistance could lead to misinterpretations, anxiety and inappropriate medical decisions. Furthermore, genetic test results may uncover sensitive information that could affect not only the individual but also their family members. SL and HL Content Review and Discuss: SL & HL Key Terms Mutation Polymorphism Gene Substitution Mutations Allele Insertion Mutations Phenotype Codon Deletion Mutations Mutagen Point Mutations Radiation Frameshift Mutations Germ Cell Single-Nucleotide Polymorphisms SL and HL Content Review and Discuss: SL & HL Key Terms Somatic Cell Cancer Genetic Variation Natural Selection SL and HL Content D1.3 Mutation and Gene Editing - IB Linking Questions How can natural selection lead to both a reduction in variation and an increase in biological diversity? How does variation in subunit composition of polymers contribute to function? Additional HL Content HL Content Only Additional HL Content: D1.3 Mutation and Gene Editing D1.3.8: Gene knockout as a technique for investigating the function of a gene by changing it to make it inoperative D1.3.9: Use of the CRISPR sequences and the enzyme Cas9 in gene editing D1.3.10: Hypotheses to account for conserved or highly conserved sequences in genes HL Content Only HL Only Key Terms Gene Knockout CRISPR-Cas9 Guide RNA (gRNA) Gene Therapy Conserved Gene Sequences Highly Conserved Gene Sequences HL Content Only D1.3.8: Gene knockout as a technique for investigating the function of a gene by changing it to make it inoperative Students are not required to know details of techniques. Students should appreciate that a library of knockout organisms is available for some species used as models in research. Gene Knockout Gene knockout is a genetic engineering technique where a targeted gene is inactivated or removed from an organism using specific nucleases. This allows scientists to study the function of the gene by observing changes in the phenotype of the organism Gene Knockout Organisms Scientists have created a vast living library of knockout organisms, where the organisms are genetically modified so they have non-functional versions of one specific gene. These knockout organisms are used as models for various research purposes. Mice: Mice libraries have been created for nearly all the mice coding genes. A strain of knockout mice was produced with non-functional versions of the leptin gene. These mice became very obese, showing that leptin regulates fat There are many lines of Knockout Mice deposition. Fruit fly: There are libraries of knockout fruit flies which cover most of the fruit fly genome. Zebrafish: Knockout libraries of zebrafish have been developed to study vertebrate development and disease. HL Content Only D1.3.9: Use of the CRISPR sequences and the enzyme Cas9 in gene editing Students are not required to know the role of the CRISPR–Cas system in prokaryotes. However, students should be familiar with an example of the successful use of this technology. HL Content Only D1.3.9: Use of the CRISPR sequences and the enzyme Cas9 in gene editing Nature of Science: Certain potential uses of CRISPR raise ethical issues that must be addressed before implementation. Students should understand that scientists across the world are subject to different regulatory systems. For this reason, there is an international effort to harmonize regulation of the application of genome editing technologies such as CRISPR. CRISPR-Cas9 CRISPR-Cas9 technology allows scientists to edit genes by modifying or deleting specific sections of DNA. CRISPR, which stands for Clustered Regularly Interspaced Short Palindromic Repeats. CRISPR are specific regions of DNA that are found in bacteria and contain short, repeated sequences and unique spacer sequences that are incorporated, usually from viral DNA encountered by the bacteria CRISPR-Cas9 CRISPR-Cas9 technology requires a CRISPR sequence, the enzyme Cas 9 and a guide RNA. Cas 9 is an endonuclease enzyme that was discovered in bacteria. It destroys viral DNA that enters the bacterial cells. During gene editing, the enzyme is used to cut the DNA target sequence. CRISPR Sequences: These are naturally occurring stretches of DNA in bacteria containing snippets of past invaders (viruses, plasmids) the bacteria encountered. They serve as a record of previous CRISPR-CAS9 Technology is a gene editing tool threats. This allows the bacterium to identify and destroy similar foreign DNA in future encounters using the CRISPR-Cas9 system Read the linked article on CRISPR-Cas9. Scientists design a guide RNA (gRNA) based on the CRISPR sequence that matches the desired target DNA CRISPR-Cas9 location. This is essential because the CAS9 would not function properly if the guide RNA is not based on a CRISPR sequence. The guide RNA is made by transcribing a “spacer” sequence and a “repeat” sequence from the CRISPR. The spacer sequence forms part of the gRNA that is complementary to the target DNA sequence. The repeat sequence on the other hand forms a distinct molecular shape that allows it to bind to Cas9 So when scientists identify the specific DNA sequence they want to target for editing, they explore the CRISPR sequences, looking for a spacer sequence with high similarity to the target DNA. The guide RNA is designed based on the chosen spacer How CRISPR works sequence and its accompanying repeat sequence. The sgRNA molecule is designed to specifically target and bind to a particular DNA sequence of interest, guiding the Cas9 enzyme to that location and enabling it to make precise cuts in the DNA, resulting in a double-strand break. Once the break has been made at a specific location in the DNA, scientists can add, delete or modify the DNA sequences at that point This can knock out the gene, or a DNA sequence can be inserted into the DNA where the gene was cut. Uses of CRISPR-CAS9 There are many potential uses of CRISPR-Cas9 technology including: Gene therapy: CRISPR-Cas9 can be used to replace or repair a gene that is responsible for genetic diseases such as sickle cell anaemia. Genetic research: CRISPR-Cas9 is an effective method to knock out genes, allowing researchers to better understand the action of specific genes. Malaria prevention: CRISPR-Cas9 can be used to modify the genomes of mosquitoes, which could reduce the spread of malaria. Curing Sickle Cell Anaemia and Improving Agriculture CRISPR-CAS9 can be used to repair the haemoglobin gene for blood stem cells removed from a patient with sickle cell anaemia.The stem cells can then be re-inserted into the patient with sickle cell anaemia, and cure them of the disease. Sicilian Rouge High GABA tomatoes are the first CRISPR-Cas9-edited crop plants sold for human consumption. They have four to five times more GABA, a neurotransmitter that can reduce blood pressure and aid relaxation. The tomatoes were engineered to have inactive glutamate decarboxylase, an enzyme that metabolizes GABA, allowing them to retain high GABA concentrations CRISPR-CAS9 can be used to cure sickle cell anemia until ripe. Ethical Issues and CRISPR The ethical implications of gene editing, particularly in humans and germ line editing, must be carefully considered before the widespread use of techniques such as CRISPR. International cooperation is necessary to address the potential global impact of gene editing, as some countries allow germline gene editing while others only allow it for research not reproduction. Special ethical concerns include safety, the potential for demands for enhancement purposes, and the lack of informed consent for future generations affected by germline editing. Ethical Issues and CRISPR Nature of Science: Scientists across the world are subject to different regulatory systems. For this reason, there is an international effort to harmonize regulation of the application of genome editing technologies such as CRISPR. HL Content Only D1.3.10: Hypotheses to account for conserved or highly conserved sequences in genes Conserved sequences are identical or similar across a species or a group of species; highly conserved sequences are identical or similar over long periods of evolution. One hypothesis for the mechanism is the functional requirements for the gene products and another hypothesis is slower rates of mutation. Conserved Gene Sequences Conserved sequences are identical or similar genes across a species or a group of species. Highly conserved sequences are identical or similar genes over long periods of evolution so may occur across a wider range of species. Mutations occur randomly at any location within a genome. Scientists are doing research on why certain gene sequences are conserved in many different species over long periods of evolutionary time, and mutations do not often appear in the conserved gene sequence. Conserved Gene Sequences Hypotheses which may explain the conservation of gene sequences include: Functional Constraint Hypothesis suggests that gene sequences are essential to the structure and function of the protein coded by the gene. Any mutation to the gene sequence is naturally selected against as the associated protein would not function. Slower Rates of Mutation Hypothesis suggests that highly conserved sequences are under selective pressure to maintain their function, which results in a slower rate of mutation in these sequences. HL Only Key Terms Gene Knockout CRISPR-Cas9 Guide RNA (gRNA) Gene Therapy Conserved Gene Sequences Highly Conserved Gene Sequences