Cell Membrane Structure and Function PDF
Document Details
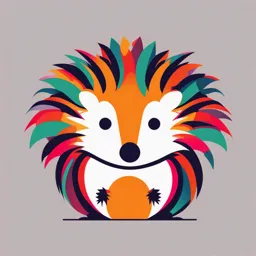
Uploaded by MightyHappiness8588
Yarmouk University
Nicole Tunbridge and Kathleen Fitzpatrick
Tags
Summary
This presentation covers the structure and function of cell membranes. It details the fluid mosaic model, the roles of phospholipids, proteins, and cholesterol, and how they affect membrane fluidity. It also briefly touches on the importance of cell-surface proteins in biology and medicine.
Full Transcript
Chapter 7 Membrane Structure and Function Lecture Presentations by Nicole Tunbridge and © 2017 Pearson Education, I...
Chapter 7 Membrane Structure and Function Lecture Presentations by Nicole Tunbridge and © 2017 Pearson Education, Inc. Kathleen Fitzpatrick Life at the Edge The plasma membrane is the boundary that separates the living cell from its surroundings The plasma membrane exhibits selective permeability, allowing some substances to cross it more easily than others Transport proteins are often responsible for controlling passage across cellular membranes © 2017 Pearson Education, Inc. Figure 7.1 © 2017 Pearson Education, Inc. Figure 7.1a Potassium ion Potassium ion channel protein © 2017 Pearson Education, Inc. Video: Structure of the Cell Membrane © 2017 Pearson Education, Inc. Concept 7.1: Cellular membranes are fluid mosaics of lipids and proteins Phospholipids are the most abundant lipid in the plasma membrane Phospholipids are amphipathic molecules, containing hydrophobic (“water-fearing”) and hydrophilic (“water-loving”) regions The hydrophobic tails of the phospholipids are sheltered inside the membrane, while the hydrophilic heads are exposed to water on either side © 2017 Pearson Education, Inc. Figure 7.2 Two phospholipids WATER Hydrophilic head Hydrophobic tail WATER © 2017 Pearson Education, Inc. In the fluid mosaic model, the membrane is a mosaic of protein molecules bobbing in a fluid bilayer of phospholipids Proteins are not randomly distributed in the membrane © 2017 Pearson Education, Inc. Figure 7.3 Fibers of extra- cellular matrix (ECM) Carbo- Glyco- hydrates protein Glycolipid EXTRACELLULAR SIDE OF MEMBRANE Phospholipid Cholesterol Microfilaments of cytoskeleton Peripheral proteins Integral CYTOPLASMIC protein SIDE OF MEMBRANE © 2017 Pearson Education, Inc. Figure 7.3a Fibers of extra- cellular matrix (ECM) Carbo- hydrates Glyco- protein Phospholipid Cholesterol Microfilaments of cytoskeleton © 2017 Pearson Education, Inc. Figure 7.3b Glycolipid EXTRACELLULAR SIDE OF MEMBRANE Peripheral proteins Integral protein CYTOPLASMIC SIDE OF MEMBRANE © 2017 Pearson Education, Inc. The Fluidity of Membranes Membranes are held together mainly by weak hydrophobic interactions Most of the lipids and some proteins can move sideways within the membrane Rarely, a lipid may flip-flop across the membrane, from one phospholipid layer to the other © 2017 Pearson Education, Inc. Figure 7.4_1 Results Membrane proteins Mouse cell Human cell Data from L. D. Frye and M. Edidin, The rapid intermixing of cell surface antigens after formation of mouse-human heterokaryons, Journal of Cell Science 7:319 (1970). © 2017 Pearson Education, Inc. Figure 7.4_2 Results Membrane proteins Mouse cell Human cell Hybrid cell Data from L. D. Frye and M. Edidin, The rapid intermixing of cell surface antigens after formation of mouse-human heterokaryons, Journal of Cell Science 7:319 (1970). © 2017 Pearson Education, Inc. Figure 7.4_3 Results Membrane proteins Mixed proteins Mouse cell after 1 hour Human cell Hybrid cell Data from L. D. Frye and M. Edidin, The rapid intermixing of cell surface antigens after formation of mouse-human heterokaryons, Journal of Cell Science 7:319 (1970). © 2017 Pearson Education, Inc. As temperatures cool, membranes switch from a fluid state to a solid state The temperature at which a membrane solidifies depends on the types of lipids Membranes rich in unsaturated fatty acids are more fluid than those rich in saturated fatty acids Membranes must be fluid to work properly; membranes are usually about as fluid as salad oil © 2017 Pearson Education, Inc. The steroid cholesterol has different effects on the membrane fluidity of animal cells at different temperatures At warm temperatures (such as 37ºC), cholesterol restrains movement of phospholipids At cool temperatures, it maintains fluidity by preventing tight packing Though cholesterol is present in plants, they use related steroid lipids to buffer membrane fluidity © 2017 Pearson Education, Inc. Figure 7.5 (a) Unsaturated versus saturated hydrocarbon tails Fluid Viscous Unsaturated tails Saturated tails pack prevent packing. together. (b) Cholesterol within the animal cell membrane Cholesterol reduces membrane fluidity at moderate temperatures, but at low temperatures hinders solidification. Cholesterol © 2017 Pearson Education, Inc. Evolution of Differences in Membrane Lipid Composition Variations in lipid composition of cell membranes of many species appear to be adaptations to specific environmental conditions Ability to change the lipid compositions in response to temperature changes has evolved in organisms that live where temperatures vary © 2017 Pearson Education, Inc. Membrane Proteins and Their Functions Somewhat like a tile mosaic, a membrane is a collage of different proteins, often clustered in groups, embedded in the fluid matrix of the lipid bilayer. Phospholipids form the main fabric of the membrane Proteins determine most of the membrane’s functions © 2017 Pearson Education, Inc. Figure 7.UN01 © 2017 Pearson Education, Inc. Peripheral proteins are bound to the surface of the membrane Integral proteins penetrate the hydrophobic core Integral proteins that span the membrane are called transmembrane proteins The hydrophobic regions of an integral protein consist of one or more stretches of nonpolar amino acids, often coiled into α helices © 2017 Pearson Education, Inc. Figure 7.6 N-terminus EXTRACELLULAR SIDE α helix C-terminus CYTOPLASMIC SIDE © 2017 Pearson Education, Inc. Cell-surface membranes can carry out several functions: Transport Enzymatic activity Signal transduction Cell-cell recognition Intercellular joining Attachment to the cytoskeleton and extracellular matrix (ECM) © 2017 Pearson Education, Inc. Figure 7.7 Signaling molecule Receptor Enzymes ATP Signal transduction (a) Transport (b) Enzymatic (c) Signal activity transduction Glyco- protein (d) Cell-cell (e) Intercellular (f) Attachment to the recognition joining cytoskeleton and extracellular matrix (ECM) © 2017 Pearson Education, Inc. Figure 7.7a Signaling molecule Receptor Enzymes ATP Signal transduction (a) Transport (b) Enzymatic (c) Signal activity transduction © 2017 Pearson Education, Inc. Figure 7.7b Glyco- protein (d) Cell-cell (e) Intercellular (f) Attachment to the recognition joining cytoskeleton and extracellular matrix (ECM) © 2017 Pearson Education, Inc. Cell-surface proteins are important in the medical field For example, HIV must bind to the immune cell- surface protein CD4 and a “co-receptor” CCR5 in order to infect a cell HIV cannot enter the cells of resistant individuals who lack CCR5 Drugs are now being developed to mask the CCR5 protein © 2017 Pearson Education, Inc. Figure 7.8 HIV Receptor Receptor (CD4) (CD4) but no CCR5 Co-receptor Plasma (CCR5) membrane (a) HIV can infect a cell with (b) HIV cannot infect a cell CCR5 on its surface, as in lacking CCR5 on its surface, most people. as in resistant individuals. © 2017 Pearson Education, Inc. The Role of Membrane Carbohydrates in Cell- Cell Recognition Cells recognize each other by binding to molecules, often containing carbohydrates, on the extracellular surface of the plasma membrane Membrane carbohydrates may be covalently bonded to lipids (forming glycolipids) or, more commonly, to proteins (forming glycoproteins) Carbohydrates on the extracellular side of the plasma membrane vary among species, individuals, and even cell types in an individual © 2017 Pearson Education, Inc. Synthesis and Sidedness of Membranes Membranes have distinct inside and outside faces The asymmetrical distribution of proteins, lipids, and associated carbohydrates in the plasma membrane is determined when the membrane is built by the ER and Golgi apparatus © 2017 Pearson Education, Inc. Figure 7.9 Lipid bilayer Transmembrane Secretory glycoproteins protein Golgi apparatus Vesicle Attached carbohydrate Glycolipid ER lumen Plasma membrane: Cytoplasmic face Transmembrane Extracellular face glycoprotein Secreted protein Membrane glycolipid © 2017 Pearson Education, Inc. Concept 7.2: Membrane structure results in selective permeability A cell must exchange materials with its surroundings, a process controlled by the plasma membrane Plasma membranes are selectively permeable, regulating the cell’s molecular traffic © 2017 Pearson Education, Inc. The Permeability of the Lipid Bilayer Hydrophobic (nonpolar) molecules, such as hydrocarbons, can dissolve in the lipid bilayer and pass through the membrane rapidly. Hydrophilic molecules including ions and polar molecules do not cross the membrane easily. Proteins built into the membrane play key roles in regulating transport. © 2017 Pearson Education, Inc. Transport Proteins Transport proteins allow passage of hydrophilic substances across the membrane. Some transport proteins, called channel proteins, have a hydrophilic channel that certain molecules or ions can use as a tunnel Channel proteins called aquaporins greatly facilitate the passage of water molecules © 2017 Pearson Education, Inc. Transport Proteins Other transport proteins, called carrier proteins, bind to molecules and change shape to shuttle them across the membrane. A transport protein is specific for the substance it moves © 2017 Pearson Education, Inc. Concept 7.3: Passive transport is diffusion of a substance across a membrane with no energy investment Diffusion is the tendency for molecules to spread out evenly into the available space. Although each molecule moves randomly, diffusion of a population of molecules may be directional. At dynamic equilibrium, as many molecules cross the membrane in one direction as in the other. © 2017 Pearson Education, Inc. Figure 7.10 Molecules of dye Membrane (cross section) WATER Net diffusion Net diffusion Equilibrium (a) Diffusion of one solute Net diffusion Net diffusion Equilibrium Net diffusion Net diffusion Equilibrium (b) Diffusion of two solutes © 2017 Pearson Education, Inc. Passive transport Substances diffuse down their concentration gradient, the region along which the density of a chemical substance increases or decreases. No work must be done to move substances down the concentration gradient. The diffusion of a substance across a biological membrane is passive transport because no energy is expended by the cell to make it happen © 2017 Pearson Education, Inc. Effects of Osmosis on Water Balance Osmosis is the diffusion of water across a selectively permeable membrane Water diffuses across a membrane from the region of lower solute concentration to the region of higher solute concentration until the solute concentration is equal on both sides © 2017 Pearson Education, Inc. Figure 7.11 Lower concentration Higher concentration More similar of solute (sugar) of solute concentrations of solute Sugar H2O molecule Selectively permeable membrane Osmosis © 2017 Pearson Education, Inc. Osmosis Selectively permeable membrane Water molecules can pass through Water molecules pores, but sugar cluster around molecules cannot. sugar molecules. This side has This side has more fewer solute solute molecules molecules and and fewer free more free water water molecules. molecules. Osmosis © 2017 Pearson Education, Inc. Water Balance of Cells Without Cell Walls Tonicity is the ability of a surrounding solution to cause a cell to gain or lose water The tonicity of a solution depends on its concentration of solutes that cannot cross the membrane relative to that inside the cell © 2017 Pearson Education, Inc. Water Balance of Cells and solutions Isotonic solution: Solute concentration is the same as that inside the cell; no net water movement across the plasma membrane Hypertonic solution: Solute concentration is greater than that inside the cell; cell loses water Hypotonic solution: Solute concentration is less than that inside the cell; cell gains water Cells without cell walls will shrivel in hypertonic solution and lyse (burst) in a hypotonic solution © 2017 Pearson Education, Inc. Figure 7.12 Hypotonic Isotonic Hypertonic (a) Animal cell H2O H2O H2O H2O Lysed Normal Shriveled Cell Plasma wall H2O Plasma H2O membrane H2O membrane H2O (b) Plant cell Turgid (normal) Flaccid Plasmolyzed © 2017 Pearson Education, Inc. Solutions and Osmoregulation Hypertonic or hypotonic environments create osmotic problems for organisms that have cells without rigid walls Osmoregulation, the control of solute concentrations and water balance, is a necessary adaptation for life in such environments For example, the unicellular eukaryote Paramecium, which is hypertonic to its pond water environment, has a contractile vacuole that acts as a pump © 2017 Pearson Education, Inc. Solutions and Osmoregulation Contractile vacuole 50 µm © 2017 Pearson Education, Inc. Osmoregulation Bacteria and archaea that live in hypersaline (excessively salty) environments have cellular mechanisms to balance internal and external solute concentrations © 2017 Pearson Education, Inc. Water Balance of Cells with Cell Walls Cell walls help maintain water balance A plant cell in a hypotonic solution swells until the wall opposes uptake; the cell is now turgid (firm) If a plant cell and its surroundings are isotonic, there is no net movement of water into the cell; the cell becomes flaccid (limp) © 2017 Pearson Education, Inc. Solutions and Osmoregulation In a hypertonic environment, plant cells lose water The membrane pulls away from the cell wall, causing the plant to wilt, a potentially lethal effect called plasmolysis © 2017 Pearson Education, Inc. Facilitated Diffusion: Passive Transport Aided by Proteins In facilitated diffusion, transport proteins speed the passive movement of molecules across the plasma membrane. Transport proteins include channel proteins and carrier proteins © 2017 Pearson Education, Inc. Facilitated Diffusion: Passive Transport Aided by Proteins Channel proteins provide corridors that allow a specific molecule or ion to cross the membrane Aquaporins facilitate the diffusion of water Ion channels facilitate the transport of ions Some ion channels, called gated channels, open or close in response to a stimulus For example, in nerve cells, ion channels open in response to electrical stimulus © 2017 Pearson Education, Inc. Figure 7.14 (a) A channel EXTRACELLULAR protein FLUID Channel protein Solute CYTOPLASM Carrier protein Solute (b) A carrier protein © 2017 Pearson Education, Inc. Carrier Proteins Carrier proteins undergo a subtle change in shape that translocates the solute-binding site across the membrane This change in shape can be triggered by the binding and release of the transported molecule © 2017 Pearson Education, Inc. Concept 7.4: Active transport uses energy to move solutes against their gradients Facilitated diffusion is still passive because the solute moves down its concentration gradient, and the transport requires no energy Some transport proteins, however, can move solutes against their concentration gradients © 2017 Pearson Education, Inc. The Need for Energy in Active Transport Active transport requires energy, usually in the form of ATP hydrolysis, to move substances against their concentration gradients. All proteins involved in active transport are carrier proteins. © 2017 Pearson Education, Inc. The Need for Energy in Active Transport Active transport allows cells to maintain concentration gradients that differ from their surroundings For example, an animal cell has a much higher potassium (K+) and a much lower sodium (Na+) concentration compared to its surroundings This is controlled by the sodium-potassium pump, a transport protein that is energized by transfer of a phosphate group from the hydrolysis of ATP © 2017 Pearson Education, Inc. Figure 7.15 EXTRACELLULAR [Na+] high FLUID [K+] low Na+ Na+ Na+ Na+ [Na+] low Na+ Na+ [K+] high CYTOPLASM P ATP 1 K + ADP K+ 2 6 Na+ Na+ Na+ K+ K+ K+ K+ P 3 P Pi 5 4 © 2017 Pearson Education, Inc. Figure 7.15a EXTRACELLULAR [Na+] high FLUID [K+] low Na+ Na + Na+ Na+ Na+ [Na+] low P ATP Na+ [K+] high CYTOPLASM ADP 1 Cytoplasmic Na+ binds 2 Na+ binding to the sodium-potassium stimulates phosphory- pump. The affinity for Na+ lation by ATP. is high when the protein has this shape. © 2017 Pearson Education, Inc. Figure 7.15b Na + Na+ K+ Na+ K+ P P P i 3 Phosphorylation leads 4 The new shape has a to a change in protein high affinity for K+, which shape, reducing its affinity binds on the extracellular for Na+, which is released side and triggers release outside. of the phosphate group. © 2017 Pearson Education, Inc. Figure 7.15c K+ K+ K+ K+ 5 Loss of the phosphate 6 K+ is released; group restores the affinity for Na+ is high protein’s original shape, again, and the cycle which has a lower affinity repeats. for K+. © 2017 Pearson Education, Inc. Figure 7.16 Passive transport Active transport Diffusion Facilitated diffusion ATP © 2017 Pearson Education, Inc. How Ion Pumps Maintain Membrane Potential Membrane potential is the voltage across a membrane Voltage is created by differences in the distribution of positive and negative ions across a membrane The cytoplasmic side of the membrane is negative in charge relative to the extracellular side © 2017 Pearson Education, Inc. How Ion Pumps Maintain Membrane Potential Two combined forces, collectively called the electrochemical gradient, drive the diffusion of ions across a membrane A chemical force (the ion’s concentration gradient) An electrical force (the effect of the membrane potential on the ion’s movement). © 2017 Pearson Education, Inc. How Ion Pumps Maintain Membrane Potential An electrogenic pump is a transport protein that generates voltage across a membrane The sodium-potassium pump is the major electrogenic pump of animal cells. The main electrogenic pump of plants, fungi, and bacteria is a proton pump, which actively transports hydrogen ions (H+) out of the cell Electrogenic pumps help store energy that can be used for cellular work © 2017 Pearson Education, Inc. How Ion Pumps Maintain Membrane Potential ATP – + EXTRACELLULAR FLUID + H+ H+ H+ H+ Proton pump + H+ CYTOPLASM – + H+ © 2017 Pearson Education, Inc. Cotransport: Coupled Transport by a Membrane Protein Cotransport occurs when active transport of a solute indirectly drives transport of other substances. The diffusion of an actively transported solute down its concentration gradient is coupled with the transport of a second substance against its own concentration gradient © 2017 Pearson Education, Inc. Figure 7.18 + – Sucrose Sucrose H+/sucrose cotransporter Diffusion of H+ + H+ H+ – H+ – + H+ H+ H+ H + Proton pump H+ – + H+ ATP © 2017 Pearson Education, Inc. Concept 7.5: Bulk transport across the plasma membrane occurs by exocytosis and endocytosis Small molecules and water enter or leave the cell through the lipid bilayer or via transport proteins. Large molecules, such as polysaccharides and proteins, cross the membrane in bulk via vesicles. Bulk transport requires energy. © 2017 Pearson Education, Inc. Exocytosis In exocytosis, transport vesicles migrate to the membrane, fuse with it, and release their contents outside the cell Many secretory cells use exocytosis to export their products © 2017 Pearson Education, Inc. Endocytosis In endocytosis, the cell takes in macromolecules by forming vesicles from the plasma membrane Endocytosis is a reversal of exocytosis, involving different proteins There are three types of endocytosis Phagocytosis (“cellular eating”) Pinocytosis (“cellular drinking”) Receptor-mediated endocytosis © 2017 Pearson Education, Inc. Figure 7.19 Receptor-Mediated Phagocytosis Pinocytosis Endocytosis EXTRACELLULAR FLUID Solutes Solutes Pseudopodium Receptor Plasma membrane Coat protein Food or Coated other pit particle Coated vesicle with specific solutes Coated (purple) bound to vesicle Food receptors (red) vacuole CYTOPLASM © 2017 Pearson Education, Inc. Endocytosis In phagocytosis, a cell engulfs a particle in a vacuole The vacuole fuses with a lysosome to digest the particle. In pinocytosis, molecules dissolved in droplets are taken up when extracellular fluid is “gulped” into tiny vesicles © 2017 Pearson Education, Inc. Figure 7.19a Phagocytosis EXTRACELLULAR FLUID Solutes Green algal cell Pseudopodium 5 µm Food Pseudopodium of amoeba or An amoeba engulfing a green algal other cell via phagocytosis (TEM) particle Food vacuole CYTOPLASM © 2017 Pearson Education, Inc. Figure 7.19aa Green algal cell 5 µm Pseudopodium of amoeba An amoeba engulfing a green algal cell via phagocytosis (TEM) © 2017 Pearson Education, Inc. Figure 7.19b Pinocytosis Solutes Plasma membrane 0.25 µm Coat protein Pinocytotic vesicles Coated forming (TEMs) pit Coated vesicle © 2017 Pearson Education, Inc. Receptor-mediated endocytosis In receptor-mediated endocytosis, binding of specific solutes to receptors triggers vesicle formation Receptor proteins, receptors, and other molecules from the extracellular fluid are transported in the vesicles Emptied receptors are recycled to the plasma membrane © 2017 Pearson Education, Inc. Figure 7.19c Receptor-Mediated Endocytosis Plasma Coat Receptor membrane protein Coated 0.25 µm vesicle with specific solutes (purple) bound to receptors (red) Top: A coated pit Bottom: A coated vesicle forming during receptor- mediated endocytosis (TEMs) © 2017 Pearson Education, Inc. Figure 7.19ca Plasma Coat membrane protein 0.25 µm Top: A coated pit Bottom: A coated vesicle forming during receptor- mediated endocytosis (TEMs) © 2017 Pearson Education, Inc. Receptor-mediated endocytosis Human cells use receptor-mediated endocytosis to take in cholesterol, which is carried in particles called low-density lipoproteins (LDLs) Individuals with the disease familial hypercholesterolemia have missing or defective LDL receptor proteins © 2017 Pearson Education, Inc. Figure 7.UN02a Glucose Uptake over Time in Guinea Pig Red Blood Cells 100 radioactive glucose (mM) 80 Concentration of 60 40 15-day-old guinea pig 20 1-month-old guinea pig 0 0 10 20 30 40 50 60 Incubation time (min) Data from T. Kondo and E. Beutler, Developmental changes in glucose trans- port of guinea pig erythrocytes, Journal of Clinical Investigation 65:1–4 (1980). © 2017 Pearson Education, Inc. Figure 7.UN03 LDL LDL receptor Normal Mild Severe cell disease disease © 2017 Pearson Education, Inc. Figure 7.UN04 Passive transport: Facilitated diffusion Channel Carrier protein protein © 2017 Pearson Education, Inc. Figure 7.UN05 Active transport ATP © 2017 Pearson Education, Inc. Figure 7.UN06 “Environment” ‘‘Cell” 0.01 M sucrose 0.03 M sucrose 0.01 M glucose 0.02 M glucose 0.01 M fructose © 2017 Pearson Education, Inc.