Chapter 3 Neurophysiology Lecture PDF
Document Details
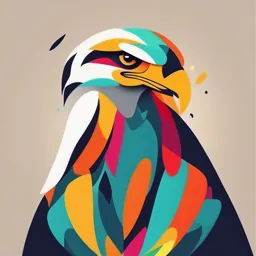
Uploaded by AmusingRhyme2178
University of Florida
Tags
Summary
This document presents a lecture on neurophysiology, focusing on communication within and between neurons. The lecture details topics like membrane potential, action potentials, and the roles of different ions. It appears to be part of a larger biology course.
Full Transcript
Chapter 3: Neurophysiology Communication Within a Neuron - local changes in membrane potential - the action potential Communication Between Neurons - synaptic and non-synaptic communication - integration of inputs 1 Communication Within a Neuron...
Chapter 3: Neurophysiology Communication Within a Neuron - local changes in membrane potential - the action potential Communication Between Neurons - synaptic and non-synaptic communication - integration of inputs 1 Communication Within a Neuron - membrane potential: stored source of energy, the difference in electrical charge across membrane from inside to outside of cell - resting potential: polarized potential, approximately -70 mV in prototypical neuron (varies somewhat) Resting Potentials in different types of mammalian cells Cell types Resting potential Skeletal muscle −95 mV Smooth muscle –60 mV Astroglia –80 to –90 mV Neurons –60 to –70 mV Erythrocytes –9 mV Photoreceptor –40 mV 2 2 Communication Within a Neuron - depolarization: a decrease in membrane potential toward neutrality 0 membrane potential (mV) -20 -40 depolarization -60 -80 ‐60 mV -100 time (msec) - hyperpolarization: an increase in membrane polarization membrane potential (mV) 0 -20 -40 -60 -80 hyperpolarization -100 ‐85 mV time (msec) 3 3 Communication Within a Neuron - action potential: large depolarization and reverse polarization propagated down axon to terminal region - initiated if stimulus is above threshold (approx. -55mV) - invariant magnitude regardless of size of initiating stimulus peak reverse polarization membrane potential (mV) repolarization depolarization threshold failed resting initiations “all-or-none” potential afterhyperpolarization time (msec) 4 4 Communication Within a Neuron - concentration gradient - diffusion: - molecules in constant motion - molecules diffuse to minimize concentration gradient - impermeable or semi-permeable membranes affect distribution of molecules 5 5 Communication Within a Neuron - voltage gradient / electrostatic potential: - electrolytes dissociate into ions in solution - e.g. NaCl dissociates into Na+ (cation) and Cl- (anion) - like forces repel, and opposite forces attract - impermeable or semi-permeable membranes affect distribution of molecules 6 6 Communication Within a Neuron - voltage gradient / electrostatic potential: - ions distributed across the membrane create a “cloud” of charge over the intracellular and extracellular surfaces 7 7 Communication Within a Neuron - the membrane potential and ion distribution. - cations: Na+, K+ - anions: Cl-, proteins - channel proteins: cylindrical proteins that allow controlled exchange of ions 8 8 Communication Within a Neuron - resting potential (-70mV): - Na+ more than 10x more concentrated in extracellular fluid - K+ more than 20x more concentrated in intracellular fluid - [Na+] >>> [K+] - Cl- more concentrated in extracellular fluid - proteins virtually absent in extracellular space, and cannot cross cell membrane Na+ K+ Cl- protein 9 9 Communication Within a Neuron - Sodium-Potassium Pump: Na+ and K+ are actively transported across the membrane by specific Na+/K+ transport proteins - Na+: Na+/K+ pump actively transports 3 Na+ out of the cell - Na+ concentration gradient would push Na+ back in - electrical gradient would push Na+ back in - BUT membrane is almost impermeable to Na+ - K+: Na+/K+ pump actively transports 2 K+ into the cell - K+ concentration gradient would push K+ back out - membrane is semipermeable to K+, so K+ could leak out - BUT electrical gradient keeps K+ inside the cell - extremely energy expensive, uses up to 40% of neurons energy resources 10 10 Communication Within a Neuron - Exchange of ions through membrane: - diffusion of ions limited by cell membrane, but diffusion through channels - electrostatic attraction generates thin “cloud” of charge over inner and outer surfaces 11 11 Communication Within a Neuron - summary of forces on ions at resting potential: Ca2+ Ca2+ 12 12 Communication Within a Neuron Membrane Potential: Electrical and Thermodynamic Forces Determine the Passive Distribution of Ions - using K+ as reference ion (membrane channels somewhat permeable to K+) - outward diffusion due to concentration gradient - held in cell by electrostatic potential - when influx/efflux of K+ ions creates membrane potential (Vm) wherein electrostatic potential balances force of diffusion, the K+ is in equilibrium - equilibrium potential for K+ is approx. -90 to -100mV in most cells - equilibrium potential (E; known as Nernst potential) is calculated by the Nernst equation for any ion for K+, Z = 1, and at 25ºC RT/ZF ≅ 26mV [K+]o = 4mM; [K+]i = 139mM so EK+ = 26mV x ln (4mM/139mM) ≅ -92mV semi-permeable thermodynamic (diffusion) factor membrane electrostatic factor 13 13 Communication Within a Neuron Membrane Potential: Movements of Ions Can Cause Either Hyperpolarization or Depolarization - at equilibrium for a specific ion (e.g. K+), no net flux of the ion species - at -92mV, no exchange of K+ - at -105mV (hyperpolarized), net influx of K+ to partially depolarize cell - at -70mV (depolarized, resting potential), net efflux of K+ to hyperpolarize cell - thus, Nernst potential or equilibrium potential is also known as reversal potential - at potential more positive than -92mV, increasing membrane permeability to K+ will cause K+ efflux to push potential toward -92mV - similarly, at potential more negative than +65mV, ion cytoplasm extracellular Nernst fluid potential increasing membrane permeability to Na+ will K+ 139mM 4mM -92mV cause Na+ influx to push potential toward +65mV - similarly, at potential more positive than -88mV, Na + 12mM 145mM +65mV increasing membrane permeability to Cl- will Cl- 4mM 116mM -88mV cause Cl influx to push potential toward -88mV - Ca 2+ 0.1µM 1.8mM +127mV - similarly, at potential more negative than +127mV, Mg2+ 0.8mM 1.5mM +8mV increasing membrane permeability to Ca2+ will HCO3- 12mM 29mM -22mV cause Ca2+ influx to push potential toward +127mV A- 138mM 9mM 14 14 Communication Within a Neuron Ca2+ Ca2+ 0 membrane potential (mV) -20 -40 EK+ = -92mV -60 -80 -100 -70mV -120 time (msec) K+ channel 15 15 Communication Within a Neuron Ca2+ Ca2+ 0 membrane potential (mV) -20 -40 EK+ = -92mV -60 -80 -100 -92mV -120 time (msec) K+ channel 16 16 Communication Within a Neuron Ca2+ Ca2+ 0 membrane potential (mV) -20 -40 EK+ = -92mV -60 -80 -100 -100mV -120 time (msec) K+ channel 17 17 Communication Within a Neuron Ca2+ Ca2+ 0 membrane potential (mV) -20 -40 ENa+ = +65mV -60 -80 -100 -70mV -120 time (msec) Na+ channel 18 18 Communication Within a Neuron Ca2+ Ca2+ 0 membrane potential (mV) -20 -40 ENa+ = +65mV -60 -80 -100 -100mV -120 time (msec) Na+ channel 19 19 Communication Within a Neuron Ca2+ Ca2+ 0 membrane potential (mV) -20 -40 ENa+ = +65mV -60 -80 -100 0mV -120 time (msec) Na+ channel 20 20 Communication Within a Neuron Ca2+ Ca2+ 80 membrane potential (mV) 60 40 ENa+ = +65mV 20 0 -20 +65mV -40 time (msec) Na+ channel 21 21 Communication Within a Neuron Ca2+ Ca2+ 0 membrane potential (mV) -20 -40 ECl- = -88mV -60 -80 -100 -70mV -120 time (msec) Cl- channel 22 22 Communication Within a Neuron Ca2+ Ca2+ 0 membrane potential (mV) -20 -40 ECl- = -88mV -60 -80 -100 -88mV -120 time (msec) Cl- channel 23 23 Communication Within a Neuron Ca2+ Ca2+ 0 membrane potential (mV) -20 -40 ECl- = -88mV -60 -80 -100 -100mV -120 time (msec) Cl- channel 24 24 Communication Within a Neuron Ca2+ Ca2+ 0 membrane potential (mV) -20 -40 ECa2+ = +127mV -60 -80 -100 -70mV -120 time (msec) Ca2+ channel 25 25 Communication Within a Neuron Ca2+ Ca2+ 0 membrane potential (mV) -20 -40 ECa2+ = +127mV -60 -80 -100 -100mV -120 time (msec) Ca2+ channel 26 26 Communication Within a Neuron Ca2+ Ca2+ 0 membrane potential (mV) -20 -40 ECa2+ = +127mV -60 -80 -100 0mV -120 time (msec) Ca2+ channel 27 27 Communication Within a Neuron 28 28 Communication Within a Neuron - why maintain energy-expensive resting potential? - by maintaining high Na+ concentration gradient and electrochemical potential, neuron is prepared to exert very rapid and powerful response when required - THE ACTION POTENTIAL - electrochemical input from dendrites/soma arrives at axon hillock - if above threshold, action potential is initiated - size/shape of action potential are independent of suprathreshold stimulus intensity voltage-gated sodium channels 29 29 Communication Within a Neuron - voltage-gated sodium channels - axon hillock has highest abundance voltage-gated sodium channels - Voltage-gated Na+ channels open if the threshold depolarization is reached -70mV -55mV voltage-gated Na+ channel (closed) voltage-gated Na+ channel (open) 30 30 Communication Within a Neuron - Wrong, wrong, wrong, wrong, wrong, wrong, wrong, wrong, wrong, wrong, wrong, wrong, wrong, wrong, wrong, wrong, wrong, wrong, wrong, wrong, wrong, wrong, wrong, wrong, wrong, wrong, wrong, wrong, wrong, wrong, wrong, wrong, wrong, wrong, wrong, wrong. refractory period absolute relative 8th ed pg. 68 9th ed pg. 71 10th ed pg. 75 31 31 Communication Within a Neuron - voltage-gated ion channels: membrane channels open or close according to value of membrane potential; when specific ion channels open, ions move according to concentration gradient and electrostatic potential - at -70 to -55 mV: - some Na+ channels open, allowing only small Na+ influx - some K+ channels open, allowing small K+ efflux - at -55 mV to +40 mV: - Na+ channels open at -55 mV, allowing large Na+ influx - K+ channels open later, allowing K+ efflux - rapid depolarization - at +40 mV to -70 mV: - Na+ channels close at about +40 mV and become refractory - by now, all K+ channels open, allowing K+ efflux - rapid repolarization - afterhyperpolarization: - Na+ channels reset can trigger another action potential - K+ channels close, but excess extracellular K+ causes hyperpolarization - excess K+ can diffuse - Na+/K+ transporter eventually restores ionic balance (not very important for individual action potential) 32 32 Communication Within a Neuron - voltage-gated ion channels: membrane channels open or close according to value of membrane potential; when specific ion channels open, ions move according to concentration gradient and electrostatic potential - at -70 to -55 mV: - some Na+ channels open, allowing only small Na+ influx - some K+ channels open, allowing small K+ efflux - at -55 mV to +40 mV: - Na+ channels open at -55 mV, allowing large Na+ influx - K+ channels open later, allowing K+ efflux - rapid depolarization - at +40 mV to -70 mV: - Na+ channels close at about +40 mV and become refractory - by now, all K+ channels open, allowing K+ efflux - rapid repolarization - afterhyperpolarization: ABS REL - Na+ channels reset can trigger another action potential - K+ channels close, but excess extracellular K+ causes hyperpolarization - excess K+ can diffuse, cleared by astrocytes - Na+/K+ transporter maintains ionic balance (not very important for individual action potential) 33 33 Communication Within a Neuron - action potential is propagated along axon - propagated action potential retains intensity of signal - constantly renewed at each voltage-gated Na+ channel or node of Ranvier - depolarization around action potential initiates new action potential 34 34 Communication Within a Neuron - speed of conduction varies - thin unmyelinated → less than 2 m/s - thick unmyelinated → approx 10 m/s - thin myelinated → approx 5-35 m/s - thick myelinated → approx 80-120 m/s - motor neurons → approx 50 m/s - sensory neurons → approx 45 m/s - pain Aδ fibers → approx 5-35 m/s - pain C fibers → less than 2 m/s (mechanical, thermal, and chemical) pain (mechanical and thermal) proprioception pain touch 35 35 Communication Within a Neuron - saltatory conduction in myelinated axons - action potential cannot regenerate at myelin due to insulation, and lack of voltage- gated Na+ channels - positive charges repel to next node - action potential re-established at next node 36 36 Communication Within a Neuron - uni-directional propagation of the action potential - absolute refractory period prevents rebound 37 37 Communication Within a Neuron - uni-directional propagation of the action potential - absolute refractory period prevents rebound 38 38 Communication Within a Neuron - graded potentials in interneurons - interneurons lack axon or have short axon without voltage-gated channels - depolarize or hyperpolarize in proportion to the intensity of stimulus - release neurotransmitter in graded manner - alterations in membrane potential decay rapidly as they are conducted 39 39 Communication Between Neurons - Charles Scott Sherrington – Discovery of the Synapse - (1906) demonstrated gaps between neurons, behaviorally - studied the leg flexion reflex in a dog - measured conduction velocity in sensory & motor neurons - measured distance of input to spinal cord - measured distance of output to muscle - pinched foot, measured delay until flexion - found delay longer than expected - reasoned gaps between neurons - called gaps “synapses” (after Cajal) pinch 40 m/sec S M muscle ~15 m/sec S I M muscle 40 40 Communication Between Neurons - Charles Scott Sherrington – Discovery of the Synapse - 3 conclusions: 1) Reflexes are slower than conduction along an axon. Consequently, there must be some delay at synapses 2) Several weak stimuli presented at slightly different times or slightly different locations produce a stronger reflex than a single stimulus does. Therefore, the synapses must be able to summate stimuli 3) When one set of muscles is excited, flexor another set is relaxed. Accordingly, the input can simultaneously excite outputs at some synapses while inhibiting outputs at other synapses extensor 40 m/sec S M muscle ~15 m/sec S I M muscle 41 41 Communication Between Neurons - Otto Leowi – Discovery of Chemical Neurotransmission - (1921) demonstrated neurons transmit using a chemical messenger - stimulated frog vagus nerve - transferred bath from stimulated heart to second heart - both hearts decreased rate of beating 42 42 Communication Between Neurons - structure of the synapse: - cleft = approx 200 Å - neuronal average = 10,000 synapses Golgi apparatus 43 43 Communication Between Neurons - axodendritic synapses - axosomatic synapses - axoaxonic synapses - dendrodendritic synapses 44 44 Communication Between Neurons - axodendritic synapses - axosomatic synapses 45 45 Communication Between Neurons - axoaxonic synapses - dendrodendritic synapses 46 46 Communication Between Neurons - Type I synapses provide depolarizing input - located primarily on dendritic spines and shafts - round vesicles - thick postsynaptic density - narrow cleft - Type II synapses provide hyperpolarizing input - located primarily on soma - flattened (ellipsoidal) vesicles - thin postsynaptic density - narrow cleft Type I Type II Type I Type II 47 47 Communication Between Neurons - varicosities: beadlike swellings along axon where NT is released; may form synapse or leak NT to extracellular space - ectopic transmission: axonal varicosities leak NT outside of traditional synapses - en passant: axonal shaft forms synapse that is not at the axon terminal 48 48 Communication Between Neurons - gap junctions (electrical synapses): ion channels communicate directly between cells - common in invertebrates membranes gap junction - less common in vertebrates - functions largely unknown in vertebrates - may participate in plastic processes such as sensitization 49 49 Communication Between Neurons - neuromuscular junction: very highly specialized and very highly efficient synapse between a motor neuron and a muscle fiber 50 50 Communication Between Neurons - nonsynaptic chemical communication: in addition to prototypical synaptic receptors, neurons have extra-synaptic membrane-bound receptors - these non-synaptic receptors bind a variety of neurotransmitters, neuromodulators, and hormones - most non-synaptic membrane-bound receptors are metabotropic, some are ionotropic 51 51 Communication Between Neurons - nonsynaptic chemical communication: neurons also have cytosolic and nuclear receptors - cytosolic and nuclear receptors bind a variety of hormones - all known cytosolic and nuclear receptors are metabotropic 52 52 Communication Between Neurons - neurons exhibit basal rate or spontaneous activity - product of intrinsic properties and basal input levels - EPSPs and IPSPs influence firing rate of neurons basal or spontaneous firing rate excitatory input inhibitory input 53 53 Communication Between Neurons - the Rate Law - a characteristic of a physical stimulus (e.g. intensity of the stimulus) is represented by rate of firing of action potentials 54 54 Communication Between Neurons - two main classes of receptors: ionotropic and metabotropic - ionotropic receptors open an ion channel when neurotransmitter binds (usually 2 or more molecules) - metabotropic receptors activate chemical signaling mechanisms when neurotransmitter binds (usually 2 or more molecules) 55 55 Communication Between Neurons - two main classes of receptors: ionotropic and metabotropic - ionotropic receptors open an ion channel when NT binds (usually 2 molecules) - Na+ channels = EPSP - K+ channels = IPSP - Cl- channels = IPSP - Ca2+ channels = EPSP 56 56 Communication Between Neurons - two main classes of receptors: ionotropic and metabotropic - metabotropic receptors activate G protein or other biochemical signal, to open or close an ion channel or initiate changes in biochemical activity when NT binds 57 57 Communication Between Neurons - ionotropic vs. metabotropic receptors - ionotropic = rapid onset, transient actions - metabotropic = slow onset, enduring actions 58 58 Communication Between Neurons - summation of EPSPs and IPSPs - excitatory and inhibitory inputs diffuse along interior surface of cell membrane 59 59 Communication Between Neurons - summation of EPSPs and IPSPs - excitatory and inhibitory inputs diffuse along interior surface of cell membrane, summate (or cancel) and net potential registered at axon hillock may initiate action potential 60 60 Communication Between Neurons - spatial summation: two or more simultaneous inputs arrive at postsynaptic neuron A EPSP B EPSP C IPSP 61 61 Communication Between Neurons - temporal summation: a single input provides repeated stimulation in rapid succession A EPSPs 62 62 Communication Between Neurons - signaling between neurons is foundation of information processing - convergence allows summation of inputs - divergence distributes information to relevant system 63 63 Communication Between Neurons Seven steps of neurotransmission 1) neurotransmitter synthesis 2) packaging & storage 3) release 4) binding to autoreceptors 5) binding to postsynaptic receptors 6) reuptake 7) enzymatic degradation 64 64 Communication Between Neurons Seven steps of neurotransmission 1) neurotransmitter synthesis: - peptide neurotransmitters synthesized from DNA template in soma - altered by enzymes after synthesis - other neurotransmitters synthesized by modification of ingested substances - may be manufactured right in axon terminal - energy for these actions is provided by chemical reactions in mitochondria 65 65 Communication Between Neurons 2) neurotransmitter storage: - vesicular packaging occurs in golgi apparatus in cell body or in axon terminal - some vesicles are further packaged into storage granules that hold many vesicles - any neurotransmitter that leaks from vesicles is destroyed by catabolizing enzymes 66 66 Communication Between Neurons 3) neurotransmitter release: - action potentials cause vesicles to migrate toward presynaptic membrane and to fuse with membrane - action potentials cause vesicles to open and release neurotransmitters into synapse 67 67 Communication Between Neurons 3) neurotransmitter release (cont’d…) - released neurotransmitter diffuses passively across synapse - Ca2+-dependent process 68 68 Communication Between Neurons 3) neurotransmitter release (cont’d…) - “kiss-and-run” vs. “merge-and-recycle”, bulk endocytosis 69 69 Communication Between Neurons 3) neurotransmitter release (cont’d…) - 3 pools of neurotransmitter vesicles: readily releasable, recycling, reserve pools 70 70 Communication Between Neurons 4) neurotransmitters can bind to autoreceptors: - released neurotransmitters can bind to autoreceptors on presynaptic membrane to inhibit further synthesis and release 71 71 Communication Between Neurons 5) neurotransmitters can bind to postsynaptic receptors: - usually 2 or more molecules of neurotransmitter needed - some degree of neurotransmitter → receptor selectivity - postsynaptic response determined by receptor - effect from releasing one vesicle of neurotransmitter on postsynaptic neuron very small – a quantum effect - many quanta required to significantly alter activity of postsynaptic neuron 72 72 Communication Between Neurons 6) Neurotransmitter reuptake: - released neurotransmitters are reuptaken into the presynaptic neuron by specialized transporter proteins (e.g. DAT, NET, SERT) - acetylcholine broken down and choline reuptaken - glutamate reuptake mostly by glia, modified, returned to glutamatergic neurons - no reuptake of peptide neurotransmitters 73 73 Communication Between Neurons 7) enzymatic degradation: - released neurotransmitters are degraded by specific extracellular enzymes - most are excreted as waste products - acetylcholine broken down and choline reuptaken 74 74