Biology 1A03 Notes - Cell Membrane Structure & Function PDF
Document Details
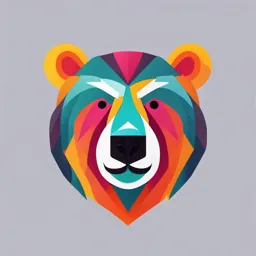
Uploaded by LionheartedCantor
Toronto Metropolitan University
Tags
Summary
These notes summarize the structure and function of cell membranes, discussing their composition, including phospholipids, proteins, and carbohydrates. It explores the movement of molecules across membranes, such as passive diffusion, and influences on membrane fluidity.
Full Transcript
THEME 1 MODULE 1: The Composition and Structure of Membranes Unit 1: Cells in Our Body 1) We began as a single cell, the result of the union of the egg and sperm 2) Mitotic cell divisions create 2 cells, then 4 cells, then eventually we have 10 trillion cells as an adult human a)...
THEME 1 MODULE 1: The Composition and Structure of Membranes Unit 1: Cells in Our Body 1) We began as a single cell, the result of the union of the egg and sperm 2) Mitotic cell divisions create 2 cells, then 4 cells, then eventually we have 10 trillion cells as an adult human a) Each of the cells vary and differ in function, there may muscle cells, skills cells kidney cells, and neurons b) All of these cells are eukaryotic cells: meaning they have a nucleus that contains most of the genetic material in the cell If we wanted to count the number of cells within our body what do we have to do? 1) There must be a common definition of the term cell 2) What is a cell? Do we mean prokaryotic cells, eukaryotic? 3) We carry a lot of passenger cells within our body, namely prokaryotic cells Eukaryotic cells: - Plant cells, animal cells, fungi Prokaryotic cells: - Bacterial cells There are an estimated 10 times as many bacterial cells in the human body as our own eukaryotic cells - Make up two to three % of our body mass - 150 pound person = 3-4.5 pounds of this weight comes from bacterial cells - Most of these cells are harmless and they are an important part of our bodies - Provide essential functions throughout our physiological systems - Some of these cells are potentially harmful but are contained or managed by our immune system Microbiome: a population of microorganisms or microbes - Organisms not visible to the naked eye, but only visible under a microscope - Prokaryotic bacteria, small eukaryotic organisms - Bacterial cells are found in every part of our body, they are on our skin, our teeth, in our digestive system and respiratory tract - 10 000 distinct species of microbes on and in a healthy human body Streptococcus Aureus (found on money): Able to cause skin and respiratory infections Streptococcus Salivarius: a normal inhabitant of the upper respiratory tract and oral cavity - A member of a collection of bacteria that contribute to the formation of dental plaque - One of the first microbes to colonize a germ-free newborn’s oral cavity and gastrointestinal tract - It resides on our skin and is pretty harmless if it stays there, but if it gets inside the body it can be pathogenic and lead to the activation of the immune system Staphylococcus haemolyticus - Resides on skin Bacteroides thetaiotaomicron: a predominant intestinal bacteria - Makes enzymes that are useful in the breakdown of plant material that we can ingest such as oat fiber Unit 2: Phospholipids are the Building Blocks of Membranes 1) All cells are surrounded by a membrane the contains phospholipids Cell: A membrane-bounds structure containing macromolecules 1) Nucleic acids (DNA & RNA): Hereditary material of the cell and are found in the DNA; chromosome/nucleoid. The information encoded in the DNA is converted into functional products of cells (RNAs and eventually proteins) 2) Proteins: Make up the structural elements of the cell (flagellum on bacterium) and perform metabolic activities (through ribosomes that synthesize enzymes) 3) Polysaccharides/carbohydrates: Important structural components of many cells (bacterial capsule or plant cell wall) and sources of stored energy (foods made from wheat or rice) 4) Phospholipids: Essential part of the cell membrane - an essential component of our definition of a cell Cell Membrane: Membranes separate an internal environment from the external environment. This allows these distinct environments to have different chemical composition. 1) This important to protect the cell from damaging toxins 2) To ensure the cell allows entry of important compounds 3) Disposal of metabolic wastes What components form the structure of a cell membrane? 1) It is made up of lipid macromolecules. Each of these macromolecule has hydrophobic (water hating) core and peripheral hydrophilic (water loving) properties that allow stacked lipid bilayers to form a) Water molecules interact with the head regions of the phospholipid, and as a result the tals will interact with tails to form hydrophobic cores and the hydrophilic heads will interact with water in the extracellular or intracellular environment b) Phospholipids can aggregate and form lipid micelles. These kinds of structures are important for i) Absorption of fat-soluble vitamins and complex lipids in the human body ii) The lipid aggregates form spontaneously without the use of any energy 2) The cell membrane is not thick and rigid, rather they are very thin, from 5-10 nm in thickness, so thin that 100 membranes stacked on top of each other is a piece of paper - Different lipids can be in cells - - Phospholipids consists of a glycerol molecule linked to a phosphate and 2 fatty acids, often called fatty acid tails - These hydrophobic tails make up the hydrophobic core of the bilayer - The hydrophilic head groups (glycerol + phosphate) form the hydrophilic surface that faces the aqueous environments outside and inside the cell. - Phospholipids are said to be amphipathic because they have both a hydrophobic and hydrophilic part. 1) Phospholipid tails consist of a pair of hydrocarbon chains a) There are 16 or 18 carbons in a single chain b) The bonds connection the carbons may be single bonds (saturated) or double bonds (unsaturated) c) These properties change the shape, size and behavior of the phospholipids 2) Steroids: Lipids characterized by a 4-hydrocarbon ring structure. Contain a hydrophilic head group and a hydrophobic tail. How do these components “behave” when they form membranes? What kind of barrier is the cell membrane: What can pass through the cell membrane and what can not? Unit 3: Organization of Cell Membranes Phospholipids are not stationary because membranes are fluid - They can move laterally within one layer of the lipid bilayer, left, right, backward, forward. - However, phospholipids cannot flip easily from one layer to the other in the bilayer without the use of a great amount of energy The fluidity of membranes can be affected by various factors 1) NUMBER OF CARBONS IN THE FATTY ACID TAILS : The number of carbons in the hydrocarbon chain varies: typically there are 16 or 18 carbons. The longer chains pack together more tightly than the shorter chains, reducing the fluidity of the membrane 2) UNSATURATED OR SATURATED FATTY ACIDS Double bonds within a hydrocarbon tail produce kinds or bends in the chain. This has the effect of pushing neighboring phospholipids further apart and increasing fluidity. Unsaturated fatty acid tails can lead to kinds in the hydrophobic tails that affect overall permeability 3) TEMPERATURE External environmental factors such as temperature can also influence membrane permeability. Higher temperatures promote fluidity, while lower temperatures decrease fluidity. Cold-adapted organisms, interestingly tend to have more UNSATURATED phospholipids in their membranes that help to maintain fluidity 4) THE PRESENCE OR ABSENCE OF CHOLESTEROL Steroids such as cholesterol are found in the membranes of every cell in our body, making up about 50% of the molecules found in the lipid membranes. a) A lipid membrane containing just phospholipids is actually TOO fluid. Cholesterol molecules constrain fluidity of the membrane by packing closely to neighboring phospholipids. i) At low temperatures, phospholipid bilayers behave like many other fats; they begin to solidify ii) At these lower temperatures cholesterol helps to maintain fluidity by keeping the phospholipids apart from one another Membrane fluidity: ability of phospholipids to move. The fluidity of the cell membrane allows for transmembrane movements which includes movement of substances from exterior to the interior of the cell and the reverse. - Regions of the membrane high in saturated fatty acids are less permeable than regions of a membrane that contain high amounts of unsaturated fatty acids Region of lower fluidity: Lipid raft, it can sequester or hold macromolecules together in the membrane. These rafts are found to gather proteins involved in the same metabolic pathway or a collection of receptors on the surface of the cell. If the region of the lipid raft is taller - Longer phospholipid hydrocarbon tails - Are straight rather than kinked because they are saturated, allowing phospholipids to pack together lowering the fluidity, making it possible to hold the macromolecules in the raft - A high concentration of cholesterol decreases fluidity within the microdomain of the lipid raft Unit 4: Movement Across Cell Membranes Macromolecules can move laterally within the cell membrane. - The fluidity of the cell membrane allows for transmembrane movements which includes movement of substances form the exterior to the interior of the cell and the reverse - Changing the fluidity will alter how much and how quickly a substance will move across the membrane. Regions of the membrane high in saturated fatty acids (lipid raft) are less permeable than regions of a membrane that contain a high amount of unsaturated fatty acids. Cell Membranes - The ability of cell membranes to control the traffic of substances into and out of the cell and its organelles makes them selectively permeable - Small molecules and ions can cross the membrane along a concentration gradient (from high to low concentration) through a process called diffusion - Small and nonpolar molecules or hydrophobic molecules can pass through the phospholipid bilayers relatively quickly - Larger POLAR substances have greater difficulty moving across the lipid bilayer of the cell membrane if at all How do lipid insoluble molecules and ions get across the cell membrane? 1) Cell membranes are not exclusively made up of lipid macromolecules 2) Rather cell membranes are made up of lipids, proteins, and carbohydrates a) Most have carbohydrates that can be attached to lipids or proteins b) The lipid bilayer is attached with sugar residues, glycolipids, and interspersed steroid molecules such as cholesterol, and many protein channels Membrane-associated proteins can be attached to the cell membrane on the interior or the exterior of the cell, or actually embedded in the phospholipid bilayer 1) When considering movement of molecules in and out of the cell, it is the transmembrane proteins (or integral membrane) embedded within the cell membrane that enable the transport of hydrophilic molecules across the cell membrane The fluid mosaic model states that membranes consist of proteins and carbohydrates embedded in a fluid phospholipid bilayer. - The ability of membrane components to move laterally was found through a scientific model. A testable idea about the way a system works, where the predictions are consistent with the experimental observations made over the last 30 years. Unit 5: Mechanisms of Transport Across the Cell Membrane Passive Diffusion: from areas of high to low concentration (along a gradient) - Movement of small molecules in the direction of a concentration gradient - Molecule comes in contact with and crosses the phospholipid bilayer into or out of the cell Involves the movement of solute molecules 1) Lipid soluble molecules 2) Gasses 3) Uncharged polar molecules and even some water across the cell membrane without the need of any energy 4) Molecule comes in contact with and crosses the phospholipid bilayer into or out of the cell 5) Substances are moving from an area of higher concentration to an area of lower concentration 6) Even some water molecules 7) NO ENERGY REQUIRED Passive Transport (facilitated diffusion): from areas of high to low concentration (along a gradient) - Movement of small molecules with the concentration gradient but involves proteins embedded in the cell membrane and does not require energy - Transport proteins provide a hydrophilic core that allows large hydrophilic molecules most water to move across the cell membrane - Most water crosses the membrane through this way Most common way for substances to move passively across the cell membrane. 1) Generally requires the help of transmembrane proteins that span the width of the phospholipid bilayer 2) Is useful because the phospholipid bilayer is relatively impermeable to ions and most hydrophilic molecules 3) Requires no energy PASSIVE TRANSPORT OSMOSIS: The transport of water across membranes (NO USE OF ENERGY) 1) Water diffuses from a less concentrated solution (hypotonic, chamber A) to a more concentration solution (hypertonic, chamber B) 2) Focus on the concentration of solutes in the solution on either side of the selectively permeable membrane 3) The net movement of water is the process of equilibrating the osmotic concentrations on either side of the membrane PASSIVE TRANSPORT (FACILITATED) 1) The movement of water across selectively permeable biological membranes can go at a much faster rate due to aquaporin transmembrane proteins. 2) These protein channels exclusively allow water to pass through hydrophilic protein cores through the process of osmosis at over 10 times the rate water takes to move directly through the phospholipid bilayer Active Transport: molecules move against the concentration gradient (fueled by energy from the hydrolysis of ATP. - Movement of molecules against a concentration gradient and involves proteins embedded in the cell membrane which require energy from ATP to drive transport ISOTONIC ENVIRONMENT: Scenario One (Same extracellular fluid concentration with the same osmolarity as its interior) - No net movement of water - The cell retains its cell shape and optimal cellular activities - Red blood cells in isotonic solutions retain their concave disk-like shapes HYPOTONIC ENVIRONMENT: Scenario 2 (Lower solute concentration than the inside of the cell). - The net movement of water down its concentration gradient - Results in excessive movement of water into the cell and leads to the cell swelling or even nursing, when cells burst they become “ghost cells” HYPERTONIC ENVIRONMENT: Scenario 3 (an environment with a higher solute concentration on the exterior of the cell relative to the interior of the cell can lead to the net loss of water from the cell interior towards the exterior environment) - Cell membrane shrinks and shrivels ACTIVE TRANSPORT This type of movement requires energy which is provided by the hydrolysis of ATP molecules on the intracellular side of the cell - If the transmembrane transport protein is directly affected by the energy released from ATP hydrolysis, it undergoes a conformational change to pump the substance across the membrane against a concentration gradient (PRIMARY ACTIVE TRANSPORT) - Direct expenditure of ATP-dependent - IF NEIGHBORING transmembrane transport proteins take advantage of electrochemical gradients established by their primary active transport pumps to move their own solutes against the gradient, this is SECONDARY ACTIVE TRANSPORT Sodium Potassium Pump: Embedded in many cellular membranes is a classic example of primary active transport mechanisms 1) The pump is essential in allowing the cell to establish differences in the concentration of sodium and potassium on either side of the cell membrane, which is very important for biological processes a) ENERGY IS REQUIRED AND COMES FROM THE HYDROLYSIS of ATP b) In most cells there is a greater concentration of potassium in the interior of the cell relative to the extracellular environment of a cell in our body c) More sodium on the outside d) This concentration difference is maintained by the ACTIVE role played by the sodium-potassium pump How it works: 1) For every 3 sodium ions pumped out, 2 potassium ion are pumped from the extracellular environment into the interior of the cell 2) The energy molecule ATP gives up an energetic phosphate group to the transport protein because this occurs against the concentration gradient a) This energetic phosphate group that binds and causes a conformational change in the transport protein, and therefore allows the release of the sodium ions into the extracellular fluid in exchange for potassium b) When the sodium potassium pump binds 2 potassium ions that will enter the cell, the phosphate group is released from the transport protein and the protein returns to its original shape and recess potassium ions in the cytoplasm Net loss of positive ions (resting potential of the cell is slightly more negative) THEME 1 MODULE 2: THE STRUCTURE OF THE CELL, ORGANELLES AND ENERGY Unit 1, Comparing Eukaryotic and Prokaryotic cells Life began 4 billion years ago, it was exclusively unicellular for most of Earth’s history because oxygen was absent from the oceans and atmosphere - Harsh in hospital environment The first photosynthetic cells (could create oxygen) opened up diversity that led to the variation of organisms that exist today. - One of the most interesting time-points in the history of life was the appearance of the first photosynthetic cells, especially the first photosynthetic eukaryotes that evolved in the Proterozoic eon. - Many unicellular organisms began interacting with one another, and the interactions that allowed these organisms to deal with harsh environments until these organisms became co-dependent. Both have a: An external membrane that separates the inside of the cell from the outside of the cell. Eukaryotic cells: an organisms whose cells contain a nucleus and many membrane bound organelles - Internal network of membranes - Double membrane bound nucleus that serves as an internal compartment to separate the genetic material of the cell (chromosomes) from the rest of the cell interior - True compartmentalized nucleus Prokaryotic cells: unicellular organisms that lack a nucleus and have few to no organelles 1) Life on earth was almost exclusively unicellular for most of Earth’s history with oxygen virtually absent until the first photosynthetic cells 2) Prokaryotic cells have been around the longest, eukaryotic cells appeared about 2 billion years ago Early Eukaryotes: Single-celled organisms that contained organelles and internal membrane systems with distinct structures and functions. Organelles: Membrane-bound structures inside the cell Chloroplasts: In plant cells. Photosynthetic protists and plant cells contain chloroplasts, actively engage in photosynthesis, producing chemical energy from light energy. - Has a double membrane around its exterior and an interior filled with hundred of flattened and stacked membranes called thylakoids - Thylakoid stacks are organized into piles called grana - In these membranes, pigments and enzymes participate in photosynthesis Mitochondria: In animal and plant cells. Mitochondria generate energy in the form of ATP. Convert energy from the food we eat into energy that powers most cellular functions in our bodies. - Mitochondrial malfunction = exhaustion, a common symptom of mitochondrial diseases Unit 2: The Evolution of Chloroplasts and Mitochondria Photosynthesis: a process in plant cells and other organisms to convert light energy into chemical energy. Chemical energy is stored in the bonds of carbohydrate molecules - We rely on photosynthesis to produce sugars we consume in our diets Cellular respiration: a process used by plant and animal cells to release the chemical energy stored in the bonds of carbohydrate molecules and partially capture it in the form of ATP. - Chemical requirements of our bodies can be obtained from sugars we consume - We have between 50 to over a million mitochondria within one of our cells, depending on which type - Mitochondria have two membranes - Outer Membrane: Surrounds the organelle - Inner membrane: Connected to a series of sac-like structures called cristae. - It is in these membranes that most of ATP is synthesized in eukaryotic cells. How did mitochondria end up in our cells? - Dr. Lynn Margulis proposed that eukaryotic cells originated as communities of interacting entities that joined together - She thought prokaryotic cells were ingested or entered into a host cell, where over time the prokaryotes and eukaryotes developed a mutually beneficial interaction and became obligatory for host survival. - Mitochondria and chloroplasts are two distinct membrane bound compartments thought to have entered from external origins. Mitochondria - Look like bacterial cells - About the same size as bacterial cells - Have their own circular genome - Produce the enzymes necessary for protein synthesis Why is it advantageous to develop this type of endosymbiotic relationship? 1) The early atmosphere of the Earth was a hostile environment 2) Anaerobic ancestral eukaryotes had the capability of producing small amounts of ATP from carbon compounds, and the interactions with aerobic bacteria are able to produce high amounts of aTP from the same carbon compounds through a complex membrane system 3) It is likely that a mutually advantageous relationship developed when anaerobic eukaryotes engulfed aerobic bacteria a) The bacterium could supply lots of energy, while the eukaryote provide a safe environment with a supply of carbon compounds Endosymbiotic theory of organelle evolution: 1) A timeline that outlines how all cells were derived and modified from an ancestral prokaryote that contains genetic material of inheritance 2) Cell membrane allowed ancestral prokaryotes to compartmentalize the genetic information into a nucleus and obtain greater regulation of games and processing of proteins to become most ancestral eukaryotes 3) These cells containing nuclei and an endomembrane system became hosts to aerobic and photosynthetic prokaryotes (modern-day mitochondria and chloroplasts) 4) All eukaryotic cell types have mitochondria, but not all have chloroplasts Unit 3: What is the scientific evidence that supports the endosymbiotic theory of organelle evolution? A hypothesis or theory leads to a set of predictions. What predictions can we make if the endosymbiotic theory is an accurate representation of organelles such as the chloroplast and mitochondria? 1) One prediction is that there might be living examples of acquired endosymbiotic relationships which can be seen in eukaryotic organisms a) There are examples of eukaryotic organisms that acquire the ability to engage in photosynthesis only after they engulf a photosynthetic bacteria that will perform those functions (phagocytosis by an early eukaryote, first step in the origin of plastids) b) Green Algae cymbomonas is an example where living bacteria is maintained inside the protists and share their photosynthetic products with the protist host, both organisms benefit from this relationship These types of relationships are also seen in other animals. (temporary endosymbiotic relationships) 1) Corals (similar to the human microbiome) live symbiotically with microbes called dinoflagellates. They photosynthesize as long as they have light and nutrients. They don’t keep the products of photosynthesis but release them to coral. 2) Sea slugs take this relationship one step further, the sea slug consumes photosynthetic algae and harvests the chloroplast from the algal cells. These chloroplasts are maintained in the cells of the sea slug. Endosymbiotic relationships in the context of evolution 1) These temporary relationships must become permanent and heritable 2) When the host cells divides, then the organelles are distributed evenly between two daughter cells 3) Both mitochondria and chloroplasts contain their own circular genomes, such as found in bacteria, and analysis of the genes shows significant similarity to bacteria Different organelles existing within the cell allow for compartmentalization of different cellular functions. Organelles are a distinct domain within a cell with a specific function Different compartments contain: 1) A unique set of enzymes 2) Produce and contain different products Increased membrane surface area can 1) Increase the potential metabolic capacity across the membrane This is advantageous because: 1) Specific sets of enzymes responsible for specific biochemical functions can be kept in close proximity to each other 2) Chloroplast has all enzymes necessary for photosynthesis, mitochondria for aerobic respiration 3) Efficiency of chemical reactions is increases if enzymes, substrates, and products are concentrated in an organelle 4) Incompatible processes like synthesis and degradation can be kept separate and not interfere with each other Unit 4, How do these organelles function in the cell? A chloroplast that is actively engaging in photosynthesis, harnesses light energy and together with atmosphere carbon dioxide it makes important organic sugars and gaseous oxygen. The energy present in sugars can then flow into the remaining ecosystem. 1) Living things need energy from outside sources (non-photosynthetic organisms) because they lack chloroplasts and cannot harness light energy to make organic molecules 2) Much energy is provided through metabolism of organic molecules produced by chloroplasts in the plants that we consume directly, or by feeding animals that feed on plants 3) Cyclists intake sugars to synthesize ATP to power their muscle cells How do chloroplasts make energy? 1) Presence of sunlight, carbon dioxide, water, and the primary energy source being produced as carbohydrates as glucose and oxygen as a byproduct 2) During the photosynthetic cascade of events, light energy is transformed into chemical energy in the form of ATP and NADPH which then enters the Calvin Cycle 3) In the Calvin cycle NADPH and ATP are used to drive the reduction of atmospheric carbon dioxide into carbohydrates This cascade shows the important of light reactions and the calvin cycle to produce sugar and oxygen Carbohydrates are the most abundant sources of ATP that fuel our bodily processes 1) Formed by the polymerization monosaccharides through glycosidic bonds to form complex sugars 2) Monosaccharides include glucose, fructose, and galactose 3) Special proteins called enzymes can catalyze the condensation reaction between specific hydroxyl groups of certain monosaccharides to produce disaccharides such as lactose, sucrose, maltose and others (metabolism of carbohydrates requires proteins called enzymes) 4) The reaction occurs between the OH group of carbon 1 of one molecule and carbon 4 of another monosaccharide to give either a) Alpha 1-4 glycosidic linkage b) Beta 1-4 glycosidic linkage Polysaccharides are the structures that form when many monosaccharides are linked together 1) Simple disaccharides or more, or larger and more common polysaccharides like starch and glycogen 2) Starch is the storage polysaccharide found in all photosynthetic plants made up of a) Alpha 1-4 glycosidic linkages between alpha-glucose monomers Two types of starch include two types of polysaccharides 1) Unbranched amylose 2) Branched amylopectin 3) These differences in structure vary only based on the interactions between neighboring carbon atoms of each glucose monomer a) In amylopectin the branches formed by glycosidic linkages between the carbon 1 of one glucose molecule and carbon 6 of another b) It is often these starches that we consume and use as energy in our own bodies When starches are consumed, we store digested carbohydrates as highly branched glycogen polysaccharide helices. This stored glycogen energy can be broken into glucose through cellular respiration to produce ATP for necessary cellular processes. Our cells are dynamic 1) We require molecular fuel to support our activities 2) The molecular fuel that gives energy for all our cellular processes is derived from ATP a) Cells can generate ATP from the metabolism of simple sugar molecules (cellular respiration) i) 4 steps take place in cellular respiration that result in ATP synthesis ii) A given cell typically only contains enough ATP to fuel 30 seconds - 1 minute of cellular activity, meaning the evolution of fast glucose processing systems has occurred (they are constantly active and efficient) Glycolysis: Occurs in the cytosol of the cell and leads to the production of 2 molecules of ATP and 2 NADH molecules that act as electron donors Step 1 of Glucose Processing 1) Glycolysis results in the production of 2 molecules of a 3-carbon compounds called pyruvate (NADH) for each molecule of glucose 2) Following glycolysis, pyruvate is processed to form acetyl CoA, which is free to enter the mitochondrial matrix of the mitochondria, while also producing carbon dioxide and NADH 3) Once in the mitochondrial matrix, acetyl CoA is further processed in the Krebs cycle producing ATP, carbon dioxide, and additional electron donors in the form of NADH and FADH2. These electron donors will add up during glucose processing to facilitate production of ATP in the mitochondria Not much ATP is produced in the first 3 steps of glucose processing, not a whole lot of ATP is synthesized 4) The bulk of the electron donors are used in the electron transport chain in the inner mitochondrial membranes or cristae to produce another 32 molecules of ATP a) As a result for every glucose molecule that we process in our cells, we produce 36 theoretical ATP, with negligible amounts coming from glycolysis and the Krebs cycle and most ATP forming when electron donors NADH and FADH2 move through the protein complexes of the electron transport chain in the extensive inner mitochondrial membranes b) The transfer of these electrons allows protons to be pumped into the intermembrane spaces of the mitochondria i) Creates an electrochemical concentration gradient across the inner mitochondrial membranes that drives protons through the ATP synthase protein channels that are embedded in membranes ii) This results in the synthesis of 32 additional ATP Until recently it was thought 36 molecules of ATP were synthesized per molecule of glucose, the theoretical maximum, but recent research using more precise measurements suggests its closer to 30 ATP molecules per molecule of glucose A proton gradient drives the synthesis of ATP Carbohydrates are not the only source of energy used to make ATP 1) Proteins and lipids can be converted into components that enter into the cellular respiration pathway and lead to the production of ATP 2) All three of these macromolecules contribute to ATP production, carbohydrates are metabolized first, then fats, and then proteins Why is ATP a source of molecular energy? 1) It fuels cellular processes because it has a very high potential energy. ATP has 3 phosphate groups attached to a ribose sugar and an adenine. The 3 phosphate group shave 4 negative charges in close proximity, so these negatively charged phosphate groups repel each other, giving the electrons of the phosphate groups a very high potential energy 2) The potential energy of ATP can be released and harnessed during hydrolysis, where ATP can react with water and beak the bond between the outermost phosphate group and its neighbor resulting in the formation of ADP and inorganic phosphate 3) The hydrolysis of ATP is highly exergonic (releases energy) The cell’s interior machinery takes advantage of the energy that is released during atp hydrolysis to perform, mechanical transport, and chemical work - Cyclist pedaling uses ATP hydrolysis to fuel the contraction of his or her muscles during strenuous exercise - ATP is also important for the transport of substances across the cell membrane AGAINST a concentration gradient - Many enzymes within a cell require energy to perform their functions and the cell is then able to use ATP-derived energy to help drive endergonic or energy requiring reactions Today’s eukaryotes were likely derived and modified from an ancestral prokaryote that contained genetic material of inheritance. 1) The endosymbiotic theory states that these early eukaryotes became hosts to aerobic and photosynthetic prokaryotes which are now mitochondria and chloroplasts Endomembrane system: a group of organelles in eukaryotic cells that performs most lipid and protein synthesis; endoplasmic reticulum, golgi apparatus, and lysosomes THEME 1 MODULE 3: Proteins 1) TRANSPORT AND SIGNALING: Transport proteins embedded in the cell membrane - move substances across the cell membrane a) Certain proteins embedded in this membrane interact with carbohydrates for cell signaling b) Cytoplasmically situated proteins allow for amplification of these cell to cell signals 2) ENZYMES: Proteins can act as enzymes to speed up the synthesis of carbohydrates 3) ENZYMES: Enzymes in the mitochondria that allow for the release of stored energy from carbohydrates 4) MOVEMENT: Proteins also server a large part of structures that are important for moving an individual cell or large cargo molecules within the cell a) Actin and myosin that slide past each other in contractile bundles 5) DEFENSE MECHANISMS: Made by specialized immune cells and serve the role of antibodies that can destroy disease causing viruses and bacteria. Proteins have various functions. Their different sizes and shapes relate to their functions 1) TATA-box binding protein - interacts with DNA molecules, and is able to do so due to a groove in the protein that allows for this 2) Porin proteins - are important for the transport of water across the cell membrane and have a structural hydrophilic pore 3) Most soluble cellular proteins may take more globular shapes with a hydrophobic interior and hydrophilic exterior that allows the protein to be soluble in the aqueous environment of cytosol, such as hemoglobin Representing protein structures 1) Space-filling model a) Relative size and location of each atom, the different atoms are different colors 2) Ribbon Diagram a) The lines represent the backbone of the protein polymer b) The broader line represents an organized reiterated structure called an alpha helix while the thin line is a less ordered loop Unit 3 An Overview of Protein Synthesis: from DNA to Primary Protein Structures Basic principles of protein synthesis are the same for prokaryotic and eukaryotic cells 1) The genetic information for every protein is encoded in the DNA of the cell 2) First step is to transcribe that information into RNA which serves as the template for synthesis of a polypeptide 3) In the prokaryotic cells this is closely linked to translation of RNA into proteins by ribosomes 4) In the eukaryotic cell the primary RNA transcript is processed to messenger mRNA and is transported into the cytoplasm where it is translated into the protein by ribosomes The Nucleus 1) Contains most of the genes in eukaryotic cells 2) In this high-resolution electoral micrograph, the double-membraned nuclear envelope surrounds the nucleus and encloses the genetic material (chromosomes), separating the nuclear contents from the cytoplasm 3) The nucleus contains the nucleolus a) Nucleolus: Ribosomal RNA molecules are transcribed, an important component of ribosomes b) Before protein synthesis, the appropriate segment of DNA is read and transcribed into RNA in the nucleus The information in a gene (GNA) is transcribed into a copy (messenger RNA) Nuclear Envelope: has embedded nuclear pore complexes that allow for material to flow into and out of the nucleus. - Most ribosomal RNAs are manufactured in the nucleolus where they bind to proteins to form the ribosomal subunits. These structures get exported into the cytoplasm THE NUCLEAR PORES connect the nucleus with cytosol This nuclear pore complexes allow for 1) Transcribed RNA to exit the nucleus for further processing in extranuclear regions 2) Messenger RNA which has been transcribed from DNA carries the information to make proteins is also transported into the cytoplasm through nuclear pores 3) They also allow the important building blocks of DNA and RNA into the nucleus along with enzymes that are important for the transcription of DNA into RNA Cellular Machinery involved in synthesis of proteins: Ribosomes 1) Structural components of ribosomes are made in the nucleolus and shipped out 2) These ribosomes then assemble and have both large and small subunit components that contain RNA molecules and proteins 3) Remain soluble in cytoplasms as free ribosomes or can attach to the endoplasmic reticulum to become bound ribosomes a) Produce two different types of ribosomes that can produce different proteins and be destined for different parts of the cell or even secretion Cells produce large numbers of distinct proteins, depending on the cell you’re looking at there can be a few hundred to thousands 1) Derived from 20 core amino acids a) A central carbon atom bound to an amino group b) A carboxyl group c) A hydrogen atom d) Variable side chain ( R ) 2) Amino acid monomers are put together to make linear strands called polymers or polypeptides a) A polypeptide is a strand of amino acids that is covalently bound to one another through condensation (releases water) - condensation polymerize amino acids b) Bond can be broken through hydrolysis (requires addition of water) 3) Unique sequence of amino acids in each proteins is the primary structure of that protein Translation occurs inside the ribosome 1) The condensation reactions that join amino acids are catalyzed within the ribosome 2) The polymer of amino acids (green connected blocks) a) The specific sequence of the amino acids polymer is determined by translating the information in the mRNA (yellow block) in the clef between the large and small units of the ribosome 3) rRNA helps this process of translation occur. This molecule matches the sequence of nucleotides on the mRNA and carries the appropriate amino acid to the next position in the growing polypeptide chain. During the translation by a ribosome 1) Amino acids polymerize to form the primary structure when a bond forms between the carboxyl group of one amino acids and the amino group of another 2) The C-N bond that results from the condensation is called a peptide bond 3) When amino acids are linked together, the amino acids are referred to as residues and the polymer is called a polypeptide 4) The R value determines the amino acids distinct set of properties a) Some side chains are hydrophobic, some hydrophilic b) Hydrophobic chains may aggregate in aqueous environments as a result of thermodynamically stable hydrophobic interactions c) Hydrophilic side chains may be charge-polarized and capable of forming ionic bonds, while other side chains favor the formation of hydrogen bonds (these interactions between side chains determine and allow the formation of a stable three-dimensional protein) Once translation of mRNA to a polypeptide has occurred, the final folded and functional proteins may have different destinations depending on which ribosomes translate the mRNA to the proteins 1) If synthesized by ribosomes in the cytosol (free ribosomes), they will remain in the cytosol or be targeted to various cell organelles 2) Proteins that remain in the cytosol will include the enzymes involved in glycolysis or serve as the proteins that make structural components of the cell (actin or myosin) 3) Other proteins that are synthesized by free ribosomes can be transported out through nuclear pores into the nucleus to act as histone proteins or transcription factors (can head towards the mitochondria or chloroplasts and can serve the role of many membrane proteins in these organelles) 4) When proteins are targeted to organelles, it is a special sequence of the protein that targets that particular protein to an organelle Unit 3: Protein Folding Once a polypeptide is synthesized, how does it obtain the shape required for its function? 1) The side chains of amino acid residues (hydrophilicity or hydrophobicity) determine the three-dimensional shape of the protein 2) The three dimensional folded shape of the polypeptide is essential to allowing the proper function of the protein to be performed a) Whether synthesized by free or bound ribosomes, all proteins take up a three-dimensional folded shape 3) Globular cytosol protein a) The exterior is hydrophilic, which allows for its solubility in cytosol and its core is hydrophobic b) Similar protein folding occurs by proteins that are formed by bound ribosomes (continue through to the ER into the aqueous lumen of the ER, where they become folded and functional) Proteins Primary Structure 1) Determines the manner in which the protein folds 2) When looking at a polypeptide strand where each acid monomer is represented by a rectangle, the three-dimensional shape of proteins can be simplified by looking at the backbone and making a ribbon diagram Linear polymer is folded into a 3-D protein - The green polymer is distinguished by 3 figures - A spiral - A broad line with an arrowhead and a thin line Once a mRNA molecule is translated into a protein, that polypeptide begins a folding cascade determined by the properties of the amino acids making that polypeptide. SECONDARY STRUCTURES (TWO TYPES) The spirals in the ribbon diagrams are alpha helix. 1) The thick band represents the backbone and indicated side chains 2) Amino and carboxyl groups are attached by covalent bonds called peptide bonds 3) The polymer is turned into a spiral through hydrogen bonds formed between the carbonyl of the carboxyl group one amino acid and the amide of the amino group of another amino acid residue four positions away 4) All variable side chains (r- groups) stick out from the helix, determining the properties of the structure as a whole Beta Sheet 1) Arrowheads in the ribbon diagram 2) Made up of parallel protein strands with hydrogen bonds formed between carboxyl and amino groups of adjacent strands 3) Unlike alpha helices, the beta pleated sheets have hydrogen bonds that allow for the pleated like structure Interactions between components of the protein backbone are responsible for the formation of alpha helices and beta sheets. 1) Overall shape/tertiary structure is due to interactions between variable side chains and R-groups (allows bending and folding that makes the 3D shape) a) Types of interactions that occur are b) Hydrogen bonds c) Van der Waals interactions d) Covalent and ionic bonds e) Disulfide bonds f) Hydrophobic interactions When does protein folding occur? 1) Some occurs spontaneously as the polypeptide leaves the ribosome 2) Through cellular mechanisms that assist in protein folding a) Molecular chaperones: Proteins bind to hydrophobic regions of the new polypeptide and prevent incorrect folding just long enough for the correct structure to form b) Chaperonins: Large molecule complexes that form isolation chambers. Inside the chamber, a single nascent protein is sequestered from other proteins so it can fold without interference and incorrect bond formation Review: Using hemoglobin 1) Primary structure is synthesized from the mRNA that was transcribed from the globin genes 2) Based on the properties of the amino acids, these polypeptide chains fold into secondary structures such as alpha helices and beta sheets 3) The tertiary structures form because of interactions between R groups of the amino acids 4) To form the quaternary structure different polypeptide subunits interact to form the fully functional protein 1) Proteins synthesized by free ribosomes remain in the cytosol, what happens to proteins that need to be secreted from the cell? 2) Endomembrane system includes the nuclear envelope, endoplasmic reticulum, golgi apparatus, lysosomes, and allows for compartmentalization of the cell for functional roles a) Compartmentalization of the cell is seen in protein synthesis 3) There a free ribosomes in the cell but also bound ribosomes to the membranes of the rough ER that produce proteins which are able to enter the lumen of the ER for further protein processing and folding 4) How do proteins move through the Golgi apparatus to their final destinations? BOUND RIBOSOMES SYNTHESIZE PROTEINS IN THE ER mRNAs that encode proteins destined for the endomembrane system have a 1) Special signal sequence that causes ribosomes to become bound to the ER 2) This special sequence is synthesized by the ribosomes at the initial part of the protein 3) One the signal sequence appears, it binds a signal recognition particle (SRP) to a signal recognition particle receptor (SRPR) in the ER membrane 4) Once this interaction occurs the polypeptide can continue to be translated and into the lumen (central canal) of the ER a) The signal sequence is removed here b) The translated polypeptide can now undergo important changed that allow for the final stages of protein processing and maturation From this pathway alone 1) Some proteins will remain the ER 2) Many proteins will continue to their final destination through vesicles a) Small membrane-bound compartments in the cell that bud off from the ER b) ER is the site where proteins get modified by the addition of the carbohydrate chains (glycosylation) i) Glycosylation occurs on most secreted and membrane bound proteins that can contribute to protein stability, folding, and even cell to cell recognition c) Once released from the ER, the proteins goes to the golgi apparatus d) Some glycosylation directions happen in the lumen of the ER which some happen in the golgi apparatus e) Proteins get transported to the golgi apparatus in vesicles which pinch off from the ER and the vesicles can fuse and deposit their contents into the lumen of the golgi apparatus Products from the ER onto the Golgi apparatus (modified as they pass through the apparatus, after passing through proteins are shipped out in new vesicles) 1) Further protein modification occurs a) Including addition of carbohydrate groups (the golgi apparatus might be the home of the protein, but some proteins will travel farther into the endomembrane system of the cell) i) Porins , receptors will go to cell membrane ii) Antibodies, hormones, enzymes will be secreted out iii) To other organelles like the peroxisome, the lysosome, or vacomes How is it that proteins are sorted to each of these destinations? 1) Each protein that comes out of the golgi apparatus has a TAG, that allows for it to be packaged into a particular type of transport vesicle a) The transport vesicles have their own tags that allow them to go to their own destinations (the cell membrane, lysosome or back to the ER) 2) Once vesicles reach their destination a) The vesicle phospholipid bilayer fuses with that of the cell membrane, lysosome or ER b) Releases soluble proteins, or even embedded transmembrane proteins to their target How are these vesicles able to reach their destination? 1) Structural and motor components of the cell play an important role in being transport vesicles toward the cell membrane and in reverse towards the nucleus 2) The cytoskeleton is a dense network of fibers that helps maintain and change cell shape a) One cytoskeletal component is a microtubule: protein polymers that form long fibers which stretch through the cell and function as cellular roadways that allow vesicles to be transported along b) Another cytoskeletal component is motor proteins: such as kinesin and dynein that have the ability to attach a transport vesicle and then walk along microtubules using energy released from ATP hydrolysis Conclusions 1) Many soluble proteins are synthesized and folded in the cytosol where they continue to function 2) Other proteins are transported to various destination in the cell 3) Proteins destined for the mitochondria, chloroplast, and peroxisomes are synthesized on free ribosomes 4) In other cases proteins fold before transport to the organelle, and others after transport 5) Some proteins are targeted to the aqueous interior of an organelle (lumen), while others are embedded in membranes of the organelle How does protein structure relate to function? 1) Case study of Aquaporins a) Important proteins embedded in the plasma membrane that allow for the movement of water from on side of the cell membrane to the other side b) In all kingdoms of life (bacteria, animals, and plants) c) Hydrophobic exterior that allows for embedding in the cell membrane, a hydrophilic core that allows for passage of water from one side of a cell to another 2) Made up of a) 4 protein subunits (monomers), that together form the turmeric aquaporin channel (quaternary structure) b) Each subunit contains membrane-spanning alpha-helices that form a central pore i) Each monomer forms a functionally independent water pore that allows water to move through in either direction ii) Centralized core allows for several water molecules to move simultaneously through the channel c) Aquaporin proteins do not undergo any changes why transporting water, rather each water molecule is able to form specific hydrogen bonds with hydrophilic amino acid side groups in that line the core of the monomer, displacing nearby water molecules ahead in the channel Prokaryotic cells have membrane pores as well! 1) This bacterial porin has the use of beta sheets to form a barrel with a hydrophobic exterior and hydrophilic interior 2) This porin structure is found in the membranes of mitochondria and chloroplasts a) Evidence for prokaryotic ancestry Conclusions 1) Genetic information needed to produce proteins is encoded in our DNA 2) CF is a genetic disease that causes accumulation of mucus in many organs a) Linked to mutations that lead to malfunction of an important CF transmembrane conductance regulator (CFTR) ion channel protein i) Results in accumulation of thick mucus THEME 1 MODULE 4: Nucleic Acids Unit 1: the nucleic acids The fourth class of macromolecule found in all cells includes the nucleic acids: deoxyribonucleic acid (DNA) and ribonucleic acid (RNA). Where do we find DNA in the cell? 1) In prokaryotic cells, the majority of DNA is in the nucleoid and in small circular DNA molecules called plasmids (often carry one two genes). E.g. Genes for antibiotic resistance a) Plasmids can replicate independently of the core genome and be transferred from one cell to another i) This allows for the rapid spread of genes that confer properties such as antibiotic resistance in a bacterial population Chromosomes: Describes the organization of a double-stranded DNA molecule in association with proteins and RNAs 1) Eukaryotic cells have large linear chromosomes 2) Prokaryotic cells have smaller circular chromosomes, though exceptions have been observed 3) Organelles like the mitochondria have their own small chromosomes The circular chromosomes E.coli has supercoiled into about 100 loops and associated proteins to form a nucleoid in this photo 1) This allows a bacterial chromosomes that may be 1000x larger than the diameter of a cell to fold up within the nucleoid Supercoiling of DNA in a circular molecule is the coiling that occurs in addition to the coil of the helical DNA structure. 1) Preserves the double helix structure and compacts DNA into a small space In recent years a large number of prokaryotic genomes have been completely sequenced (400 bacterial strains since 2013) 1) This helps create maps that illustrate the position of all genes on the prokaryotic chromosome 2) E. coli is a common bacterium with a circular chromosome which carries genes necessary for normal function + has small circular plasmids 3) The plasmids contain a limited number of genes that may be required for survival in distinct environments such as in the presence of antibiotics This genomic map illustrates the position of the genes on the prokaryotic chromosome. There is a cluster of genes (operon) involved in the metabolism of the sugar, lactose, and another cluster of genes required for the synthesis of the amino acid, leucine. How did scientists discover that DNA was the molecule that contained genetic information? Streptococcal bacteria are normal inhabitants of the human upper respiratory tract. 1) Benign strains are harmless and not associated with any disease symptoms. 2) Virulent strains, however, are associated with mild symptoms, such as the sore throat resulting from Strep throat or as the severe symptoms resulting from pneumonia. Fred Neufeld made a number of observations in the 1920’s. 1) There are two strains of the bacteria, Streptococcus pneumoniae. a) One strain, when injected into the mouse, caused death.The S strain is a virulent form of the same bacteria. b) The other strain when injected into the mouse had no effect. The R strain is a harmless or benign member of the microbiome, in this case, the mouse microbiome. The question is, can information that determines the nature of the strain, whether benign or virulent, be transferred from one bacterial cell to another? To answer this question, an experiment was performed. In 1928, Frederick Griffith reported the results of his experiment in what is now called The Griffith's Experiment. The process of The Griffith’s Experiment 1) Virulent Bacteria is heated to high temperatures in order to kill them (heating foot to kill bacteria although the macromolecules are still there) 2) When dead S-Strain bacteria was injected in a mouse the mouse was fine Griffith hypothesized that the information that makes a cell virulent was still present, but that the cell carrying that information had been killed. Could that information still be used? To modulate this he set up an experiment with 3 controls 1) Injecting the virulent strain should kills the mouse 2) Injecting the benign strain or 3) The heat-killed virulent should not affect the mouse To test this hypothesis (3) he incubated remains of killed cells from the virulent strain to living cells from the benign strain and injected the combination into a the mouse 1) The mouse died 2) Benign cells were virulent 3) Griffith concluded that the cells of the benign strain had acquired information, or the ability to be virulent from dead cells, but how? What macromolecules carried hereditary information? This process is called transformation: a change in cell behavior resulting from the incorporation of hereditary material from outside of the cell 1944 (Oswal Avery and his colleagues looked at the work of Griffith) 1) Asked the question about which macromolecule in the bacterial cell was holding all the information that could cause transformation 2) Which molecule was carrying the hereditary information? 3) There are five macromolecules in the cell that could be considered: a) Lipids b) Carbohydrates c) Protein d) RNA e) DNA f) Lipids and carbohydrates were discounted. That left proteins, RNA, and DNA. Avery and his colleagues selectively eliminated each type of macromolecule from the cell extracts and then tested the remaining molecules for their ability to induce transformation. 1) RNase is an enzyme that specifically degrades RNA molecules; DNase degrades DNA molecules; protease degrades proteins. 2) Researchers included the important control. In the first flask, the virulent heat-killed bacterial extracts are incubated with the nonvirulent bacteria with the expectation that transformation should occur. 3) As expected, in the control group, the untreated extract could transform nonvirulent cells into virulent cells 4) In the absence of protein or RNA but in the presence of DNA there was still transformation 5) In extracts that had enzymes that destroyed DNA, they were unable to transform benign bacterial cells How does DNA Look like 1) X-ray diffraction experiments carried out by biophysicist and x-ray crystallographer Rosalind Franklin a) Aims x-rays at DNA to create images based on the diffraction of x-rays by the atoms of the DNA molecule b) Photo 51 was key to the theoretical structure of DNA where the helical nature was discovered DNA 1) Basic subunits are nucleotides 2) A single nucleotide consists of a) A phosphate group b) 5-carbon deoxyribose sugar c) Nitrogenous Base The deoxyribose sugar and phosphate group are constant in each base however 1) The four nucleotides that constitute DNA are chemically distinguished by the specific nitrogenous base found in the molecule a) Pyrimidines (cytosine and thymine) are aromatic heterocyclic molecules that have a single ring while b) Purines (guanine and adenine) consist of two rings: a pyrimidine ring fused to an imidazole ring. c) For this reason, purines are larger than pyrimidines. To assemble a DNA molecule 1) A condensation reaction releases a water molecule and forms a covalent bond called a phosphodiester bond (between two nucleotides) a) Linked along the phosphoribose backbone b) The phosphate group on the 5’ carbon of the deoxyribose forms a bond with the hydroxyl on the 3’ carbon on the next deoxyribose. The result is that the backbone has a 5’ to 3’ polarity created by these linkages and the nitrogenous bases stick out from the backbone. But we know that DNA is a double stranded molecule, how was this determined? 1) Biochemical work of Erwin Chargaff in the mid-1900’s. T a) This led to Chargaff's rules which state that DNA from any cell of all organisms should have a 1:1 ratio of pyrimidine and purine bases (this is the base Pair Rule) and, more specifically, that the amount of guanine is equal to cytosine and the amount of adenine is equal to thymine. b) This observation makes a powerful prediction about the structure of DNA. It suggests that there may be molecular pairing between purines and pyrimidines in the formation of DNA according to the rules: A pairs with T and C pairs with G. If Chargaff's rules are true, then a consistent spacing between two phosphoribose backbones should be maintained. 1) Further structural images from Rosalind Franklin's X-ray diffraction experiments suggested that the DNA molecule existed in a helical structure. 2) This led to the hypothesis that purines pair with pyrimidines in the DNA helix and that, based on the structure of the nitrogenous bases, the pairing allows for the creation of 2 hydrogen bonds between thymine and adenine and 3 hydrogen bonds between guanine and cytosine. The two strands run antiparallel to each other, with the bases facing inwards to give a diameter of 2.0 nm. The helix has a major groove and a minor groove, with one turn of the helix occurring every 10 nucleotides. RNA 1) Synthesized the same way as DNA a) Transcription of DNA sequence using the enzyme complex RNA polymerase i) Messenger RNA ii) Ribosomal RNA iii) Transfer RNA iv) Different enzymes (RNA polymerases) are used for the synthesis of different classes of RNAs To look at the information encoded in DNA to make proteins, three different classes of RNA molecules are required Two important differences between RNA and DNA 1) First, the 5-carbon sugar that makes up the backbone is ribose instead of deoxyribose. 2) Ribose has a hydroxyl (-OH) group on the carbon at position 2’, while deoxyribose has a hydrogen (-H) at position 2’. 3) We refer to the DNA nucleotides as deoxyribonucleotides and RNA nucleotides as ribonucleotides. The second difference between RNA and DNA is the nitrogenous bases used in RNA versus DNA. 1) While RNA ribonucleotides, like DNA deoxyribonucleotides, contain cytosine, guanine, and adenine, the pyrimidine, uracil, replaces thymine in its ability to pair with adenine in RNA. 2) The difference is that uracil has a hydrogen where thymine has a methyl group. When transcribing the information from a DNA molecule, new ribonucleotides are matched to the DNA template by hydrogen bonding according to the rules: U to A, C to G. 1) In addition, the 3' hydroxyl (or 3'end) of the existing polymer attacks the high-energy phosphate bond of the new ribonucleotide. 2) As a result, phosphodiester bonds, much like those seen in a DNA molecule backbone, are also formed in the RNA backbone. Messenger RNA: is the RNA copy of genes coding for proteins, they are single-stranded molecules that are then translated into proteins by the ribosomes. 1) Shown here is the RNA polymerase that synthesized the single stranded RNA copy (green) of one strand, the template strand (red), of the double-stranded DNA molecule. A transfer RNA or tRNA is a functional RNA. This RNA is never translated. 1) The sequence of the RNA is such that it folds into a 3-dimensional structure held together by base pairing between the ribonucleotides. 2) Its function is to attach to amino acids and bring them to the ribosomes during translation. Ribosomal RNA (rRNA) is another functional RNA. 1) It is transcribed from the ribosomal genes of which there are many copies in the cell, and it is a very large proportion of the total RNA in a cell. There are 3 different ribosomal RNA molecules that together form integral components of the ribosome. DNA in eukaryotes 1) The DNA molecule in the eukaryotic cell serves the same function as in the prokaryotic cell. 2) The processes of transcription into RNAs in both prokaryotic and eukaryotic cells are essentially the same. 3) The DNA codes for genes that are transcribed into the functional RNAs: tRNA and rRNA, as well as mRNAs that are templates for protein synthesis. The DNA molecules in the eukaryotic nucleus are linear molecules that are organized around proteins (called histones) to form chromatin. 1) There are several levels of organization or compaction that allow the DNA molecule to be folded into the small space of the nucleus. 2) A DNA molecule in just one human chromosome is about 6000 times longer than the width of an average human cell. This makes compaction important. A double-stranded helix is 2nm in width, but once folded around the histones, it forms a fibre that is 10 nm wide. 1) Further coiling creates a 30 nm chromatin fiber, the structure commonly found in a transcriptionally active cell. 2) In preparation for cell division, additional coiling at three distinct levels creates the metaphase chromosome seen at the top. This compacted chromosome can more easily be moved during the process of cell division. Differences between cells 1) The size of the eukaryotic chromosome is much greater than the size of the bacterial nucleoid. 2) In addition, the eukaryotic genome is much greater in size relative to the prokaryotic genome. a) There are almost 5 million base pairs in the E. coli genome and about 4 290 protein coding genes. b) This compares to 6 billion base pairs in the human genome and an estimated 20 000 protein-coding genes. DESPITE these differences, we see the same nucleotides, same double helix, and similar genes coding for proteins such as those of the RNA polymerase and ribosomes. THEME 2 MODULE 1: Transcription Unit 1: DNA Blueprint Prokaryotes and Eukaryotes 1) DNA molecules found in both chromosomes are the same 2) Use adenine, thymine, cytosine, and guanine nucleotides to make up the DNA sequence 3) DNA = blueprint a) Contains thousands of genes that code for proteins or RNA that perform various functions 4) DNA = information storage a) Can be interpreted or transmitted 5) Genes are sections of the DNA molecule that are transcribed into RNA How are RNA made and what happens to RNA (RNA itself may be functional or it may be further interpreted into messenger rna) Genes code for specific proteins CENTRAL DOGMA (Francis Crick) 1) DNA acts like blueprint 2) Specific the sequence of bases in RNA 3) Specifies sequence of amino acids in a protein 4) DNA can be copied multiple times into messenger RNA or mRNA 5) mRNA can be translated from nucleotide language of the mRNA into amino acid language of the protein 6) DNA -> mRNA -> protein = process of gene expression and requires transcription of information in DNA into RNA Unit 2: Transcription in Prokaryotes 1) DNA is transcribed from a template strand 2) Genetic information that codes for RNA is contained within genes on a chromosomes a) DNA HAS DEOXYRIBOSE SUGAR (Adenine, cytosine, guanine, and thymine) b) RNA HAS RIBOSE SUGAR, NITROGENOUS BASE URACIL THAT PAIRS WITH ADENINE RATHER THAN THYMINE (Adenine, cytosine, guanine, and uracil) c) RNA molecules are usually a single strand unlike double stranded DNA 3) During transcription a) 1) DNA IS used as a template strange to generate a complementary and antiparallel RNA (ONLY one DNA strand for each gene will be the transcribed template strand) b) The nucleotide sequence that is contained within the gene will determine the sequence of nucleotides within the RNA (Same pattern as non-template strand except URACIL REPLACES thymine) For RNA to be transcribed from DNA (so these are the steps that occur above with the pink strand) 1) RNA polymerase (an enzyme) will attach to specific promoter regions in the DNA 2) The promoter regions are the transcription starting point where RNA synthesis begins and situation upstream (in 5’ direction) relative to the gene of interest 3) DNA and RNA have directionality (one end is the 5’ (5’ phosphate on nucleotide), and the other is named 3’ for (3’OH group of the nucleotide)) 4) Everything is read in a 5’ to 3’ direction 5) RNA polymerase will recognize and bind to a promoter a) The RNA polymerase begins transcribing along the template strand from (3’ to 5’ direction). As a result, once RNA polymerase binds the promoter regions, the RNA polymerase will begin transcribed in a 3’ to 5’ (template strand) and synthesize RNA from 5’ to 3’ end. RNA polymerase will move along the template stand in a (3’ to 5’) direction until a nucleotide sequence on the template strand called the terminator stops the transcript and releases the RNA transcript b) Once transcript in released the transcriptional machinery that includes RNA polymerase and associated proteins is disassembled How did RNA start transcribing the DNA? Prokaryotic DNA 1) Not packed into chromosomes 2) Sits within non-membrane nucleoid region because the prokaryote genome is smaller and has fewer genes than eukaryotes 3) Prokaryotic gene expression has served as model to understand how gene expression is turned on and off 4) A consensus nucleotide sequence of TATAAT is position about 10 base pairs upstream or 5’ of the start site of transcription and serves as the promoter region (found by comparing many different promoters and finding common elements and nucleotides - nucleotide sequence in the DNA found at this site) 5) More consensus sequences exist, such as the TTGCCA sequence (35 nucleotides upstream) of the transcription start site. These additional sequences can enhance the rate of transcription a) All prokaryotes use single type of RNA polymerase b) While the core RNA polymerase complex is needed for transcription, the actual process of transaction requires the association of sigma (proteins) factors which facilitate the bind to the promoter region of the DNA i) To initiate transcription prokaryotic RNA polymerase recruits different sigma factors to initiate transcription of different types of genes ii) When RNA polymerase core enzyme binds to sigma subunit to create a holoenzyme that is capable of binding to and unbind the double stranded DNA helix is what allows this transcription to occur iii) Different sigma factors identify specific promoters to RNA polymerase (turn genes off and on when need be) 6) Actual transcription occurs within the RNA POLYMERASE ENZYME a) RNA polymerase binds to specific promoter regions of DNA, opens double-stranded DNA, reveals template and nontemplate strand within a transcription bubble b) RNA polymerase begins to catalyze the production of an antiparallel RNA strand that is complementary to the template strand creating an RNA-DNA duplex c) Template strand is READ in 3’ to 5’ but is synthesized in 5’ to 3’ When the RNA polymerase binds to the template strand 1) Engages an “open confirmation” and functions as a molecular machine 2) Transcription continues along the length of the gene and RNA polymerase can elongate the forming RNA transcript one nucleotide at a time 3) Structural features of RNA polymerase allow it to separate the two strands of the DNA double helix (CREATING THE TRANSCRIPTION BUBBLE) 4) Ribonucleotides are able to enter the RNA polymerase and assemble in a complementary fashion on the DNA template strand 5) RNA polymerase continues to pass the template DNA through a channel, transcribe the template into the complementary RNA and thread the same template DNA out through the other channel 6) Structural features allow DNA double helix to be disrupted and RNA-DNA duplex to be stabilized, the RNA polymerase can restore DNA double helix once the transcript is produced Transcription continues 7) RNA polymerase continues going downstream from the promoter site, while unwinding the DNA helix and creating RNA polynucleotide transcript 8) RNA molecule is synthesized and dissociates with the DNA template, allowing the DNA helix to reform 9) A single gene is able to be transcribed by several RNA polymerase molecules at a time, much like a series of trucks that are following each other in an convoy along the DNA template, allow for multiple RNA copies of a single gene in greater amounts Transcription continues: 1) The RNA polymerase will continue to add ribonucleotides to the 3’ end of the elongating RNA transcript 2) Each incoming ribonucleotide triphosphate will correctly base pair with the template DNA 3) The phosphate bond energy of the incoming ribonucleotide triphosphate is used to drive the high energy process to create a phosphodiester bond between the incoming nucleotide and growing RNA transcript 4) The cleavage of the pyrophosphate (phosphate-phosphate) group during the phosphodiester bond formation renders this polymerization reaction of RNA transcript elongation irreversible DNA molecules have hundred to thousands of different genes when any one particular gene is being expressed or transcribed, it is important to terminate the process when the end of the gene sequence is reached - This occurs through termination sequences in the DNA at the 3’ end of a gene - Transcription stops at nucleotide terminator sequences that lie near the end of the coding sequence of a gene, and they enable the release of the RNA transcript and the subsequently the transcription complex Prokaryotes have two types of terminator sequences 1) Rho-independent terminator sequences consist of inverted nucleotide repeat sequences a) Inverted repeats are sequences of nucleotides that are usually followed downstream by a specific reverse complement b) At the end of transcription these rho-independent terminator sequences are transcribed and fold back on themselves to form what is referred to as G-C rich hairpin loop along the same mRNA strand c) THIS pauses the RNA polymerase and leads to the release of the mRNA transcript 2) Rho-dependent terminator sequences use a specific prokaryotic protein or Rho factor which can bind to and subsequently utilize ATP energy to move along the formed RNA transcript while unwinding from the DNA template a) Rho factor is able to destabilize the interaction between RNA and the DNA template, leading to the release of the transcript and transcription complex Slide 18: Transcription and translation are coupled in prokaryotes 1) The process of translating mRNA into a polypeptide can begin even before transcription is complete 2) Ribosome complexes can latch onto mRNA as it is being transcribed a) These two processes can be temporally and spatially coordinated due to the fact the prokaryotic cells lack compartmentalization b) Prokaryotes have no nuclear membrane that separates the process of transcription and translation c) Prokaryotic genes are organized somewhat differently than eukaryotes, leading to characteristic differences between the mRNA transcripts that produced by prokaryotes at the end of transcription compared to eukaryotes Unit 3: Transcription and mRNA processing in Eukaryotes Transcription in eukaryotes uses the same principles as in prokaryotes 1) As in prokaryotes, eukaryotes also have specific promoter regions of the DNA sequence which indicate transcription start points, but these promoters have a greater degree of complexity 2) RNA polymerase in bacteria only requires a sigma factor to recognize and bind to a promoter region, in eukaryotes a number of specific proteins called general transcription factors are required to mediate the binding of RNA polymerase to a promoter and to initiate transcription 3) Eukaryotes have the presence of three different types of RNA polymerase a) RNA polymerase I, II, and III i) RNA polymerase I and III transcribe structural, non-coding RNAs. ii) RNA I transcribes for genes for ribosomal RNAs (rRNAs), RNA III transcribes the genes for transfer RNAs (tRNAs) as well as other small regulatory RNA molecules iii) RNA II transcribes for messenger RNAs which serve as the templates for for production 4) Similar to prokaryotes, there are core promoter consensus sequences required to set up a transcription initiation complex, specifically for the particular RNA polymerase and gene being transcribed, BUT IN eukaryotic cells the termination of transcription occurs by many different process depending on the exact polymerase utilized In eukaryotes 1) Transcription and translation are physically separated in the cell by the nuclear membrane 2) During transcription RNA polymerase reads a sequence of DNA and is able to produce a complementary antiparallel strand of RNA, upon the termination, the RNA is released from the RNA polymerase a) This is true for other noncoding RNA (tRNA and rRNA) but it is the messenger RNA that is focused on for this course 3) ONCE RNA has been been modified it can exit the nucleus Post-Transcriptional Modifications (AFTER RNA IS TRANSCRIBED) 1) Modifications occur at each end of the primary mRNA transcript a) Modifications include the addition of a 5’ cap and a 3’ poly (A) tail b) THESE ensure the stability of the mRNA molecule i) Ensure the export of the mRNA from the nucleus ii) Protect against ribonuclear enzymes that target phosphodiester bonds iii) Help with attachment of the ribosomes and initiation of translation once mRNA reaches the cytoplasm of the cell Transcription beginning 1) The 5’ end of the primary RNA transcript is synthesized first, capping of the 5’ end of an mRNA transcript involves the attached of a modified guanine to the mRNA through an unusual 5’ to 5’ triphosphate linkage a) The modified guanine is referred to as 7-methylguanosine since it also has a methyl group (-CH3) that is attached to the 7th position in the guanine b) TO BE able to attach a 7–methylguanosine, the terminal 5’ phosphate is removed from the mRNA molecule by a phosphatase enzyme and another enzyme, guanosyl transferase enzyme catalyzes the attachment of the 7-methylguanosine 5’ cap Almost all transcribed mRNA molecules have a number of adenine nucleotides added to the 3’ end 1) This is the poly(A) tail 2) These additional adenine nucleotides are added to the 3’ end of the mRNA transcript following recognition of a polyadenylation signal sequence (AATAAA) that is transcribed from the DNA template strand near the gene sequence 3) Once the signal is transcribed the mRNA is cleaved, and a poly (A) polymerase enzyme is able to add between 150-200 adenine nucleotide bases to the 3’ end of the RNA transcript (polyadenylation) 4) Polyadenylation is coordinated with the termination of transcription Termination of transcription in eukaryotes depends on the type of RNA polymerase (I, II, or III) that is used proceeding transcription of the specific DNA sequence 1) Each of the 3 RNA polymerases in eukaryotes have a different type of termination system 2) RNA polymerase II depends on a poly(A)-dependent mechanism of termination a) As a result, termination of transcription coupled with mRNA maturation where the 3’ end of the transcript is modified by polyadenylation and modification of the 3’ mRNA transcript is coupled with the termination of transcription 3) Genes transcribed by RNA polymerase I, transcription is terminated using a specific eukaryotic termination factor in a similar manner as prokaryotic rho-dependent termination and 4) Genes that are transcribed by RNA polymerase III have transcription terminated after transcription of a termination sequence in a mechanism that resembles rho-independent termination in prokaryotes On top of post transcriptional modifications of 5’ and 3’ ends of the mRNA, mRNA is further modified by the removal of large parts of the transcribed RNA molecule 1) In the eukaryotic cell, the protein-coding DNA sequence is interrupted by long noncoding sequences of nucleotides that are not part of the message that will be translated into amino acids that make up a protein 2) These sequences are a stable and important part of the regulation of gene expression but the will not code any specific amino acids a) This means the DNA sequence which codes for a eukaryotic protein is split into many segments b) These sequences necessary for coding the sequence of amino acids in the protein are called exons c) The intervening sequences are introns. In transcribing a gene from DNA to RNA, a RNA polymerase will transcribe both the introns and the exons from the DNA, but the introns need to be removed or esxised from the mRNA and the exons need to be joined or spliced prior to transcription through a process called called RNA splicing d) OTHERWISE THE PROTEIN MAY NOT CONTAIN THE CORRECT SEQUENCE OF AMINO ACIDS RNA splicing occurs at specific short nucleotide sequences that are situation at each end of an intron 1) The process of RNA splicing is catalyzed by large molecular machines called spliceosomes a) Each spliceosome is composed of five small ribonucleoproteins (snRNPs) that are made up of small nuclear RNAs and proteins that are able to recognize the splice sites within the intron Splicing process 1) To start RNA splicing, the RNA in the spliceosome is able to recognize and form complementary base pairing with nucleotide at the designated splice sites 2) The spliceosome then catalyzes a reaction that allows a specific hydroxyl group on a nucleotide in the branch site to effectively attack and form a new phosphodiester bond with a nucleotide at the donor site 3) This allows for the cut 5’ end of the intro to form a lariat intermediate which forms a loop within the intron, then the 3’ hydroxyl group at the cut end of the 5’ exon at the donor site is able to attack the phosphodiester bond at the 3’ acceptor site forming a new phosphodiester bond between the 3’ end of the upstream exon and the 5’ end of a downstream exon 4) This will allow for the release of the intron and the splicing of the exons together in much like a cut and paste mechanisms 5) The excised intron lariat is subsequently broken down into individual nucleotides that can be recycled by the cell Unit 4: Export of the mRNA out of the nucleus 1) Genetic information of eukaryotic organisms is within the nucleus allowing for the separation of transcription and translation in eukaryotes both in space and time a) A CONTRAST TO PROKARYOTES where the lack of compartmentalization allows for translation to begin while transcription is in progress b) In eukaryotes, transcription of DNA into mRNA is followed by the export of mRNA from the nuclear compartment to the cytoplasm of the cell which is facilitate by nuclear pore complexes in the nuclear membrane that act as gateways for molecules to move into and out of the nucleus c) The nuclear pores are protein-lined channels that pass through both membranes of the nuclear envelope both the outer and inner membrane. d) Transport of RNA from the nucleus to the cytoplasm, nuclear pores can transport proteins, carbohydrates, and important signaling molecules into the nucleus Transcription of a protein-coding gene into a primary transcript occurs in the nucleus of eukaryotic cells - The RNA transcript must be modified to ensure only mature mRNA is exported from the nucleus to ensure that the message from the DNA blueprint is reliably transmitted to code for the appropriate amino acids that contribute to the structure and functionality of cellular proteins The end of transcription must lead to appropriate production of a specific mRNA template that will contribute to accurate polypeptide synthesis THEME 2 MODULE 2: The Genetic Code Transcription is an extremely valuable way of creating multiple copies of the gene and it can be further regulated by RNA stability or compartmentalization. 1) That said, we still have not made protein. 2) We have not taken the transcribed nucleotide code and translated it into another language - the language of proteins. Proteins are made from 1) Proteins are made from 20 different amino acid a) but there are only four nucleotides in mRNA: adenine (A), cytosine (C), guanine (G), and uracil (U) After the discovery of the structure of DNA, researchers wanted to read the hidden messages within DNA molecules 1) These first wanted to determine how many nucleotide bases code for a single amino acid A physicist named George Gamow suggested the number of nucleotides that would be necessary for one amino acid 1) RNA tie club (20 members - amino acids, 4 honorary people - one for each nucleotide) 2) If one nucleotide encoded for one amino acid, then DNA could only code for four distinct amino acid residues (much less than the required 20 amino acids that cells utilize, so ONE BASE WASN’T enough) 1) 2 isn’t sufficient to code for 20 (4 x 4 = 16) 3 nucleotides (a triplet) - 4 x 4 x 4 = 64 This is more than we need because there are only 20 distinct codes, however, the 3-base code is the smallest group of nucleotides that accommodate the need to code for 20 amino acids. - Some code for the same amino acids - The code could also have 4-5 nucleotides but that increases redundancy To figure out how mRNA turned into functional proteins, researchers took simple mRNA sequences and saw what they coded for 1) Marshall Nirenberg and Johann Mattahei used used a cell free system to decipher the first letter of the code in 1961 a) They put all the components they believed were necessary for protein synthesis into tubes (RNA template, nucleotides, ribosomes, amino acids, and an energy source) b) They were able to determine what specific amino acid a particular RNA nucleotide template gave rise to c) They found the simple nucleic acid produced a repeated simple polypeptide sequence that contained identical amino acids i) Repeated sequence of phenylalanine (how many uracils coded for this?) With nucleic acids with uracils and cytosines in a follow-up study 1) The strand of nucleotides was translated into a polypeptide string of alternating serine and leucine Confirmed the existence of codons (3 nucleotides) UCA codes for serine, CAU codes for histidine, AUC codes for isoleucine. Codons are always written in a 5’ to 3’ direction 1) The table represents codons that can be encoded by the mRNA transcript based on 4 possible nucleotide bases in mRNA 2) You can still determine, by replacing uracils with thymine, the DNA nucleotides that were the sequence on the non-template strand or the coding 3) The non-template strand is often referred to as the coding strand because the rNA transcript had the same base-sequence as the non-template strand (coding= non-template) 4) Non-coding or template contains complementary anticodons AUG = methionine (first triplet in every protein coding sequence, it is the START CODON that signals the region where protein synthesis should begin) Tryptophan is only coded for by UGG 61 out of 64 possible nucleotide triplets are able to code for the 20 amino acids of the cell. Redundancy occurs because there are more unique triplets than there are unique amino acids. 1) One example is seen here where four distinct triplets code for the same amino acid, serine. a) Though the genetic code is redundant, it is also unambiguous. i) A unique codon triplet will always code for a specific amino acid and will never code for more than one amino acid. This is essential as the cell has to produce the correct proteins with the appropriate sequence, structure, and functionality. The unambiguous nature of the genetic code ensures that there will never be any confusion as to which amino acid is placed at each position on a growing polypeptide chain. STOP codons don’t code for any amino acid at all (signal that the translation of mRNA into a polypeptide sequence is complete, stopping the translation process) - UAA, UGA, UAG Transcription 1) Reading genetic information from DNA template 2) Synthesizing complementary or antiparallel RNA molecule a) RNA polymerase copies the DNA template strand, the sequence of the template strand is often termed non-coding. This means if you read the sequence of the DNA non-template strand, except for the substitution of U for T, it is the same as the mRNA and so is sometimes called the coding strand. The coding region of a gene = open reading frame 1) To look for genes you try to find start codons and stop codons 2) The reading frame is a reflection of how the nucleotide sequence is read 3) 3 ways to read it Once the template strand is turned into mRNA, relation or reading of the mRNA can occur from the first, second or third nucleotides, respectively, the first, second, and third reading frames. A reading frame effects the amino acids that make up the sequence of a polyp edited and the protein that is produced 1) If nothing is known about the genes on a particular DNA molecule, you have 6 readings frames in total, three in one direction, three in the reverse Francis Crick, Leslie Barnett, Sydney Brenner, and Richard Watts-Tobin provided conclusive evidence that the code was written out in triplets of nucleotides. 1) If a single nucleotide was added within the nucleotide sequence, the code was misread. 2) While transcription and translation will still occur, these nucleotide triplets now code for different amino acids in the polypeptide. 3) A similar effect would happen if 1 nucleotide were removed. 4) The researchers observed the same effect when 2 nucleotides were added or removed. 5) These types of changes are referred to as frameshift mutations. 6) Interestingly, in the example shown here, if a compensating nucleotide were removed later in the code, the original reading frame would be restored but the resulting protein would have a section of wrong amino acids in the middle of it, although the effect would be minimized. When three nucleotides are removed as shown here, there is no frameshift, that is no change in reading frame. Instead, a single triplet codon has been removed and there is a corresponding removal of a single amino acid in the polypeptide. 1) All of the codon sequences after the removal of the single codon are conserved, maintaining the identity of most of the protein. 2) Similarly, adding a codon will simply add one amino acid to the protein, but the reading frame of the mRNA and most of the remainder of the polypeptide sequence are conserved. While DNA is the blueprint 1) RNA turns information into components that make up a cell 2) Transfer RNAs act as the intermediate between mRNA and the polypeptide (translators) between the two codes 3) RNA serve as catalytic units (nuclear RNAs of the spliceosome) So, while the origin of RNA remains largely unclear, it is a subject of much discussion and debate. The RNA world hypothesis suggests that some type of ancestral RNA molecule was the precursor to current life. This is largely supported by the fact that RNA molecules play such important roles in converting our genetic information coded in DNA into functional and necessary proteins. Review Lecture Theme 1, (1-2) Module 1: The composition of Cell Membranes SLIDE CONTENT NUMBE R: 3 Inner membranes of the mitochondria help produce energy 1) Compartmentalize tasks for inner membranes 2) Outer membrane of the cell allows for selectivity in what comes in and goes out of the cell Hydrophilic 1) Hydrophilic molecules 2) Polar Saturated 1) No kinks (single bonds) Unsaturated 1) Has kinks (double bonds) These change the properties of the membrane, the shape, size, behavior, of the phospholipids and ultimalet the membranes 5 1) Signal 2) Areas that are more fluid have more unsaturated tails a) Allows more space to be between phospholipids b) Saturated phospholipids line up to make a lipid raft (hydrophobic attraction is really high) c) Lipid rafts form and uniform depending on environmental cues 3) The presence or absence of cholesterol a) Linear in its shape, very hydrophobic, tucks itself into the linear saturated tails, making less movement in the tails 4) Areas that are less fluid also have longer tails Temperature , kinetic energy a) Cholesterol 2) Higher temps increase fluidity 3) Room Temperature a) Cholesterol Decreases fluidity 4) Lower temps decrease fluidity a) Cholesterol can insert itself between the tails to ensure that the membrane has more fluidity (hibernating animals, fish don’t get out in the winter, in these fish cholesterol is inserted into membranes and acts as a spacer) To Measure Fluidity FRAP (Fluorescence recovery after photobleaching) Label proteins fluorescently (Bleach them, take away fluorescence) - Is there any recovery? Is there lateral movement? 1) Membrane proteins moving around laterally 2) Label it with a fluorescent tag called GFP 3) There is a bleached area caused by the laser 4) The bleached areas move around, and the tagged areas go into those sections Endoplasmic reticulum membrane also moves 1) Overtime, the area fills in (the bleached area) 2) Bleach it 3) Recovery still occurs The Nucleus Membrane 1) Bleach it 2) Does not recover 3) Rigidity is needed to get out genetic information out reliably (those pores are kept roughly in the same space) 7 Fluidity (proteins, phospholipids moving within the membrane laterally) is different from permeability (membranes ability to move across the cell membrane or not) - Breathing in, lungs have really fast passive diffusion Small, uncharged polar molecules can trickle through Most water requires porins Some proteins help with 1) Signaling 2) These proteins are amphipathic (both hydrophobic and hydrophilic) Facilitated Movement: Downtown toronto subway Active Movement: have ushers helping people pack into a train, they even push people into the train against the concentration gradient Sodium Potassium Pump 1) Potassium is higher in the cell, so active transport has to work against the gradient Module 2: Organelles & Energy SLIDE CONTENT NUMBE R: 15 Chloroplasts manufacturer sugar Photosynthesis: a process used in plant cells and other organisms to convert light energy into chemical energy Chemical energy is stored in the bonds of carbohydrate molecules Cellular Respiration: a process used by plant and animal cells to release the chemical energy stored in the bonds of carbohydrate molecules and partially capture it in the form of ATP ATP Synthase can run in reverse, move protons back into intermembrane space Enough sugars for 50 minutes of energy, but then our body starts breaking down fat, then protein First Applied Lecture (No Modules) SLIDE CONTENT NUMB ER: 5 1) All cells contain important internal structures 2) Cells have unique properties which are all determined by the genetic blueprint or DNA code for the cell Plant Cells Animal Cells Microscopy enables us to understand how cells function 1) Electron microscopy: Put into a big column where there are blasting electrons, very high magnification a) We can see sperm trying to get to the egg b) Speed of the electrons, go through and bounce off, that determines the structure 2) Fluorescence microscopy: Can see the structure of cells and tag down proteins within the cell a) Each color is a different protein b) Antibody chases down the protein c) Melanocyte - Contains melanin (gives skin color) - Can see cell color i) You can see the actin proteins ii) You can label proteins all the time SLIDE CONTENT NUMB ER: 7 Cells in action as recorded using brightfield microscopy 1) Running a cell race (for “bragging” rights) 2) Try to get all the cells in the world together to see how they are different 3) A flat plate 2D, a 3D environment, and a 1D environment Had a catalog of cells - Cancer cell that moved microns per second won (8-9) All of our genetic information was in the single fertilized embryo (1 fertilized cell -> 10 trillion cells) 1) All cells have the same DNA 2) But what genes are turned on or off, that's what makes cells different 3) The DNA blueprint is the same We play host to perhaps 10X as many bacterial cells as eukaryotic cells: 100 trillion We have good microbes that help digest our food, microbes that protect our skin. Microbiome: a population of microorganisms or microbes (small organisms you can’t see with your eye) - 100 trillion bacterial cells had to come from somewhere - 10000 species of microbes that healthy individuals may host 12 Babies delivered by C-section had different gut microbes than those delivered vaginally - Microbe population can change continuously, our microbiome is inherently dynamic - Always changing, touching grass etc. 14, 15) Dirtiest things