Cre-Recombinase System PDF
Document Details
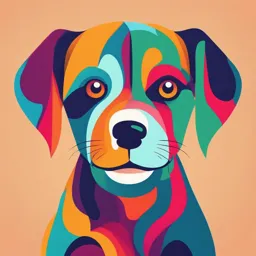
Uploaded by BriskAntigorite
Tags
Related
- A Concise History of the World: A New World of Connections (1500-1800)
- Odisha Sub-ordinate Staff Selection Commission Provisional Admission Letter PDF 2024
- Odisha Sub-ordinate Staff Selection Commission Provisional Admission Letter PDF, 2024
- Reliability Management PDF
- Odisha Sub-ordinate Staff Selection Commission Provisional Admission Letter PDF
- Functional Anatomy of Nervous System PDF
Summary
This document details the Cre-loxP system, a method for controlling and modifying gene expression in animal cells, including its mechanisms, methods, and applications. It covers gene knockout, activation, and lineage tracing.
Full Transcript
GROUP: Group 5 NAMES: Celina Castaneda, Antonette Cabral, Christine Errasquin, Micah Lorenzo, Reivic Valdez Cre Recombinase System Introduction Cre-recombinase (Cre-loxP) is a method of DNA recombination that can control and modify gene expression in animal cells, for instance, rat models. This tech...
GROUP: Group 5 NAMES: Celina Castaneda, Antonette Cabral, Christine Errasquin, Micah Lorenzo, Reivic Valdez Cre Recombinase System Introduction Cre-recombinase (Cre-loxP) is a method of DNA recombination that can control and modify gene expression in animal cells, for instance, rat models. This technique allows both the removal of existing genes from the genome and the inversion of sequences between recognized sites (Nagy, 2000). By combining cre recombination with transgenesis, many modifications to the genome become possible like eliminating gene expression of a specific gene and controlling when it occurs (conditional knockout). Figure 1. An overview of the Cre-loxP system. Cre recombinase protein recognizes the loxP sites of the 34 bp DNA recognition sequence (Kim et al., 2018) Cre recombinase is a topoisomerase produced from the Cre (referred to as the “cyclization recombinase”) gene of the P1 bacteriophage. It can catalyze changes between fragments of DNA sequences called loxP (which means “locus of X-over, P1”) sites (see Figure 1). The loxP sites have 34 base pair sequences. This system is capable of excising and adding genes to the genome as well as inducing the inversion and translocation of DNA between loxP sites (Kim et al., 2018). Methods: How does Cre-loxP system work? There are two components derived from P1 bacteriophage, the Cre recombinase protein and a loxP recognition site (Sternberg et al., 1986). The site-specific Cre recombinase is an enzyme protein responsible for intra- and intermolecular recombination events at the loxP recognition sites. The loxP site is a 34-base pair-long recognition sequence composed of two 13-base pair-long palindromic recognition sites separated by an 8-base pair-long core spacer sequence. The 13 base pair on each side is where the cre recombinase binds to proceed with the recombination process. Furthermore, this Cre-mediated recombination depends on the location and orientation of the loxP sites (Kim et al., 2018). The mechanism occurs when a Cre enzyme recognizes the loxP site and it initiates the site-specific recombination between those sites. Two directly repeated loxP sites are detected by a single Cre recombinase enzyme, and the Cre excises the loxP site sequence, thus resulting in an excised sequence – a circular and inactivated gene (Jian et al., 2002). The Cre-loxP system, which is usually utilized for genetic excision, can also be used for DNA inversion and translocation between two loxP sites, depending on the location and orientation of the loxP sites. LoxP sites can be arranged in cis (a certain distance apart on the same DNA strand) or trans (i.e., to different chromosomes) structures (Hadjantonakis et al., 2009). If loxP sites are positioned opposite of each other on the same DNA strand or in a cis-direction, then inversion happens which means that the sequence between the sites is reversed. If the loxP sites on the same strand are oriented in the same direction, it will result in deletion because the gene will be excised, creating a circular DNA thereby inactivating it. LoxP sites can also be found on different DNAs or trans-direction. This results in the translocation or insertion of the targeted gene from one DNA to another as illustrated in Figure 2 (Nagy, 2000). Figure 2. (a) 34 bp consensus sequence of loxP and (b-d) the associated Cre-mediated recombination processes based on the location and orientation of the loxP sites (Fuller et al., 2015). There are two primary methods for expressing Cre recombinase in a cell. The first method is to utilize a virus to inject the Cre recombinase gene into the cell. The other approach involves breeding transgenic mice with the Cre recombinase gene inserted after a particular promoter. Researchers have discovered that Cre expression is not always restricted to the intended cells. Furthermore, knockout phenotypes are frequently seen in many cell types in Cre transgenic mouse strains due to their affinity for non-specific expression (Schulz et al., 2007). To generate Cre transgenic mice, the Cre construct is injected into a fertilized egg's pronucleus. The zygote is then implanted into a mouse. This mouse will produce a transgenic Cre mouse (Haruyama et al., 2009). When the transgenic line is born, it will function as a Cre stock. Shown in Figure 3 is a schematics for the generation of mice using the Cre-loxP technique that has mutations in the gene of interest (GOI). In theory, if a floxed DNA-carrying mouse breeds with a Cre-carrying mouse, the resultant offspring will have both flanked DNA and Cre. As a result, GOI will be inactivated and floxed loci excised by Cre recombinase (Picciotto & Wickman, 1998). Figure 3. An example of a Cre-lox system experiment in genetics shows that mating floxed mice only results in the removal of the premature stop sequence from cells that express Cre recombinase. As demonstrated in Figure 4, inducible Cre system techniques that use exogenous inducers and cell-specific regulatory elements allow for more precise gene modification using the Cre-loxP system. This is to improve the accuracy of genetic functional investigations and clinical uses of the Cre-loxP system. A tamoxifen (Tam) induced Cre system has three elements, the fused Cre recombinase and Estrogen receptor called CreER, Tam, and HSP90. CreER and HSP90 complex is present in the cytosol, upon adding Tam, HSP90 dissociates and CreER is transported to the nucleus. Then, CreER will recognize the targeted gene flanked by loxP sites and proceed with gene editing as seen in Figure 4a. On the other hand, another cell-specific inducible system is the tetracycline (Tet) system. A tetracycline derivative, Doxycycline (Dox), is far more cost-effective and practical in regulating the Tet receptor (TetR) than tetracycline. Doxycycline-dependent Cre system can happen in two ways, “Tet-On” and “Tet-Off”. For “Tet-On”, the important elements used are reverse tetracycline-controlled transactivator (rtTA), Dox, tetracycline operon (TetO7) or tetracycline responsive element (TRE). In the absence of Dox, as illustrated in Figure 4b, rtTA won’t be able to bind to TRE, hence, Cre won’t be expressed. Consequently, the target gene will not be excised. The addition of Dox will enable rtTA to bind to TRE, consequently, allowing the expression of Cre recombinase. Then, the deletion of the target gene will commence. For the “Tet-Off” mode, the same elements will be used but instead of rtTA, tetracycline-controlled transactivator (tTA) protein is used. The process is reversed. In the presence of Dox, tTA will not be able to bind to TRE, hence, Cre expression is inhibited. On the other hand, in the absence of Dox, tTA binds to TRE enabling the expression of Cre recombinase. Therefore, the target gene is excised and inactivated as shown in Figure 4c (Kim et al., 2018). Figure 4. Inducible Cre-loxP systems featuring (a) Tamoxifen, a selective estrogen receptor modulator and (b-c) Doxycycline-dependent gene activation and activation (Kim et al., 2018). Applications Cre-loxP recombinase system is a widely utilized technique for modifying mammalian genes. It involves relatively simple manipulation and doesn't need extra components for effective recombination. Gene in vivo knockout techniques are widely used in molecular biology labs. Conditional Gene Knockout This technique employs the Cre-loxP recombinase system in selectively inactivating target genes in specific cell types in specific tissues, at certain developmental stages of an organism, or in specific conditions (Inducible gene expression). A target gene is altered for conditional mutagenesis by the insertion of two loxP sites, which allow the flanked (floxed) gene segment to be excised through Cre-mediated recombination (Friedel et al., 2011). Gene Activation On the contrary, instead of “knocking out” target genes from the genome of an organism, this technique is the selective “turning on” of specific genes. Additionally, one of the widely used applications of Cre recombination is the removal of the preceding transcriptional stop signal to permanently activate marker genes, such as reporter genes or antibiotic resistance genes (Bapst et al., 2020). Lineage Tracing This refers to the set of methods allowing for the tracking or tracing of individual cells' fates and those of their progeny with the least possible disruption of their physiological function. It has been extensively used to delineate or unravel complex biological processes involving multiple cell types with different lineage hierarchies (Wu et al., 2019). In relation to the Cre-loxP system, the cells are labeled through permanent marking or modification, which can allow researchers to gain insights into concepts such as cell fate determination and the developmental processes involved. Genetic Mosaic Analysis and Gene Therapy A genetic mosaic is defined as an individual in which different cells have various genotypes. In developmental studies, to determine where in the animal a gene of interest must be expressed to have a certain phenotypic effect, Caenorhabditis elegans genetic mosaics have been produced, recognized, and evaluated (Yochem & Herman, 2005). Essentially, genetic mosaics can provide insight into the cell specificity of gene function. Consequently, the use of the Cre recombinase system may help in research involving lineage tracing, mechanisms of diseases, and eventually gene therapy. References: Bapst, A. M., Dahl, S. L., Knöpfel, T., & Wenger, R. H. (2020). Cre-mediated, LOXP independent sequential recombination of a tripartite transcriptional stop cassette allows for partial read-through transcription. Biochimica et Biophysica Acta (BBA) - Gene Regulatory Mechanisms, 1863(8), 194568. https://doi.org/10.1016/j.bbagrm.2020.194568 Fuller, P. M., Yamanaka, A., & Lazarus, M. (2015). How genetically engineered systems are helping to define, and in some cases redefine, the neurobiological basis of sleep and wake. Temperature, 2(3), 406–417. DOI: 10.1080/23328940.2015.1075095 Friedel, R. H., Wurst, W., Wefers, B., & Kühn, R. (2010). Generating conditional knockout mice. Methods in Molecular Biology, 205–231. https://doi.org/10.1007/978-1-60761-974-1_12 Hadjantonakis, A. K., Pirity, M., & Nagy, A. (2009). Cre recombinase mediated alterations of the mouse genome using embryonic stem cells. Molecular Embryology: Methods and Protocols, 111-132. DOI: 10.1007/978-1-60327-483-8_8 Haruyama, N., Cho, A., & Kulkarni, A. B. (2009). Overview: engineering transgenic constructs and mice. Current Protocols in Cell Biology, 42(1), 19-10. DOI: 10.1002/0471143030.cb1910s42 Jian, M., Spicher, K., Boulay, G., Martín-Requero, Á., Dye, C. A., Rudolph, U., & Birnbaumer, L. (2002). Mouse gene knock-out and knock-in strategies and methods as applied to the alfa-subunits of the Gi/G0 family of G-proteins. DOI: 10.1016/S0076-6879(02)44721-0 Kim, H., Kim, M., Im, S.-K., & Fang, S. (2018). Mouse Cre-LoxP system: general principles to determine tissue-specific roles of target genes. 34(4), 147–147. https://doi.org/10.5625/lar.2018.34.4.147 Nagy, A. (2000). Cre recombinase: the universal reagent for genome tailoring. genesis, 26(2), 99-109. DOI:10.1002/(SICI)1526-968X(200002)26:23.3.CO;2-2 Picciotto, M. R., & Wickman, K. (1998). Using knockout and transgenic mice to study neurophysiology and behavior. Physiological reviews, 78(4), 1131-1163. DOI: 10.1152/physrev.1998.78.4.1131 Schulz, T. J., Glaubitz, M., Kuhlow, D., Thierbach, R., Birringer, M., Steinberg, P., ... & Ristow, M. (2007). Variable expression of Cre recombinase transgenes precludes reliable prediction of tissue-specific gene disruption by tail-biopsy genotyping. PLoS One, 2(10), e1013. DOI: 10.1371/journal.pone.0001013 Sternberg, N., Sauer, B., Hoess, R., & Abremski, K. (1986). An initial characterization of the bacteriophage P1 cre structural gene and its regulatory region. J. Mol. Biol, 187, 197-212. DOI: 10.1016/0022-2836(86)90228-7 Yochem, J., Herman, R. (2005). Genetic mosaics. WormBook, ed. The C. elegans Research Community. DOI:doi/10.1895/wormbook.1.58.1 Wu, S., Lee, J., Koo, B. (2019). Lineage Tracing: Computational Reconstruction Goes Beyond the Limit of Imaging. Mol Cells, 104–112. DOI: 10.14348/molcells.2019.0006