CHM 102 Organic Reaction Mechanisms 2022/2023 PDF
Document Details
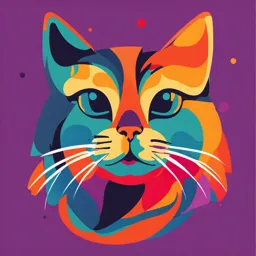
Uploaded by VirtuousConnotation1499
Prof. Olowu R.A
Tags
Related
- AQA Chemistry A-Level 3.3.1 Introduction to Organic Chemistry Notes PDF
- MED-108 Organic Chemistry Reaction Mechanisms PDF
- Chemistry 1B - Lecture 8 Reaction of Organohalides PDF
- AQA Chemistry A-Level 3.3.1 Introduction to Organic Chemistry Detailed Notes PDF
- Organic Chemistry Lecture Notes PDF
- Pharmaceutical Organic Chemistry (1) PDF
Summary
These lecture notes cover organic chemistry concepts, including reaction mechanisms, bond breaking, and enthalpy. The notes describe homolytic and heterolytic fission, reaction types, and calculations related to bond dissociation.
Full Transcript
CHM 102 (General Chemistry II) Lecture: Prof. Olowu R.A Topic: Organic Reaction Mechanism Session: 2022/2023 REACTION MECHANISM: A reaction mechanism is a sequence of two or more steps, each one represented by an equation that shows how a reaction takes place. A mechanism t...
CHM 102 (General Chemistry II) Lecture: Prof. Olowu R.A Topic: Organic Reaction Mechanism Session: 2022/2023 REACTION MECHANISM: A reaction mechanism is a sequence of two or more steps, each one represented by an equation that shows how a reaction takes place. A mechanism tries to explain the actual changes that occur in a reactions that have been carefully studied, and for which there are explanation of exactly how they occur. BOND BREAKING IN ORGANIC REACTION Organic compounds contain covalent bond. For a bond to break, the electrons in the bonding molecular orbital (MO) must receive energy from their surroundings. Specifically, the surrounding molecules must transfer some of their kinetic energy to the system (the bond being broken). The term enthalpy is used to measure this exchange of energy: ∆H = q (at constant pressure) The change in enthalpy (∆H) for any process is defined as the exchange of kinetic energy, also called heat (q), between a system and its surroundings under conditions of constant pressure. For a bond-breaking reaction, ∆H is primarily determined by the amount of energy necessary to break the bond homolytically. There are two ways for the covalent bond to break. These are hemolytic fission and heterolytic fission. Homolytic Fission: This type of bond breaking generates two uncharged species, called radicals, each of which bears an unpaired electron. In contrast, heterolytic fission is a type of bond breaking that generates charged species, called ions. Heterolytic fission usually occurs when the two atoms bonded together have different electronegativities. The atom with the higher electronegativity is the one that keeps both electrons from the bond and bears the negative charge, while the other atom becomes positive charge. Figure 1: Homolytic fission produce two radicals Figure 2: Heterolytic fission produces ions The energy required to break a covalent bond via homolytic bond cleavage is called the bond dissociation energy. The term ∆H° (with the “naught” symbol, or small circle, next to the H) refers to the bond dissociation energy when measured under standard conditions (i.e., where the pressure is 1 atm and the compound is in its standard state: a gas, a pure liquid, or a solid). Table 1 gives ∆H° values for a variety of bonds commonly encountered in organic reaction. Table 1: Bond dissociation energies (∆H°) of common bonds Most reactions involve the breaking and forming of several bonds. In such cases, we must take into account each bond being broken or formed. The total change in enthalpy (∆H°) for the reaction is referred to as the heat of reaction. The sign of DH° for a reaction (whether it is positive or negative) indicates the direction in which the energy is exchanged and is determined from the perspective of the system. The direction of energy exchange is described by the terms endothermic and exothermic. In an exothermic process, the system gives energy to the surroundings (∆H° is negative). In an endothermic process, the system receives energy from the surroundings (∆H° is positive). Figure 3: Energy profile for exothermic and endothermic reactions Class activity: Predict the sign and magnitude of ∆H° for the following reaction. Give your answer in units of kilojoules per mole, and identify whether the reaction is expected to be endothermic or exothermic. Solution: Identify all bonds that are either broken or formed: Now we must decide what sign (+ or -) to place in front of each value. For each bond broken, the system must receive energy in order for the bond to break, so ∆H° must be positive. In contrast, for each bond formed, the electrons are going to a lower energy state, and the system releases energy to the surroundings, so ∆H° must be negative. Total bond breaking = + 624 kJ/mol Total bond formed = - 762 Kj/mol ∆H° = Bond formed + bond breaking -138 kJ/mol. For this reaction ∆H° is negative, which means that the system is losing energy. It is giving off energy to the environment, so the reaction is exothermic.. Practice questions: Using the data in Table 1, predict the sign and magnitude of DH° for each of the following reactions. In each case, identify whether the reaction is expected to be endothermic or exothermic: ENTROPY OF ORGANIC REACTION Entropy is informally defined as the measure of disorder associated with a system, although this definition is overly simplistic. Entropy is more accurately described in terms of probabilities. Entropy is formally defined as a property of matter associated with the degree of disorder or degree of randomness, of particles (i.e. atoms, molecules or ions) and also with the distribution of quanta of energy between the particles. A process that involves an increase in entropy is said to be spontaneous. A spontaneous process the one that takes place without continuous intervention by us. Chemical reactions are no exception, although the considerations are slightly more complex than in a simple free expansion. In the case of free expansion, we only had to consider the change in entropy of the system (of the gas particles). The surroundings were unaffected by the free expansion. However, in a chemical reaction, the surroundings are affected. We must take into account not only the change in entropy of the system but also the change in entropy of the surroundings: ∆Stot = ∆Ssys + ∆Ssurr Where ∆Stot is the total change in entropy associated with the reaction. In order for a process to be spontaneous, the total entropy must be positive. Entropy Change of System: ∆Ssys is calculated using the expression: Entropy Change of Surrounding: Gibbs Free Energy of Organic reaction Gibbs free energy is a quantity that is used to measure the maximum amount of work done in a thermodynamic system when the temperature and pressure are kept constant. Gibbs free energy is denoted by the symbol ∆G. In other words, ∆G can be calculated by using the expression: In order for a process to be spontaneous, ∆G for that process must be negative. Any process with a negative DG will be spontaneous. Such processes are called exergonic. Any process with a positive ∆G will not be spontaneous. Such processes are called endergonic. Figure 4: Energy diagrams for an exergonic and endergonic process Reaction Equilibria in Organic Reaction Consider the energy diagram in Figure 6.9, showing a reaction in which the reactants, A and B, are converted into products, C and D. The reaction exhibits a negative DG and therefore will be spontaneous. Accordingly, we might expect a mixture of A and B to be converted completely into C and D. But this is not the case. Rather, an equilibrium is established in which all four compounds are present. Why should this be the case? If C and D aretruly lower in free energy than A and B, then why is there any amount of A and B present when the reaction is complete? Figure 6: The energy diagram of an exergonic reaction in which reactants (A and B) are converted into products (C and D). When the reaction begins, only A and B are present. As the reaction proceeds, the concentrations of A and B decrease, and the concentrations of C and D increase. As the reaction proceeds, the free energy decreases until it reaches a minimum value at very particular concentrations of reactants and products. If the reaction were to proceed further in either direction, the result would be an increase in free energy, which is not spontaneous. At this point, no further change is observed, and the system is said to have reached equilibrium. The exact position of equilibrium for any reaction is described by the equilibrium constant, Keq, Figure 7: An energy diagram illustrating the relationship between ∆G and concentration. A minimum in free energy is achieved at particular concentrations (equilibrium). If the concentration of products is greater than the concentration of reactants, then Keq will be greater than 1. On the other hand, if the concentration of products is less than the concentration of reactants, then Keq will be less than 1. The term Keq indicates the exact position of the equilibrium, and it is related to DG in the following way, where R is the gas constant (8.314 J/mol · K) and T is the temperature measured in Kelvin: ∆G = -RT ln Keq For any process, whether it is a reaction or a conformational change, the relationship between Keq and G is defined by the equation above. If ∆G is negative, the products will be favored (Keq > 1). If DG is positive, then the reactants will be favored (Keq < 1). In order for a reaction to be useful (in order for products to dominate over reactants), ∆G must be negative; that is, Keq must be greater than 1. Practice Questions: In each of the following cases, use the data given to determine whether the reaction favors reactants or products: (a) A reaction for which ∆G = +1.52 kJ/mol (b) A reaction for which Keq = 0.5 (c) A reaction carried out at 298 K, for which DH = +33 kJ/mol and DS = +150 J/mol # K (d) An exothermic reaction with a positive value for ∆Ssys (e) An endothermic reaction with a negative value for ∆Ssys Types of Reactions in Organic Chemistry There are five basic types of reaction in organic chemistry: 1. Addition reaction 2. Substitution reaction 3. Oxidation reaction 4. Reduction reaction 5. Polymerization reaction 1. Addition Reaction: In addition reaction, two reactant species combine together to form a single species. All the species involved are usually molecules. One example is the reaction between ethane and bromine. C2H4 + Br2 → C2H4Br2 2. Substitution Reaction: In a substitution reaction, two reactants species combine together to form two products. C2H5Br + OH- → C2H5OH + Br- In the reaction above, OH has taken the place of, or substituted the Br atom. 3. Oxidation Reaction: This is a reaction in which a substance gains oxygen or loses hydrogen. The oxygen atom is usually from on oxidizing agent (eg. K2CrO7 or KMnO4) represented by [O]. C2H5OH + [O] → CH3CHO + H2O 4. Reduction Reaction: This is a reaction in which a substance gains hydrogen or loses oxygen. The hydrogen is usually from a reducing agent (eg. NaBH4) CH3CHO + 2[H] → CH3CH2OH 5. Polymerization Reaction: This is a reaction in which two or more smaller molecules combine together to form a larger one. The smaller molecules are called monomer while the larger one is called polymer. A general equation for this reaction is NUCLEOPHILE AND ELECTROPHILE Nucleophile: This a chemical species that forms bonds by donating an electron pair. All molecules and ions with a free pair of electrons or at least one pi bond can act as nucleophiles. Examples of nucleophiles are anions such as Cl− OH- carbanion (C-), or a compound with a lone pair of electrons such as H2O, NH3 (ammonia) and PR3 Electrophile: This is a species that is attracted to a region of high electron density. They are either positively charged or neutral species. Examples include carbocations (C+) and carbonyl compounds. QUESTIONS: SIMPLE MECHANISM OF CHLORINATION OF METHANE In the free radical chlorination of methane, the chain initiation step involves the formation of chlorine radicals. Here, the homolytic cleavage of the Cl2 molecule takes place in the presence of heat or light because the Cl−Cl bond is weaker than C−C and C−H. Hence, Cl−Cl is easier to break and forms chlorine radicals. Chlorination of methane: