Transport In Plants PDF
Document Details
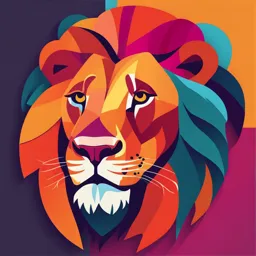
Uploaded by UnbiasedAloe3477
Tags
Summary
This document provides an overview of transport in plants, covering topics such as plant transport systems, water transport (including uptake and movement through the plant), and phloem transport. It explains the importance of these systems for plant survival and growth, using figures and diagrams to illustrate key concepts.
Full Transcript
# Chapter 5: Transport in Plants ## By the end of this chapter you should be able to: - explain the uptake of ions by active transport in roots, and the role of the endodermis - describe the entry of water into plant roots in terms of water potential - relate the structure of xylem vessels to their...
# Chapter 5: Transport in Plants ## By the end of this chapter you should be able to: - explain the uptake of ions by active transport in roots, and the role of the endodermis - describe the entry of water into plant roots in terms of water potential - relate the structure of xylem vessels to their functions in transport and support - make drawings from prepared slides of xylem vessels - relate the structure of sieve tubes and companion cells to their function - make drawings of sieve tubes and companion cells from prepared microscope slides - label pertinent features in an electron micrograph of a sieve tube and companion cell - explain how phloem loading in the leaves occurs against a concentration gradient - outline the ascent of water in plants, including the roles of root pressure, capillarity, cohesion, adhesion and transpiration pull, and the role of stomata in transpiration - investigate the impact of environmental factors, including light and air movements, on the rate of transpiration - discuss mass (pressure) flow as a possible method of translocation, including experimental evidence for and against this hypothesis ## Plant transport systems - Plant cells, like animal cells, need a regular supply of oxygen and nutrients. - All plants are multicellular, and some of them are very large. - Most plants, however, have a much more branching shape than animals, and this provides a much larger surface area: volume ratio for exchange with their environment than in an animal of the same body mass. - The requirements of plants differ from those of animals in several ways, both in the nature of the nutrients and gases required and the rate at which these need to be supplied. **• Carbon dioxide:** Photosynthesising plant cells need a supply of carbon dioxide during daylight. They obtain this from the air. Aquatic plants get carbon dioxide from the water that surrounds them. **• Oxygen:** All living plant cells need oxygen for respiration. Cells that are actively photosynthesising produce more than enough oxygen for their needs. Cells that are not photosynthesising have to take in oxygen from their environment, but they do not respire at such a high rate as mammals and so they do not need such a rapid oxygen supply. **• Organic nutrients:** Some plant cells make many of their own organic food materials, such as glucose, by photosynthesis. However, many plant cells do not photosynthesise and need to be supplied with organic nutrients from photosynthetic or storage cells. **• Inorganic ions and water:** All plant cells require a range of inorganic ions and also water. These are taken up from the soil, by roots, and are transported to all areas of the plant. ## **Uptake of ions** - Plants take up inorganic ions from the solution that is present between soil particles. Ions are absorbed into the root hairs, transported across the root, and then into the xylem. - They then travel, in solution in water, to all parts of the plant. - The structure and function of xylem tissue, and the way in which water moves in it, is described later in the chapter. The mechanism by which ions are taken up by root hairs depends on their concentration in the soil solution. - If a particular type of ion is in a higher concentration in the soil than inside the root hair cell, then it will be absorbed by facilitated diffusion. This does not require any energy input by the plant. - If, however, the concentration of the ion in the soil is lower than that inside the root hair cell, then it must be absorbed by active transport. Specific transporter proteins use energy, derived from the hydrolysis of ATP, to move ions through the cell membrane, into the cytoplasm. ## **Water transport** - The pathway taken by water as it is transported through a plant: Water from the soil enters a plant through its root hairs and then moves across the root into the xylem tissue in the centre. - It then moves upwards in the xylem vessels through the root into the stem and finally into the leaves. ### **From soil into root hair** - The roots of plants have very thin, single-celled extensions of some of the cells that make up the outer layer (epidermis) of the root. These root hairs are a specialised exchange surface for the uptake of water and mineral ions. ## **From root hair to xylem** - Water that has been taken up by the root hairs travels across the cortex and into the centre of the root. It does this because the water potential inside the xylem vessels is lower than that in the root hairs and the cells in between. - Water moves passively down its water potential gradient, from the edge of the root to the xylem in the centre. ### **The apoplast pathway** - The cells of the cortex, like all plant cells, are surrounded by cell walls made of many layers of cellulose fibres criss-crossing each other. Water soaks into these walls rather as it would soak into filter paper, and then seeps across the root from cell wall to cell wall, and through the spaces between the cells, without ever entering a cell. ### **The symplast pathway** - Some water, however, enters the cells and moves from cell to cell by osmosis, or through strands of cytoplasm that make direct connections between adjacent cells, called plasmodesmata. ## **Up through the xylem vessels** - Water is carried from the roots all the way up to the top of the plant inside xylem vessels. In a large tree, the distance from the roots to the topmost leaf could be tens of metres. Yet there is no pump making the water move. ### **The structure of xylem vessels** - Xylem vessels are made of many long, narrow cells called xylem elements stacked end to end. - Xylem elements began as living cells with cytoplasm, nucleus, and cellulose cell walls, but they then differentiated into extremely specialised structures and died. - Xylem elements contain no living material, and are just the empty shells of the cells from which they developed. - Each xylem element has a wall made of cellulose and a substance called lignin. Lignin is a very strong, waterproof material. - The end walls of the xylem elements usually disappear completely, so that the stack of elements makes up a continuous tube, like a drainpipe, reaching all the way from the roots, through the stem and up to the very top of the plant. - There are usually several xylem vessels side by side. Water can move sideways between them, and out of the xylem vessel into the surrounding cells, through pits in their walls. ## **From leaf to atmosphere - transpiration** - The cells in the spongy mesophyll layers are not tightly packed, and there are many spaces around them filled with air. - The water in the cells seeps into the walls, so these cell walls are always wet. - Some of this water evaporates into the air spaces, so that the air inside a leaf is usually saturated with water vapour. - **Factors affecting transpiration:** - Humidity - Temperature - Light Intensity - Air movements - Stomatal aperture - Plant structure - Leaf anatomy ## **Comparing rates of transpiration** - It is not easy to measure the rate at which water vapour is leaving a plant's leaves. This makes it very difficult to investigate directly how different factors, such as light or air movement, affect the rate of transpiration. - However, it is relatively easy to measure the rate at which a plant stem takes up water. - The apparatus used for this is called a **potometer**. It is essential that everything in the potometer is completely watertight and airtight, so that no leakage of water occurs and so that no air bubbles break the continuous water column. ## **Transport in phloem** - The transport of soluble organic substances within a plant is called translocation. - These are substances that the plant itself has made such as sugars, which are made by photosynthesis in the leaves. - The main substance transported in phloem is sucrose. - Assimilates are transported in sieve elements. - Sieve elements are found in phloem tissue, together with several other types of cells including companion cells. **• Sieve elements** - A sieve tube is made up of many elongated sieve elements, joined end to end vertically to form a continuous column. - Each sieve element is a living cell. - They are very narrow, often between 10 and 15µm in diameter. - Like a normal plant cell, a sieve element has a cellulose cell wall, a plasma membrane and cytoplasm containing endoplasmic reticulum and mitochondria. - However, the amount of cytoplasm is very small and only forms a thin layer lining the inside of the wall of the cell. - The end walls of two meeting sieve elements form a sieve plate, which is perforated by large pores. - Perhaps the most striking feature of sieve elements is their end walls. - Where the end walls of two sieve elements meet, a sieve plate is formed - When sieve plates are viewed using a transmission electron microscope in longitudinal section, strands of fibrous protein can sometimes be seen passing through these pores from one sieve element to another. - However, these strands are produced by the sieve element in response to the damage caused when the tissue is cut during preparation of the specimen for viewing. - In living phloem, the protein strands are not present, and so the pores are open and present little barrier to the free flow of liquid through them. **• Companion cells** - Each sieve element has at least one companion cell lying close beside it. - Companion cells have the structure of a 'normal' plant cell, with a cellulose cell wall, a plasma membrane, cytoplasm, a vacuole and a nucleus. - However, the number of mitochondria and ribosomes is rather larger than usual, and the cells are metabolically very active. - Also, the vacuole remains small and does not form a large central vacuole. - Companion cells are very closely associated with the neighbouring sieve elements. - Many plasmodesmata (strands of cytoplasm) pass through their cell walls, providing a direct pathway between the cytoplasm of the companion cell and the cytoplasm of the sieve element. **• Plasmodesmata** - A plasmodesma (plural: plasmodesmata) is a strand of cytoplasm that runs directly from one plant cell to the next. - In a tissue containing closely packed plant cells, each cell may have as many as 10000 plasmodesmata. - The electron microscope shows that the gap in the cell wall is about 25 nm wide at its narrowest point. - The plasma membranes of the cells run right through this gap and are continuous with each other. - The membranes enclose cytoplasm and endoplasmic reticulum. - This arrangement makes it easy for many different types of molecules to pass directly from one cell to the next, without having to cross a membrane. - The presence of these plasmodesmata means that all the cells of a plant are connected, which is a very different situation from that found in animal bodies, where most cells have no large pores in their plasma membranes connecting adjacent cells. - Most pores in animal cells just connect with the extracellular solution. - Small molecules can diffuse freely, but it appears that the pores can, at times, allow larger molecules through, including small proteins. **• The contents of sieve tubes** - The liquid inside phloem sieve tubes is called phloem sap, or just sap. - Phloem sap moves by mass flow. - The flow is always in one direction, and can be both upwards and downwards. - Any area of a plant from which sucrose is loaded into the phloem is called a source. - An area that takes sucrose out of the phloem is called a sink. ## **Loading sucrose into phloem** - Sucrose is soluble, so it dissolves in the water in the cell. - Sucrose is loaded into companion cells by active transport. - This is done in a rather roundabout way. First, hydrogen ions are pumped out of the cell by active transport, using ATP as the energy source. - This creates a large excess of hydrogen ions outside the cell. - They can move back into the cell down their concentration gradient, through a protein that acts as a carrier for both hydrogen ions and sucrose at the same time. - The sucrose molecules are carried through this co-transporter into the companion cell, against the concentration gradient for sucrose. - The sucrose molecules can then move from the companion cell into the sieve tube, through the plasmodesmata that connect them. ## **Unloading sucrose from phloem** - Unloading occurs in any tissue that requires sucrose. - It is likely that the sucrose moves out of the phloem and into the tissue by facilitated diffusion. - Once in the tissue, the sucrose is converted into something else by enzymes. ## **Evidence for the mechanism of phloem transport** - Until the late 1970s and 1980s, there was considerable argument about whether or not phloem sap did or did not move by mass flow, in the way we have described. - The stumbling block was the presence of the sieve pores and phloem protein, as it was felt that these must have some important role. Several hypotheses were put forward, which tried to provide a role for the phloem protein. - It is now known that the phloem protein is not present in living, active phloem tissue, and so there is no need to provide it with a role when explaining the mechanism of phloem transport. - There is now a lot of evidence that phloem transport does occur by mass flow. - The rate of transport in phloem is about 10000 times faster than it would be if substances were moving by diffusion rather than by mass flow. - The actual rates of transport measured match closely with those calculated from measured pressure differences at source and sink, assuming that the pores in the sieve plates are open and unobstructed. ## **Transpiration and climate** - Most of us are aware that cutting down rainforests reduces photosynthesis, increasing the amount of carbon dioxide in the air and contributing to global warming. - But fewer people realise how transpiration can affect climate. - **Changes in land cover** - In the mid-west, the natural vegetation was grassland, but as this has been replaced by agricultural land, the average temperature in those regions has dropped by almost 1°C. - This is because grass does not transpire as much as crops. - The extra transpiration from the crop plants increases the humidity of the air and has a cooling effect. - In contrast, when forest is removed to grow crops, as has happened on the east coast of the USA, the reverse effect is seen. - Forest trees transpire much more than most crop plants, and so the air above the farmland often contains less water vapour than the air above a forest. - As forest has been replaced by farmland, the climate in these regions has become warmer. - There have also been effects on local rainfall, slightly more rainfall where there are forests, compared to areas where forest has been replaced by crops. - But these are much less marked than the temperature changes.