Cook Sensory Slides PDF
Document Details
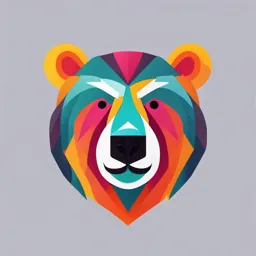
Uploaded by MindBlowingFibonacci
McGill University
Tags
Summary
This document covers various aspects of the nervous system, including its divisions, brain anatomy, spinal cord anatomy, cranial nerves, sensory inputs, and perceptions. It also details the development, circulation, and blood supply to the brain. Topics included discuss sensory modalities and receptors, and the blood-brain barrier.
Full Transcript
Major divisions of the nervous system Afferent (sensory input) Efferent (motor output) Cell bodies out of CNS Cell bodies in CNS Cranial Nerves Cranial Nerves Somatic, visual, olfactory, taste, auditory, vestibular Spinal Nerves...
Major divisions of the nervous system Afferent (sensory input) Efferent (motor output) Cell bodies out of CNS Cell bodies in CNS Cranial Nerves Cranial Nerves Somatic, visual, olfactory, taste, auditory, vestibular Spinal Nerves Somatic efferent Innervates skeletal muscle Spinal Nerves Only excitatory (ACh) Somatic sensation Motor neurons Touch Autonomic efferent Temperature Innervates interneurons Pain Smooth & cardiac muscle Excitatory & inhibitory Visceral Enteric Brain anatomy Cerebrum (cortex) Frontal Central sulcus Corpus callosum Parietal Thalamus Temporal Occipital Brainstem Cerebellum Gyrus Midbrain Pons Medulla Sulcus Spinal cord Brain anatomy: coronal slice Ventricles Gray matter White matter Thalamus Basal nuclei (ganglia) Limbic system Divisions of the spinal cord Cervical nerves (8 pair) Neck, shoulders, arms, and hands. Thoracic nerves (12 pairs) Shoulders, chest, upper abdominal wall. Lumbar nerves (5 pairs) Lower abdominal wall, hips, and legs. Sacral nerves (5 pairs) Genitals and lower digestive track. Coccygeal nerves (1 pair) Spinal cord anatomy Dorsal horn Gray matter Central Canal Ventral horn White matter Spinal segment { Dorsal root Ventral root Spinal nerve Dorsal root ganglion Twelve cranial nerves Olfactory nerve Cerebral cortex Optic nerve Cerebellum Brain edema Increased intercranial pressure pushes brain out the base of the skull. Compresses the brainstem and cranial nerves that regulate the pupillary response. Early development of the nervous system Blastocyst (week 1) Inner cell mass Ball of cells Blastocyst (week 2) Fertilized egg (Ovum) Embryonic disk Neural plate Blastocyst (week 3) Week 3 Development: the neural tube Ectoderm Neural plate Mesoderm Week 3 } Embryonic disk Endoderm Neural Groove Neural tube Neural crest become part of PNS Week 4 Becomes dura Neural tube becomes CNS and part of PNS The neural tube Vesicles develop during week 4 Forebrain Midbrain Hindbrain The neural tube becomes the CNS Cerebral hemispheres Forebrain Thalamus Midbrain Midbrain Pons Medulla Hindbrain Cerebellum Spinal cord Cavity becomes the ventricles and central canal Front Ventricles contain 150 ml of cerebral spinal fluid (CSF) Lateral ventricles Choroid plexus Third ventricle Fourth ventricle Cerebrospinal spinal fluid Formation: Produced by the choroid plexus (in the four ventricles, but mainly the two lateral) at a rate of 500 ml/day. Function: 1) Supports and cushions the CNS. Specific gravity of CSF and the brain are equal. 2) Provides nourishment to the brain. 3) Removes metabolic waste through absorption at the arachnoid villi. Composition: Sterile, colorless, acellular fluid that contains glucose. Circulation: Passive (not pumped). CSF circulation Third ventricle Foramen of Monro Cerebral aqueduct (midbrain) Fourth ventricle (between brainstem and cerebellum) Central canal CSF circulation Subarachnoid space Arachnoid villi CSF enters the subarachnoid space Foramens of Lushka (two lateral) & Magendie CSF circulation Hydrocephalus 1) Communicating 2) Noncommunicating 3 Meninges (membranes) of the CNS Skin Subarachnoid space Meninges cover the Bone brain and spinal cord Dura mater } Arachnoid membrane CSF Pia mater Gray matter (cerebral cortex) Trabeculae White matter Blood vessel Dural (venous) sinus Arachnoid villi CSF returns to the blood at the dural sinus Dura Arachnoid membrane CSF Pia Left Subarachnoid Right Gray matter space White matter Midline Blood supply to the brain Glucose is usually the only substrate metabolized by the brain. Very little glycogen in the brain. Brain needs a continuous supply of glucose and oxygen (glucose transport into the brain does not require insulin). A few seconds of blood supply interruption can lead to loss of consciousness. A few minutes can lead to neuronal death (stroke). Brain receives 15% of total blood (but is 2% of total mass) Blood supply to the brain External carotid artery Internal carotid artery (outside of the head) (base of the brain) Vertebral artery Common carotid artery Aorta From heart To body (85%) Blood supply: front view Circle of Willis Safety factor! Basilar artery Internal carotid Vertebral External carotid Common carotid Aorta From heart To body (85%) Cerebral circulation: CSF and blood DURAL SINUS Arachnoid villi Basilar Ventricles artery Blood-brain barrier (capillary wall) Foot processes of astrocytes Extracellular fluid of the brain Endothelial cells Water Tight junctions CO2 between endothelial cells O2 Capillary Lipid-soluble substances Active transport of Na+ K+ X Plasma proteins glucose and some amino acids Cl- Large organic molecules Blood-brain barrier: astrocytes (glia) Phagocytosis of debris Glutamate K+ Astrocytes Capillary Provide structural support Induce tight junctions Sensory modalities Perception of the external world Sensation: Awareness of sensory stimulation. Perception: The understanding of a sensation’s meaning. We do not perceive the “energy” of a sensory stimulus directly. We only perceive the neural activity that is produced by sensory stimulation. Perception of the external world Law of specific nerve energies: Regardless of how a sensory receptor is activated, the sensation felt corresponds to that of which the receptor is specialized. Example: Rub your eyes hard and you will see light. Law of projection: Regardless of where in the brain you stimulate a sensory pathway, the sensation is always felt at the sensory receptors location. Example: Penfield electrically stimulated somatic sensory cortex and patients perceived somatic sensation in the body. Example: Phantom limb pain after amputation. Sensory inputs are represented by a labeled-line code Modality: General class of a stimulus. Labeled-line: The brain “knows” the modality and location of every sensory afferent. See laws of specific nerve energies and projections. Sensory receptors 3) Transduction 5) Afferent 4) Ion channel activation 1) Stimulus energy 2) Receptor membrane Adequate stimulus (specificity) Receptor cell Stimulus energy is converted into afferent activity Stimulus energy Receptor Membrane depolarization potential (mV) Action Threshold to produce potentials action potentials Propagation of action potentials Release of neurotransmitter Stimulus intensity and afferent response Stimulus Subthreshold Weak Strong energy Magnitude of receptor potential Frequency of action potentials Magnitude of neurotransmitter release Adaptation of afferent response Majority of afferents show adaptation and this allows us to be sensitive to changes in sensory input. Stimulus Non-adapting Encodes stimulus intensity and slow changes Slowly adapting Afferent Some stimulus intensity and response moderate stimulus changes Rapidly adapting Fast stimulus changes On-response Off-response Time Receptive field (RF) The region in space that activates a sensory receptor or neuron. CNS Graded response across RF RF RF Afferent response (Number of Skin spikes) A B C A B C Stimulus location Receptive fields overlap Overlapping RFs produce a population code A B C Response to stimulus A B C Stimulus acuity and RF size Acuity: ability to Small RF differentiate one stimulus High acuity from another Back Large RF Low acuity Back Lips Response Lips Location Lateral inhibition sharpens Perception Without sensory acuity lateral With lateral inhibition inhibition Response to stimulus A’ B’ C’ 2nd order neurons - Inter- neurons + A’ B’ C’ A B C A B C Descending pathways modulate sensory inputs Neurons in Sensory information is the CNS shaped by both “bottom up” and “top down” mechanisms Presynaptic inhibition Sensory modalities Somatic (bodily) sensation is mediated by several types of receptors Glabrous skin (hairless) Touch: Mechanoreceptors with specialized end organs that surround the nerve terminal. These end organs allow only selective mechanical information to activate the nerve terminal. Superficial layers: Meissner’s corpuscle (A): Fluid-filled structure enclosing the nerve terminal. Rapidly adapting. Light stroking and fluttering. Merkel disk (B): Small epithelial cells surround the nerve terminal. Slowly adapting. Pressure and texture. Somatic (bodily) sensation is mediated by several types of receptors Glabrous skin (hairless) Touch: Mechanoreceptors with specialized end organs that surround the nerve terminal. These end organs allow only selective mechanical information to active the nerve terminal. Deep layers: Pacinian corpuscle (D): Large concentric capsules of connective tissue surround the nerve terminal. Rapidly adapting. Strong vibrations. Ruffini endings (E): Nerve endings wrap around a spindle-like structure. Slowly adapting. Stretch and bending of the skin (shape of an object). Somatic (bodily) sensation is mediated by several types of receptors Proprioception: Muscle spindles provide sense of static position and movement of limbs and body. We will discuss these receptors in the motor section. Mechanoreceptors are activated by stretching the cytoskeletal strands Mechanical deformation Extracellular Intracellular Cytoskeletal Ion channels strands Somatic (bodily) sensation is mediated by several types of receptors Glabrous skin (hairless) Temperature: Thermoreceptors are free nerve endings containing ion channels that respond to different temperature ranges. Cold afferents: 0 – 35ºC. (Activated by menthol) Warm afferents: 30 – 50ºC. (Activated by capsaicin and ethanol) Extreme temperatures activate pain receptors. Somatic (bodily) sensation is mediated by several types of receptors Glabrous skin (hairless) Pain: Nociceptors are free nerve endings containing ion channels that open in response to intense mechanical deformation, excessive temperature, or chemicals. Pain afferents are highly modulated (enhanced and suppressed). Visceral pain receptors: Activated by inflammation. Nociceptors are enhanced by many mediators 5. Enhancement of surrounding nociceptors by injured tissue & afferent feedback onto mast cells 1. 2. 3. Substance P released in spinal cord 4. 6. Dilation of nearby blood vessels Hyperalgesia (bottom up) Dorsal columns Somatosensory cortex Touch and proprioception Thalamus Medial lemniscus Medulla Ipsilateral Contralateral Dorsal columns Dorsal root ganglion Spinal nerve Somatosensory Anterolateral cortex pathway Thalamus Temperature and pain Branches into the reticular formation Dorsal horn Anterolateral column (spinothalamic) Dorsal root ganglion Spinal nerve Somatosensory Anterolateral cortex pathway Thalamus Temperature and pain Branches into the reticular formation Dorsal horn Anterolateral column (spinothalamic) Dorsal root ganglion Spinal nerve Somatosensory cortex Low Somatotopic map acuity High acuity Front Legs Motor cortex Arms Central Head sulcus Contralateral representation Somatosensory cortex Referred pain Visceral & somatic pain afferents commonly synapse on the same neurons in the spinal cord. Heart attacks commonly Perception of pain produce pain in the left arm. Activation of pain afferent Referred pain Descending pathways Somatosensory cortex regulate nociceptive information Periaqueductal gray matter (midbrain) Reticular formation (medulla) Dorsolateral funiculus Anterolateral column - (spinothalamic) Analgesia (top down) Reduction of pain through presynaptic inhibition Descending pathways from the brainstem Opiate neurotransmitters Pain stimulus (presynaptic inhibition) Pain afferent Substance P X released in spinal cord Morphine Sensory modalities Visual perception is dependent on context Anatomy of the eye Vitreous humor Retina Retinal pigment Lens epithelium Optic nerve Iris Sclera Pupil Cornea Blood vessels Fovea centralis (highest visual acuity) Optic disk (blind spot) A lens refracts (bends) light to a single point Light is refracted by the cornea and lens Cornea refracts light more than the lens Focus Accommodation for near vision Limited focal range Ciliary muscles control lens shape Lens accommodates for changes in object location Common optical defects of the eye The eye is myopic Is near work a contributing factor to myopia? Table 1. Hours Spent per Week in Various Activities Outside of School All Subjects Myopes (n = Activity (n = 366) 67) Studying 9.4 ± 5.7 11.2 ± 7.2* Reading for 4.4 ± 4.5 5.8 ± 4.8 ** pleasure Watching TV 8.3 ± 5.9 9.2 ± 6.8 Video games/ 2.3 ± 3.3 2.7 ± 4.1 computer Diopter-hours 53.8 ± 26.8 65. ± 34.1** Sports 9.3 ± 6.4 7.4 ± 6.7 Data are expressed as mean hours ± SD. * P < 0.05. , ** P < 0.005 Mutti DO, Mitchell GL, Moeschberger ML, Jones LA, Zadnik K. Invest Ophthalmol Vis Sci. 2002. Common optical defects of the eye Astigmatism: the lens or The eye is myopic cornea are not spherical Presbyopia: the lens gets stiff and is unable to accommodate for near vision The eye is hyperopic Cataract: changes in lens color Organization of the retina Retinal Pigment Epithelium Rods Transduction Cones Change in neurotransmitter Horizontal release Bipolar cells In fovea cells Processing and centralis, the Amacrine cells convergence retinal circuitry is shifted out of the way. Ganglion cells Becomes optic nerve Vitreous humor Light Phototransduction Outer segment Chromophore (retinene) cGMP GMP Inner 2. G-protein cascade + segment 1. Light activates opsin molecule X 3. cGMP to GMP 4. Channels close X Transmitter Light causes photoreceptors to hyperpolarize released in dark Four different opsin molecules (rhodopsin is found in the rods) Differences between rods and cones Rods Cones High sensitivity, night vision Low sensitivity, day vision More rhodopsin, captures more Less opsin light High amplification, single photon Lower amplification closes many Na+ channels Slow response time Faster response time More sensitivity to scattered light Most sensitive to direct axial rays Rod system Cone system Low acuity: not present in central High acuity: concentrated in fovea, fovea, highly convergent less convergent Achromatic: one type opsin Chromatic: three types of opsin Light and dark adaptation Dark adaptation Bright light Dark Rods are inactivated Temporary blindness until Cones are active rods “re-activate” and take over Light adaptation Dark Bright Light Cones are inactive Rods are initially saturated. Rods are active Temporary blindness until rods “inactivate” and cones take over Light and dark adaptation Light and dark adaptation It takes time to put the chromophore and opsin back together Opsin+chromophore Light causes Retinal Pigment Epithelium photoreceptors to hyperpolarize Chromophore Retina reports relative intensity of light Organization of the retina Retinal Pigment Epithelium Rods Transduction Cones Change in neurotransmitter Horizontal release Bipolar cells In fovea cells Processing and centralis, the Amacrine cells convergence retinal circuitry is shifted out of the way. Ganglion cells Becomes optic nerve Vitreous humor Light Retinal ganglion cells have center-surround receptive fields Inhibitory surround - - Excitatory ++ - + Excitatory surround + - Inhibitory - - center + + center Light on Light on Bright center – – + + dark surround –+– +– + – + Dark center – – + + –+– +– + bright surround – + Uniform – – + + –+– +– + light – + Retinal ganglion cells signal the relative differences of the light (contrast) across their receptive fields. Photoreceptors are sensitive to wavelength The opsin molecule determines the chromatic sensitivity of the photoreceptor. Perception of color is context dependent Retinal ganglion cells have color-opponent receptive fields Center surround RF Center surround RF with color The output of the retina encodes relative values of brightness and color. Color blindness Flow of visual information in the brain Right visual Left visual field field Optic nerve (one eye with both visual fields) Optic tract (both eyes with Optic chiasm contralateral visual field) Lateral geniculate nucleus (Thalamas) Optic radiations Visual cortex in occipital lobe (both eyes with contralateral visual field) The anatomy of visual field deficits Right Left Right Left 1. Loss of vision in the ipsilateral eye. 1. 2. Loss of vision in 3. contralateral visual field. 2. 3. Bilateral loss of temporal visual hemifields. X 4. Loss of vision in XXX 4. contralateral visual field. Cortical representation of the visual world Polymodal: visual and other sensory modalities are combined. Parietal visual stream (Where): Large RFs, spatial features and motion. Primary visual cortex: Small RFs, simple image features such as oriented Object recognition: line segments. Faces. Temporal visual stream (What): Large RFs, complex image features. Model of: V1 orientation elective responses from retina and LGN center-surround responses LGN Primary Retina visual cortex The pupillary reflex Light in one eye Ciliary sphincter muscles Optic nerve Both pupils constrict To contralateral 3rd cranial nerve eye Level of the midbrain 4th ventricle Sensory modalities Amplitude and frequency of sound Hertz Normal audibility curve dB = 20 log (sound pressure / reference pressure ) 160 Pain Pain 140 Discomfort 120 100 Damage threshold Live Rock 80 Heavy Traffic 60 Conversation 40 Threshold 20 Whisper 0 10 100 1000 10,000 Frequency (Hz) afford to turn up the volume. But if you use your MP3 player for hours on end, you'll want to keep the volume lower. According to the study, if the volume is turned as high as it will go (100%), the safe listening time is only five minutes per day using standard earbuds. At 50% volume, on the other hand, most people can safely listen all day. Here's the chart of maximum listening time per day using stock earphones: Volume Level Maximum Listening Time per Day 50% or below No limit 60% 18 hours 70% 4.6 hours 80% 1.2 hours 90% 18 minutes 100% 5 minutes If you use headphones instead of earbuds, adjust the above volume levels by adding 10%. For example, you can listen for 1.2 hours at 90% volume instead of 80% volume. (It won't sound any louder to you; the loudness at your ear will be the same.) Results were quite consistent across several brands and models of MP3 player. The irony is that many people use iPods and similar devices to Clinical Implications: Advancing age and Presbycusis Progressive, bilateral hearing loss with increasing age, mainly for frequencies > 1,000 Hz Anatomy of the ear Middle Pinna ear Malleus, incus & stapes Inner ear External Cochlea auditory canal Eustachian tube Tympanic membrane Anatomy of the inner ear Inner ear Utricle (Horz) Semicircular Saccule (Vert) canals Sensory Oval window epithelia Round window Cochlea USCA Team CNS/Sensory Emma Margie [email protected] Monika Maneva [email protected] CNS/Cogni-ve Motor Brianna Luo [email protected] Ava Charnetski [email protected] Flow of sound energy Amplification (modulated by skeletal muscles) Fluid Sound energy Basilar Cochlear membrane duct Motion of basilar membrane is frequency dependent Basilar membrane Scala vestibuli Local vibrations Scala tympani The basilar membrane in action (voice of Dr. James Hudspeth, Rockefeller University) Provided by Dr. Vollrath Basilar membrane motion is converted into neuronal activity at the organ of Corti Cochlear Scala vestibuli duct Basilar membrane Scala tympani Deflection of basilar membrane produces shearing of hair cell stereocelia Tectorial membrane Stereocilia Inner hair cells Outer hair cells Basilar membrane Stereocilia Stereocilia The Cochlear Amplifier Outer hair cell “electromotility" Hypothesis: Hair cell - Shorten when depolarized electromotility augments - Lengthened when hyperpolarized basilar membrane motion Video by J. Ashmore provided by Dr. Vollrath Clinical implications of outer hair cell “electromotility" Otoacoustic emissions (reflex) are used to evaluate hearing in newborns. Provided by Dr. Vollrath Hair cells contain mechanoreceptors Hair bundle (stereocilia) UtricleStim Cell body Provided by Dr. Vollrath Movement of hair cell stereocilia Dr. David Corey, Harvard Medical School Provided by Dr. Vollrath Tip links connect each stereocilia Provided by Dr. Vollrath Tip links gate ion channels in the stereocilia Stereocilia Mechano-transduction at tip link activates afferent neurons Stereocilia Stereocilia Clinical Implications: Ringing in your ears (Tinnitus) Transient (< 24 hours): - Usually due to loud noise. - Excessive mechanical stress of stereocilia. - Tip-links are thought to break, but eventually grow back (ringing stops). Chronic: - Many causes, but predominately loud noise. - Origin can be either inner ear, nerve or central pathways. - Impacts quality of life (does not stop). Visual versus auditory transduction Visual transduction Auditory transduction Photons: high energy but hard Sound waves: low energy but to catch all around (~100X106 photoreceptors) (~15,000 hair cells) Several hundred thousand tip Trillions of opsin molecules links Slow: G-protein cascade Fast: direct channel activation Amplification: one photon No amplification of the closes many ion channels transduction Cochlear Implant Hair cell loss due to aging, loud sounds, ototoxic drugs: 1) Implanted through round window 2) Electrode placed in scala tympani 3) Electrodes are spaced along the cochlear spiral to stimulate groups of afferent fibers that respond to different frequencies. Generally ~12 electrodes. Central auditory pathways Primary auditory cortex Primary auditory Thalamus cortex Midbrain Medulla 8th cranial nerve (vestibular and auditory) Sensory modalities Vestibular organs Linear acceleration Semicircular Utricle Horizontal canals Saccule Vertical Angular acceleration Vestibular ocular reflex Eyes rotate in the opposite direction Head rotates Gaze does not change Tip links gate ion channels in the stereocilia Stereocilia Organization of semicircular canals Ampula Cupula Stereocilia Stereocilia bend Hair cells Semicircular canal Utricle and saccule detect linear acceleration Motion Otoliths Otoliths lags behind Stereocilia bend Sensory modalities Taste (gustation) Taste afferent Papillae Taste cell Taste pore Taste bud You have about 10,000 taste buds Taste transduction Umami Salty Sour (high acid) Bitter Na+ Block Na+ H+ H+ Glutamate channels receptors G-protein cascade K+ K+ Bitter Sweet Various G-protein cascades G-protein cascade Central taste pathways Ipsilateral gustatory Thalamus cortex Medulla Cranial nerves Sensory modalities Olfaction Olfactory bulb Olfactory nerve Olfactory Olfactory receptor tract cells Nasal cavity Mucus Cilia Nose Olfactory epithelium Olfactory signal transduction 1) Odorant binding Oderant receptors 2) G-Protien activation 3) Opening About 1000 of ion different oderant channel receptors. Central olfactory pathways Limbic system Olfactory bulb