CHE 176 - Organic Chemistry I - Lecture Notes PDF
Document Details
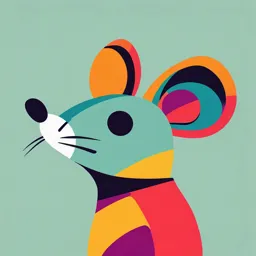
Uploaded by SupremeOnyx5924
O. O. Sonibare
Tags
Summary
These lecture notes cover the fundamentals of organic chemistry, focusing on the unique properties of carbon and its bonding. The material includes discussions on hybridization, drawing diagrams of molecules, types of reactions and also details different functional groups of organic compounds.
Full Transcript
CHE 176 – ORGANIC CHEMISTRY I PROF. O O. SONIBARE OFFICE: F104, CHEMISTRY DEPT. LECTURE OUTLINE Basic concept of organic chemistry – definition and importance of organic chemistry, atom and molecular orbitals including bonding, unique nature of carbon and hybridization...
CHE 176 – ORGANIC CHEMISTRY I PROF. O O. SONIBARE OFFICE: F104, CHEMISTRY DEPT. LECTURE OUTLINE Basic concept of organic chemistry – definition and importance of organic chemistry, atom and molecular orbitals including bonding, unique nature of carbon and hybridization Determination of molecular composition and structure of organic compounds Functional group classes of organic compounds and nomenclature Reaction mechanism and Kinetics Organic reactions- types and factors affecting them. DEFINITION OF ORGANIC CHEMISTRY What is Chemistry? Chemistry is the branch of physical science that deals with the study of composition, properties and reactions of substances. What is Organic Chemistry? Branch of chemistry that deals with carbon compounds. This branch of chemistry is very important because of the large numbers of organic compounds and their occurrences and applications. IMPORTANCE OF ORGANIC CHEMISTRY Most of the substances we use in our daily life are organic in nature: (a) The food types that sustain human body are made up of organic compounds, e.g. carbohydrates, proteins, fats and oils, vitamins etc. (b) the clothes we wear (wool and cotton- cellulose) (c) the commodities we use (wood- cellulose, plastic- polymer of hydrocarbons) (d) the source of primary energy (petroleum, natural gas, coal) (e) The medicine we take (f) In agriculture (fertilizers and agrochemicals) UNIQUE NATURE OF CARBON What makes carbon special? Why is a whole field of organic chemistry dedicated to carbon? Carbon is very unique due to many reasons: 1. Tetravalent nature: Due to its tetravalent nature, carbon always form covalent bonds by sharing electrons with one, two, three or four carbon atoms or atoms of other elements or group of atoms. Carbon can form single, double or triple bonds with other atoms because of its bonding ability. 2. Catenation: The tetra-covalency of carbon atom allows it to combine easily with other carbon to form stable chain like structure. Catenation: Catenation is the ability of an element to form chains and rings in which its atoms are bonded to one another i.e. ability to exhibit self– linkage. 3. Formation of strong C-C covalent bonds: The single bond formed between the carbon atoms is strong. This results in the formation of stable compounds. Carbon atoms also form stable bonds with other atoms e.g. H, Cl, Br, O etc. 3. Formation of C-C multiple bonds: Due to its small size, the C-atom can also form multiple bonds. This gives rise to a variety in the carbon compounds. 4. Hybridisation: Formation of hybrid orbitals allows carbon to form double and triple bonds. ATOMS, ATOMIC AND MOLECULAR ORBITALS – Refer to your CHE 156 Note HYBRIDISATION OF ORBITALS IN CARBON Hybridization simply means mixing Hybridization of orbitals involves mixing of atomic orbitals to form new identical orbitals known as hybrid orbitals. These hybrid orbitals are subsequently used in bonding to give more stable compounds. 3 types of Hybridization exist in carbon: sp3, sp2 and sp All the three types involve mixing of s and p orbitals to form new hybrid orbitals. sp3 HYBRIDIZATION IN CARBON Atomic Number of Carbon = 6 Electronic configuration of Carbon in ground state = 1s2 2s2 2p2 [2p1x 2p1y 2pz], thus implying that valency of carbon should be 2. However, carbon exhibits a valency of 4 i.e. its tetravalent. WHY IS THIS SO? One electron is promoted from the 2s orbital to the vacant 2pz orbital giving rise to four (4) unpaired electrons viz - the new electronic configuration of carbon = 1s2 2s1 2p1x 2p1y 2p1z 1s electrons are considered to be core electrons and are not available for bonding. Furthermore, there is mixing between the 2s orbital and the three 2p orbitals to form four (4) new sp3 hybrid orbitals. This is sp3 hybridisation i.e. mixing of one s orbital with 3p orbitals to form 4 sp3 hybrid orbitals. The sp3 hybrid orbital has the characteristic of both s and p orbitals. The 4 sp3 hybrid orbitals are arranged in a tetrahedral shape i.e. they point towards the corner of a regular tetrahedron as illustrated below. 109.5o H H 109.5 o C H H In CH4 , the 4 sp3 hybrid orbitals overlap with 1s atomic orbital of hydrogen to form 4 equal, covalent sigma C–H bonds resulting in methane [CH4]. The C–H bond is known as sigma (σ) bond. The electron pair [..] between the carbon and the hydrogen (C–H) constitutes a sigma bond which is a covalent bond i.e. carbon donates one electron and hydrogen donates one electron to form the covalent bond between them. + C H sp3 orbital of C 1 s orbital of H C - H bond The 4 C–H bonds point at an angle of 109.5o to each other towards the corners of a regular tetrahedron, hence CH 4 has a tetrahedral shape. H H 109.5 o C H H Carbon uses sp3 hybrid orbitals when it combines with 4 other atoms. The sp3 hybrid orbital may also overlap with other s or p orbitals and hybrid orbitals to form different bonds, e.g. In forming C – C bond, one sp3 hybrid orbital of a C atom overlaps with sp3 hybrid orbital of another C atom to form a molecular orbital. C C Thus, sp3 hybridised orbitals are used in formation of alkanes [Saturated hydrocarbons]. Example 1: Formation of Ethane (C2H6): In the formation of ethane (C2H6), 2sp3 hybridized orbitals overlap to form the C–C bond in ethane (CH3– CH3); while one sp3 hybrid orbital of carbon overlaps with 1s atomic orbital of hydrogen to form the C-H bond. Ethane also has a tetrahedral shape. H H H C C H H H Example 2: In the formation of tetrachloromethane (CCl4), the sp3 hybrid orbital of C overlap with 4 chlorine 3p orbitals to form the 4 C – Cl bonds. Cl : 1s2 2s2 2p6 3s2 3p5 3p Cl Cl C Cl C Cl Cl Thus, carbon is able to form so many compounds. SUMMARY OF sp3 HYBRIDIZATION sp3 hybridization results in: (1) Tetrahedral shape (2) Bond angle of 109.5o (3) Formation of alkanes [i.e. saturated hydrocabons] sp2 HYBRIDIZATION IN CARBON This involves the mixing of one 2s orbital and two 2p orbitals to form three sp2 hybrid orbitals. All sp2 hybrid orbitals lie in a plane and point at an angle of 120o to each other thus giving a TRIGONAL shape. E.g. Formation of ethene [C2H4] C: 1s2 2s1 + 2p 1 2p 1 2pz1 x y 3sp2 hybrid orbitals 1 + 2p 3 (+) 2s orbital 2p orbital sp2 hybrid orbital H H Covalent (bond 120o C C Sp2 H H S Trigonal shape In forming ethene, the sp2 hybrid orbitals of C overlap with the 1s atomic orbital of 2 H atoms and 1sp2 hybrid orbital of another carbon atom to form 3 sigma (σ) bonds. The remaining unhybridised 2pz orbitals on the carbon atoms overlap to form another bond known as the pie (π) bond. bond CH CH C C bond Hence, there are 2 bonds between the 2C atoms of ethene (a sigma bond and a pie bond). The presence of the π bond in ethene makes it more reactive than ethane and accounts for the greater reactivity of alkenes. The C=C (double bond) is shorter than the C–C (single bond) due to the greater closeness from the overlap of the unhybridized 2pz orbitals. Note: C=C bond length = 1.34 Å, C–C bond length = 1.54 Å SUMMARY OF sp2 HYBRIDISATION (1) Trigonal shape [planar molecule] (2) Bond angle of 120o (3) Formation of alkenes sp HYBRIDISATION IN CARBON This is the mixing of one 2s orbital and one 2p orbital to form two sp hybrid orbitals. For example, in the formation of ethyne, the C atom uses the 2 sp hybrid orbitals to form 2 sigma bonds [one between C and C; and one between C and H]. 2px 1 + - (+) 2S 2px sp hybrid orbital 180o [sp + 1s] H C C H [sp + sp The sp hybrid orbitals point at an angle of 180o to each other giving a linear shape. The 2 unhybridized p orbitals on C overlap to form 2 pie (π) bonds, resulting in 3 bonds (triple bond) between the 2 C atoms of ethyne as shown below: H–C≡C–H The 3 bonds thus consist of 1σ and 2π bonds. This makes ethyne more reactive than ethene. Thus alkynes are more reactive than alkenes The C≡C bond (Bond length = 1.2 Ao), is also shorter than the C=C (1.34 Å) bond. sp hybridization is common to all alkynes. SUMMARY of sp HYBRIDISATION (1) Diagonal / linear shape (2) Bond angle of 180o (3) Formation of Alkynes ELECTRONEGATIVITY OF HYBRIDISED ORBITALS The greater the s character of a hybrid orbital, the greater the electronegativity of the orbital relative to the other unhybridized bonding orbitals. Electronegativity scale: sp > sp2 > sp3 180o 120o 109.5o Also, increasing the p character (or decreasing the s character) of the bond decreases the bond angle and vice versa. DETERMINATION OF MOLECULAR COMPOSITION AND STRUCTURE OF ORGANIC COMPOUNDS This involves many steps viz; (1) Isolation and Purification of the compound (2) Qualitative Analysis of the compound which involves: (a) Test for the elements in the compound (b) Test for the functional Groups (3) Quantitative Analysis which includes: (a) Combustion experiments to determine the amount of each element present in the compound (b)Determination of Empirical Formulae (c)Determination of molecular mass/weight (d) Determination of molecular formulae (4) Structure Determination which involves: (a) Use of the information in 1 to 3 above (b) Spectroscopic Analysis – IR, UV, NMR, Mass Spectrometry e.t.c. (c) Preparation of Derivatives e.g. esters (d) Degradation reactions (e) Synthesis. ISOLATION AND PURIFICATION OF THE COMPOUND The organic compound could be isolated from different natural sources: plants, soil, microorganisms etc. Process of isolation and purification involves extraction with different solvents (solvents of different polarities) and various chromatographic techniques e.g. Thin Layer Chromatography (TLC), Column chromatography (CC), Paper Chromatography (PC), High Performance or (Pressure) Liquid Chromatography (HPLC) etc. QUALITATIVE ANALYSIS This involves test for the elements present in the compound and the different functional groups. Test for C and H is unnecessary since most organic compounds contain C and H, (However their presence can be detected (confirmed) by combustion experiment in which the organic compound is heated with copper (II) oxide [CuO]. The C is converted to CO2 and the H to H2O. CO2 turns lime water [Ca(OH)2] milky while H2O turns anhydrous CuSO4 blue and anhydrous Cobalt(II)Chloride [CoCl2] pink. 2CHO + 3CuO Δ 2CO2 + H2O + 3Cu The presence of N, S and Halogens (X) is detected by Sodium Fusion test known as [LASSAIGNE’S] TEST. In this test, the organic compound (small portion) is fused (heated strongly) with small piece of sodium metal in a combustion tube. The products of the fusion reaction are extracted with water and analyzed (tested) accordingly. During fusion, the Nitrogen [N] is converted to sodium cyanide [NaCN], the Sulphur [S] to sodium sulphide [Na2S] and the Halogens [X] to sodium halide [NaX] It is important to use a little excess of the sodium metal to prevent formation of sodium thiocynate [NaCNS] which may hinder detection of the N and S. In the presence of the excess Na, any thiocynate formed is converted to NaCN and Na2S i.e. NaCNS + 2Na Δ NaCN + Na2S At the end of the reaction, the combustion tube and contents are added to water, mixed together and filtered. The filtrate is used to test for the elements. TEST FOR NITROGEN This is the first test to be carried out. To a portion of the filtrate in a test tube, add freshly prepared Iron (II) sulphate [FeSO4] solution. Heat, cool and add few drops of Iron (III) Sulphate solution and dilute H2SO4. A blue or green colouration with or without precipitate indicates presence of CN i.e. (N) in the compound. 6NaCN + FeSO4 → Na2SO4 + Na4Fe(CN)6 Na4Fe(CN)6 + 2Fe2(SO4)3 → 6Na2SO4 + Fe4[Fe(CN)6]3 Prussian blue TEST FOR SULPHUR To a portion of the filtrate in a test tube, add dilute HNO3 to acidify and few drops of lead ethanoate (acetate) solution. A black precipitate of lead sulphide confirms presence of S. Na2S + Pb(OCOCH3)2 → PbS + 2CH3COONa TEST FOR HALOGENS If N and /or S are (is) present, a small portion of the filtrate is heated with dil. HNO3 first in a beaker/ flask to about half its volume. The solution is cooled, then AgNO3 is added. The purpose of heating with dil. HNO3 is to remove the N/S present in the compound as HCN and H2S so as not interfere with the detection of the halide. NaCN + HNO3 Δ HCN + NaNO3 Na2S + 2HNO3 Δ H2S + 2NaNO3 Otherwise, the cyanide and sulphide can react with AgNO3 to give insoluble AgCN and Ag2S which will give a false positive test for the halides. CN- + S2- + 3Ag+ → AgCN + Ag2S If N/S is absent in the compound, dilute HNO3 solution and AgNO3 is added to a portion of the filtrate right away. The different precipitates produced indicate the presence of the halogens. (a) A white precipitate, soluble in excess NH4OH indicates the presence of chloride [Cl-] (b) A cream (light yellow) precipitate slightly soluble in excess NH4OH shows Bromide [Br -]. (c) A yellow precipitate, insoluble in excess NH4OH shows presence of Iodide [I-]. TEST FOR FUNCTIONAL GROUP The various functional groups [viz; alkenes and alkynes, alcohols, alkanals, alkanones, carboxylic acids, esters, amides, phenols, amines, nitriles, nitro compounds aromatic compounds etc can also be tested for (DETAILS LATER). QUANTITATIVE ANALYSIS The goal of quantitative analysis is to know OR determine the relative amount of the different elements present in the organic compound i.e. to determine the percentage of each element present in the compound. Conventional Method of Determination of the Percentage Composition of Elements in an Organic Compound This is outlined below: (a) Determination of Quantity of C and H A known weight of the organic compound is burnt (heated) in excess oxygen to give CO2 and H2O. The CO2 is absorbed in a known weight of soda lime (NaOH) or KOH while the H2O is absorbed in a known weight of magnesium perchlorate [Mg(ClO4)2] or Calcium chloride [CaCl2]. The weight of CO2 and H2O is obtained by difference or subtraction and the weights of C and H can be calculated from these: Wt of Carbon (C) = RAM of carbon × Wt ofCO2 RMM of CO2 i.e. 12 x wt of CO2 44 Wt of Hydrogen (H) = RAM of H2× Wt of H2O RMM of H2O i.e. 2 x wt of H2O 18 RAM – Relative Atomic Mass RMM – Relative Molecular Mass Determination of Quantity of Oxygen The percentage of oxygen is always determined as the difference between 100% and the sum of the percentage values for all other elements present in the compound i.e. %O =100% -(Percentage of all other elements). CALCULATION OF EMPIRICAL FORMULA Empirical Formula (E.F.) is the ratio of the number of atoms of each element present in the compound. It is usually calculated from the percentage composition of the elements. WORKED EXAMPLES Question 1: An organic compound X on quantitative analysis was found to contain 40.0% carbon and 6.7% hydrogen. Calculate its empirical formula. [RAM: H = 1, C = 12, O = 16] Solution: 1st, assume the remaining element is O and calculate its percentage composition by difference or subtraction: % O = 100 – (40.0 + 6.7)= 100 – 46.7= 53.3 % The E.F. can be calculated using a simple table as outlined below: Element Carbon [C] Hydrogen[H] Oxygen[O] %Composition OR Weight 40.0 6.7 53.3 No of Moles 40 6.7 53.3 12 1 16 Relative No of Moles 3.33 6.7 3.33 Divide the moles with the 3.33 = 1 6.7 = 2.01 3.33 = 1 3.33 3.33 3.33 lowest Simplest Mole Ratio 1 2 1 Empirical Formula (E.F.) of compound X = CH2O DETERMINATION OF MOLECULAR MASS AND MOLECULAR FORMULA The molecular formula (M.F.) is the actual number of each kind of element present in one molecule of the compound. M.F = (E.F)n where n = whole number. In order to determine the molecular formula, the molecular mass (MM) of the compound must be known. There are many methods of determining the MM. The modern method of choice is by High Resolution Mass Spectrometry [HRMS]. This technique gives the exact mass of the compound. Once the MF is known, then the structural formula or structure of the compound can be determined. Hence for compound X, If Molecular Mass (MM) = 120, what is the Molecular Formula? MF = (E.F.)n = MM MF of cpd. X = (CH2O)n =120 (12+2+16)n =30n = 120, n=120/30 = 4 MF = (CH2O)4 = C4H8O4 Hence Molecular Formula (MF) of compound X = C4H8O4 Structural formula can then be determined. Question 2: 6.51mg of an organic compound, B gave on combustion 8.36 mg of H2O and 20.47 mg of CO2. Quantitative analysis indicated absence of N,S and halogens. Determine the molecular formula [M.F.] of the compound if the MM is 126. SOLUTION 1st calculate the weight of each element in the compound and their percentage composition. Weight of carbon in sample = 12 × 20.47 = 5.58mg 44 % of Carbon = 5.58 × 100 = 85.7% 6.51 Weight of Hydrogen in sample = 2 × 8.36 = 0.93mg 18 % of H = 0.93 × 100 = 14.3% 6.51 % C + %H = 85.7 + 14.3 = 100%, hence B is an hydrocarbon, it has no oxygen. Empirical Formula(E.F.) Determination Element C H C H % 85.7 14.3 5.58 0.93 composition OR mass Number of 85.7 = 7.14 14.3 = 14.3 5.58 = 0.47 0.93 = 0.93 moles 12 1 12 1 Relative No 7.14 = 1 14.3 = 2 0.47 = 1 0.93 = 2 of moles 7.14 7.14 0.47 0.47 Simplest 1 2 1 2 Mole Ratio Therefore, Empirical formula of cpd. B = CH2 MM = 126 MF = (EF)n = MM MF = (CH2)n = 126 14n = 126 n = 126 = 9, MF = (CH2)9 = C9H18 14 Hence, MF of cpd. B = C9H18 Question 3: Quantitative analysis of a liquid organic compound D, furnished 48.76% C and 8.07% H. No other element was found. Calculate it E.F.? If the MM is 74, what is the MF? SOLUTION % C + % H = 48.76 + 8.07 = 56.83 Therefore, the compound must contain Oxygen to make 100% % O = 100 – 56.83 = 43.17 E.F. Determination Element C H O % composition 48.76 8.07 43.17 No of Moles 48.76 8.07 43.17 12 1 16 Since atoms 4.06 8.07 2.70 combine in whole numbers, Relative No of 4.06 8.07 2.70 simple mole 2.70 2.70 2.70 ratio is Moles multiplied by 2. x2 Simplest Mole Ratio 1.50 2.99 1.0 3.0 5.98 2.0 3.0 6.0 2.0 Empirical Formula of D = C3H6O2 MM = 74 MF = (EF)n = MM MF = (C3H6O2)n = 74 (36+6+32)n = 74 74n = 74, n=1 MF = (C3H6O2)1= C3H6O2 Hence, MF of cpd. D = E.F. = C3H6O2 Note: If on dividing by the smallest no/or calculating simplest mole ratio, fractions are obtained as above, multiply the ratios by small numbers (e.g. 2,3) until a ratio of nearly whole numbers is obtained. This is because atoms combine in whole numbers. Practice questions1. Compound H has a composition of 78.5% C, 8.4% H and 13.1% N. Determine its Empirical formula. If Molecular Mass of H = 107, what is the Molecular Formula? FUNCTIONAL GROUP CLASSES OF CARBON COMPOUNDS A functional group (F.G.) is defined as an atom or group of atoms responsible for the chemical identity/reactions of a compound. It’s a term used to identify organic compounds and differentiate between two or more organic compounds. The different functional groups constitute different homologous series. Homologous series A homologous series is a series of compounds (or family of compounds) having the same general formula (i.e. same functional group) and with its successive members differing by a – CH2 – unit. Members of a homologous series have similar methods of preparation and similar chemical properties. The simplest homologous series/functional group is the Alkanes with the general formula: CnH2n+2 [where n ≥ 1] FUNCTIONAL GROUP CLASSES OF ORGANIC COMPOUNDS Serial Homologous Series General Formula Functional Group Molecular Formula of Examples of Nomenclature No. [F.G.] the 1st Member 1. Alkanes CnH2n+2 [RH] CH4 Methane Where (n ≥ 1) H3C CH3 2. Alkenes CnH2n ( n ≥ 2) C C C2H4 Ethene 3. Alkynes CnH2n-2 ( n ≥ 2) C C C2H2 Ethyne 4. Arenes ArH e.t.c. C6H6 Benzene 5. Haloalkanes CnH2n+1X CH3X e.g. CH3Cl Halomethane e.g. (Alkylhalides) R X X Chloromethane 6. Alkanols (Alcohols) CH3OH Methanol R OH OH 7. Alkoxyalkanes CH3OCH3 Methoxymethane R O R' O (Ethers) (Dimethyl ether) Alkanals O O Methanal 8. R C C HCHO [CH2O] (Aldehydes) H H (Formaldehyde) 9. Alkanones (Ketones) R C O CH3COCH3 Propanone (Acetone) C O R' 10. Alkanoic Acid HCOOH Methanoic acid O O (Carboxylic Acids) R C C (Formic Acid) OH OH 11. AlkylAmines CH3NH2 Methylamine R NH2 NH2 (Amines) 12. Alkyl Nitriles CH3CN Methylnitrile (Methyl (Cyanoalkane or cyanide or Alkylcyanide) R C N C N cyanomethane) Alkyl Alkanoate O O Methyl methanoate 13. C HCOOCH3 (Esters) R C OR' OR' 14. Alkanoyl halide (Acid CH3COX e.g. CH3COCl Ethanoyl halide O O halide) R C C e.g. Ethanoyl chloride X X Alkanamide (Amide) O 15. O HCONH2 Methanamide C R C NH2 NH2 Alkanoic anhydride O Ethanoic anhydride 16. O C (CH3CO)2O (Acid anhydride) R C O O C R C O O NOMENCLATURE OF ORGANIC COMPOUNDS New organic compounds could be named in two different ways: 1. Common Names: These are not based on any logic but given to reflect the origin, history or properties of the compound e.g. methane is known as marsh gas, formic acid from ants (formica – Latin name for ants); vanillin from vanilla; Pejujapo from Jatropha podagrica, Jatrophone from Jatropha curcas etc. 2. Systematic Names: These are derived from an internationally agreed logical system based on the structure of the compound. The systematic nomenclature was first introduced in 1892 (in Geneva, Switzerland) and is controlled by the International Union of Pure and Applied Chemistry (IUPAC), hence the Systematic Nomenclature is also called IUPAC system of nomenclature. SYSTEMATIC NOMENCLATURE General Rules for Naming Acyclic Organic Compounds 1. The longest continuous chain of carbon atoms containing the principal functional group is selected as the parent chain and named accordingly. The following prefixes are used depending on the number of carbon atoms : 2. The carbon atoms in the chain are numbered serially from one end to the other in the order to indicate the position of the substituents/functional groups on the chain. The numbering is done such that the substituents/ functional groups have the least numbers possible. 6 Hex- 7 Hept- 8 Oct- 9 Non- 10 Dec- No of Carbon atoms Prefix 11 Undec- 12 Dodec- 13 Tridec- 14 Tetradec- 15 Pentadec- 16 Hexadec- 17 Heptadec- 18 Octadec- 19 Nonadec- 20 Eicos- [Alkane – eicosane] Note: Cyclic compounds carry the prefix cyclo – before the name of the compound e.g cyclobutane. X C C C C C C C 6 5 4 1 2 3 4 3C C C 4 3 2 1 x C 2 1 (About 3 ways of numbering) 3 1 7 2 5 8 4 6 3. In naming the compound, the number (position) of the substituent prefix (comes before) the name of the substituent and this prefix the name of the parent compound. 4. Where there are more than one substituent on the chain, the names of the substituents are written in alphabetical order i.e. ethyl precedes methyl, bromo precedes chloro, chloro precedes ethyl etc. 2-bromo-3-chlorohexane 5. Where there are more than one functional group, the higher functional group is taken as the parent compound while the others are named as substituents. [i.e. the functional groups are numbered preferentially to the substituents]. Order of preference ( Priority of Functional Groups) Alkanes < Arenes < Nitro < Halo < Alkenes < Alkynes < Ethers < Amine < Alkanols < Alkanones (Ketones) < Alkanals (Aldehyde) < Alkanonitrile < Amides < Acidhalides < Esters < Carboxylic acids < Acid anhydride etc. 6. In writing the name, the names of the compounds are written as one word. Numbers are separated from each other by comas and from substituent names by hyphen (–) and the substituent names prefix the name of the parent compound e.g. (CH3)2C(Cl)CH(Cl)CH2COOH Cl 5 2 OH 4 3 1 Cl O 3,4-Dichloro - 4-methylpentanoic acid. Name/Suffix of the Major Functional Groups Functional Groups Suffix Alkanes -ane Alkenes -ene Alkynes -yne Alkanols -ol Amines -amines Alkanal -al Alkanone -one Alkanoic acid -oic acid Alkanonitrile -nitrile Esters -oate Acid halides -oylhalide Amides -amide EXAMPLES 1. CH3CH2CH(CH3)CH2CH2CH2CH3 2 4 6 7 1 3 5 3 – Methylheptane 1 3 Cl 2. CH2(Cl)CH2CH2CH2(OH) 2 4 HO 4-Chloro-1-butanol or 4-Chlorobutanol O 3. CH3CH2CH(OH)CH2CH(Cl)COOH 5 3 6 4 2 1 OH OH Cl 2-Chloro-4-hydroxyhexanoic acid CH3CH2C(Br2)CH(Cl)CH(C2H5)CHO 4,4-dibromo-3-chloro-2-ethyl-hexanal 1 5 2 4 3 Br 3-Bromocyclopentene CH3CH(OH)CH(OH)CH2CH2Cl 5-chloro-2,3-pentandiol 4 1 5 2 63 6 3 5 2 4 1 1,4-cyclohexadiene OH 5 OH 4 3 Cl 2 1 5-chloro-2,3-pentandiol KINETICS AND MECHANISM OF ORGANIC REACTIONS Chemical kinetics Chemical kinetics is the study of how fast chemical reactions occur. The speed of the reaction is measured by the change in concentration of either the reactants or products with time. Chemical kinetics also sheds light on the reaction mechanisms (how the reaction occurs) Chemical reactions can be classified into three types based on the speed of the reaction: Instantaneous reaction: Reactions that occur within a short period of time (10-13 to 10-16 sec). E.g ionic reactions AgNO3 + NaCl AgCl + NaNO3 Moderate reactions: Reactios which occur not too fast or slow (some minutes to some hours). E.g. Hydrolysis of ester Very slow reaction: Reactions which occur over a long period of time (some months or years). E.g rusting of iron 4Fe + 3O2 + 6H2O → 4Fe(OH)3 Rate of Chemical Reaction Rate of chemical reaction is the ratio of change of concentration (Δc) of either reactant or product with time (Δt). So, the rate of reactions can be defined as decrease in concentration of reactant per unit time or increase in concentration of product per unit time Factors affecting reaction rate Temperature: Generally, as temperature increases, so does the reaction rate. At higher temperature, reaction molecules have more kinetcs energy, move faster and collide more often with greater energy. Concentration of reactants: As the concentration of reactants increases, the rates of collision of the reactant molecules and reaction increase. Catalyst: Catalyst will speed up reaction rate by lowering the activation energy Surface area of solid reactant: Increasing the surface area of a solid reactants will expose more of its particles to attack. This results in an increase in chances of collisions and rate of reaction. Pressure of gaseous reactants or products: Increase in pressure will results in increase in the number of collosions and thus increase in reaction rate. Rate laws - Rate laws are always determined experimentally - Reaction order is always defined in terms of reactant (not product) concentrations - The overall concentration dependence of reaction rate is given in a rate law - A general rate law for a reaction will look like For a reaction: n AB → A + B Rate α [AB] Rate = K [AB] The order of reaction of the equation R = K [AB] is known as 1st Order reaction For a reaction RX + OH- → ROH + X- Rate = K [RX][OH] Order = 2; i.e. this is a 2nd order reaction because the sum of the powers of the reactant concentration is equal to two. REACTION MECHANISM A balanced equation for a chemical reaction indicates what is reacting and what is produced , but reveals nothing about how the reaction actually takes place. Reaction mechanism is the actual sequence of events by which reactants are converted to products. Organic reactions could be one step or multi–step. In actual fact, most reactions involve many steps. The rate determining step is the slowest step in the sequence of steps leading to product formation The N2O2 in the above equation is known as reaction intermediate. Reaction intermediates are formed in one step and then consumed in a later step of the reaction mechanism. Reaction intermediate: Reaction intermediates are chemical species, often unstable and short-lived (however sometimes can be isolated), which are not reactants or products of the overall chemical reaction, but are temporary products and/or reactants in the mechanism's reaction steps. Reaction intermediates are often free radicals or ions. Organic chemical reactions normally involve breaking and forming of bonds: i.e. [AB + CD → AC + BD]. The bonds could be broken in two different ways depending on the electronegativity of the atoms involved. The two methods of bond breaking are referred to as Homolytic and Heterolytic Fission HOMOLYTIC FISSION Homolytic fission takes place between atoms of similar electronegativity. This is fission in which the electron pair [which constitutes the covalent bond] is shared equally between the two atoms sharing the bond. Thus, each atom carries an unpaired electron and is known as a radical. i.e. A–B = A :B [An electron pair = a covalent bond] e.g. Cl2 = Cl–Cl A–B → A. + B. [Radicals] Radical are very reactive species. Homolytic fission is also known as Radical Fission. Chemical reactions involving homolytic fission are known as free radical reactions. Homolytic fission also requires a large amount of energy, thus it takes place at very high temperature or under UV light. HETEROLYTIC FISSION Heterolytic fission takes place between atoms of different electronegativities. It involves the transfer of the electron pair to the more electronegative atom, leading to the formation of charged species or ions. Hence, heterolytic fission is also known as Ionic or Polar Fission. i.e. C – D → C+ + D- [D is more electronegative than C] D- is said to be electron rich while C+ is said to be electron deficient. e.g H+─Cl- → H+ + Cl- NUCLEOPHILES AND ELECTROPHILES Nucleophiles are atoms or group of atoms with a negative (-ve) charge or a lone pair of electrons. They are always electron rich. They are called nucleophiles because they are nucleus loving/seeking (i.e they seek the electron deficient centres). e.g. :NH3, H2O:, -OH, Halide ions [X-] e.g. Cl-, R3N:, e.g. (CH3N:), ROH, HS-, RO-, CH3CO2- etc. Electrophiles: These are atoms or group of atoms which are electron deficient or positively charged. They are called electrophiles because they are electron loving (seeking) i.e. they seek electron rich centres. e.g NO2, H3O+,Br+, FeCl3, RN2+, BF3, CH3C+O, R+ Types of Organic Reactions There are 5 main types of organic reactions: Substitution, Addition, Elimination, Condensation and Rearrangement. 1. SUBSTITUTION OR DISPLACEMENT REACTION This involves direct displacement of an atom or a group of atoms by another atom or group of atoms. e.g., (i) Chlorination of methane (ii) Alkylation of benzene. 2. ADDITION REACTION: This reaction is typical of unsaturated compounds and leads to a decrease in degree of unsaturation. It involves addition across an unsaturated bond and gives rise to a less unsaturated or fully saturated product. In most of these reactions, unsaturated compounds function as a source of electrons i.e. act as nucleophiles. Consequently, they are susceptible to attack by electron deficient species i.e. electrophiles. (3) ELIMINATION REACTION: This reaction involves the removal of atoms or group of atom from two adjacent C atoms to form a multiple bond. e.g (4) CONDENSATION REACTION: This is the reaction in which two or more compounds react to form another compound with the elimination of simple substances like water. e.g 5. REARRANGEMENT REACTION This involves migration of an atom or group of atoms from one site to another within the same molecule. It could involve migration of functional group from one point to the other. FACTORS AFFECTING ORGANIC REACTIONS An organic reaction can be facilitated or inhibited by certain factors. These include: (i) Distribution of electrons around the reaction site (ii) The nature and/or size of the atoms or group of atoms surrounding the reaction site. 1. INDUCTIVE EFFECT This is a permanent polarizing effect in single bonds. The effect is caused initially by a covalent bond between atoms of different electronegativities. The effect which is brought about by the unequally sharing of electrons is transmitted along a chain of sigma bonds. Cδ+−Xδ− If the carbon atom attached to polarizing atom or group is itself attached to other C-atom, the inductive effect is transmitted along the chain, although it tends to be insignificant beyond the two carbon atom. When the group (polarizing) attached withdraw electron with respect to carbon atom, the effect is term NEGATIVE INDUCTIVE EFFFECT (–I). When the group is electron donating, the effect is called a POSITIVE INDUCTIVE EFFECT (+I). E.g the alkyl groups have a +I effect. -I effect: The -I effect is seen around a more electronegative atom or group, and electron density is higher there than elsewhere in the molecule. Electron- withdrawing groups include halogen (X), nitro (−NO2), cyano (−CN), carboxy (−COOH), ester (−COOR) and aryloxy (−OAr) +I effect: The +I effect is observed among the less electronegative atoms of the molecule by electron-releasing (or electron-donating) groups. The alkyl groups ® are usually considered electron-releasing (or electron-donating) groups. 2. MESOMERIC EFFECT The shift of π electrons in multiple bonds towards the more electronegative atom is referred to as mesomeric effect. It is similar to inductive effect in single bonds. it is most common in carbonyl compounds (-C=O) where there is a shift of π electrons towards oxygen. The effect can be transmitted along the chain. The difference between the inductive and mesomeric is that there is no visible shift of electron in inductive but there is a visible shift of electrons in mesomeric effect. 3. STERIC EFFECT This is the effect due to the size of the groups surrounding the reaction site of the molecule. This occurs when certain atoms or group of atom alter or in some cases completely prevent a reaction from taking place, despite favourable electronic conditions. This effect is known steric hindrance and particularly effective when the reaction site is crowded with the large bulky groups. E.g (a) CH3COOH + CH3CH2OH CH3COOCH2CH3 + H2O Note: In (b) above, the –OH is in between the two carbon atom, this makes it less assesible to the ethanioc acids.This hinders the rate of reaction or sometimes prevents the reaction from taking place. In (a) above, the –OH group is at the end, this makes it easy for the CH3COOH to react. ISOMERISM Isomerism is the phenomenon whereby two or more compounds have the same molecular formula, but different physical and chemical properties. The compounds which exhibit isomerism are known as isomers. There are two principal types of isomerism. 1. Structural Isomerism 2. Stereoisomerism. STRUCTURAL ISOMERISM These occur when two or more compounds have the same molecular formulae but different structural formulae. Many types occur, some are; (a)Nuclear or Chain Isomerism: This is concerned with the arrangement of carbon atoms in the molecule. i.e. the structure of the nuclei e.g. C4H10 can be written as butane as butane or 2- methyl propane i.e (isobutene). CH3CHCH3 CH3CH2CH2CH3 CH3 Butane C4H10 2-Methylpropane (b) Position Isomerism: This is exhibited by isomers in the same homologous series. They have the same carbon skeleton but they differ in the position of the functional group. e.g., C3H8O (c) Functional Group Isomerism: This is exhibited by compounds with the same molecular formula but different functional groups. e.g., C3H6O can either be an alkanal or an alkanone. (d) Metamerism Describes the phenomenon whereby different atoms or groups of atoms are linked to the same functional group. The isomers belong to the same homologous series and have the same chemical properties but different structures. E.g, C4H10 can form two types of ethers [ROR]. (e) Tautomerism This occurs when two or more compounds have the same molecular formula but different structures due to the migration of an atom from one part of the molecule to another without leaving the molecule. E.g., ethylacetate E.A.A. This is known as Keto – enol tautomerism [H atom migrates from C to O]. STEREOISOMERISM This occurs when two or more compounds have the same molecular and structural formulae but differ in the spatial arrangement of their atoms i.e. the arrangement of the atoms in space. There are two types Viz; Optical and Geometrical. Optical Isomerism: This occurs when two compounds have the same molecular formulae and structural formulae but one compound is not super imposable on the other. Such compounds are termed as Optical Isomers (enantiomers). Optical isomerism occurs in compounds that has Asymmetric or Chiral carbon atoms/centres. A carbon atom is said to be asymmetric/chiral when it has 4 different substituent. This compound is said to be optically active. Such compounds are able to affect the position of plane polarized light. Such compounds also occur in two forms. These are called object and a mirror images. If the compound is place in the front of a mirror, the mirror image will be different and both will rotate plane polarized in different directions. e.g., CH3CH(OH)COOH [Lactic acid, 2-Hydroxypropanoic acid]. A solution of one enantiomer rotates the plane of polarisation in a clockwise direction. This enantiomer is known as the (+) form. A solution of the other enantiomer rotates the plane of polarisation in an anti-clockwise direction. This enantiomer is known as the (-) form. If the solutions are equally concentrated the amount of rotation caused by the two isomers is exactly the same - but in opposite directions. 50/50 mixture of the two enantiomers is known as a racemic mixture or racemate. It has no effect on plane polarised light. Could you get them to align by rotating one of the molecules? The next diagram shows what happens if you rotate molecule B. Geometrical isomerism: This occurs when there is no free rotation around the carbon atoms, either due to the presence of a multiple bond or the presence of a ring or other steric factors. There are two types of geometrical Isomer Viz; Cis and Trans Isomers. The isomer in which like substituents lie on the same side of the multiple bonds is called Cis- Isomers. When the substituents lie on the opposite sides of the multiple bond, it is called Trans- Isomers. The trans-isomers are always more stable than the Cis-isomers e.g For the cis-Butenedioc acid, the melting point is 139oC and for the trans- Butenedioc acid the melting point is 287oC. The two isomers have different physical and chemical properties.