Synaptic and Neuromuscular Junction Transmission 2024 PDF
Document Details
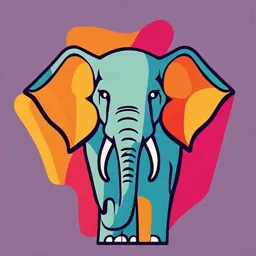
Uploaded by IllustriousPlumTree
Jabir Ibn Hayyan Medical University
Muhsin Alnajmi
Tags
Related
- Tema 3. Comunicación neural PDF
- Neurophysiology II – Action Potentials and Synaptic Transmission PDF
- Neurophysiology II – Action Potentials and Synaptic Transmission PDF
- Neurophysiology Part 3 - Synaptic Transmission and Action Potentials PDF
- 6th Lecture PDF - Synaptic Transmission & Neurophysiology
- Neurophysiology I: Nerve Cell Function/Synaptic Transmission - Lecture 2 PDF
Summary
These notes cover synaptic and neuromuscular junction transmission, including the different types of synapses, neurotransmitters involved, and the sequence of events during transmission. Useful for understanding communication between neurons and muscles. Contains anatomical and physiological information about the neuro systems.
Full Transcript
NEUROPHYSIOLGY Synaptic and neuromuscular junction transmission Department Of Physiology 2nd stage MUHSIN ALNAJIM CONSUSLTANT IN NEUROLOGY A synapse is the region where the axon, or another part of one neuron (the presynaptic neuron), connects...
NEUROPHYSIOLGY Synaptic and neuromuscular junction transmission Department Of Physiology 2nd stage MUHSIN ALNAJIM CONSUSLTANT IN NEUROLOGY A synapse is the region where the axon, or another part of one neuron (the presynaptic neuron), connects with and transmits signals to the dendrites, soma, or axon of another neuron (the postsynaptic neuron). When a neuron communicates with an effector organ (such as a muscle or gland), this specialized synaptic region is referred to as the neuroeffector junction. Neuron-to-neuron communication occurs across either a chemical or an electrical synapse. When a neuron terminates on a muscle, the connection is properly called a neuromuscular junction rather than a synapse. Transmission from a nerve to a muscle resembles chemical synaptic transmission from one neuron to another. The contacts between autonomic neurons and smooth and cardiac muscle or glands are less specialized and transmission in these locations is a more diffuse process. Each neuron forms over 2,000 synaptic connections, making neuron communication highly complex. Synapses change in number and complexity with use and experience. The minimum time for transmission across a synapse is 0.5 ms, known as synaptic delay, which occurs due to the time needed for neurotransmitter release and action on the postsynaptic neuron. As a result, conduction is slower in chains with many synapses (polysynaptic) compared to those with fewer (monosynaptic or oligosynaptic). Synaptic function and transmission Each synapse consists of three parts: 1. Presynaptic terminal 2. Synaptic cleft (20–40 nm wide) 3. Postsynaptic density – an organized structure of receptors, binding proteins, and enzymes that mediate postsynaptic effects. The presynaptic terminal contains numerous mitochondria and membrane- enclosed vesicles filled with neurotransmitters There are three kinds of synaptic vesicles: *small, clear synaptic vesicles contain acetylcholine, glycine, GABA, or glutamate; *small dense-core vesicles contain catecholamines; and *large dense-core vesicles contain neuropeptides. Synaptic vesicles & their proteins are made in the neuron’s cell body. They are transported down the axon to the nerve endings via fast axoplasmic transport. Vesicles release neurotransmitters through exocytosis by briefly fusing with the cell membrane. After releasing their contents, vesicles are refilled through endocytosis. Sometimes vesicles enter endosomes before being reused. More commonly, vesicles follow the kiss-and-run discharge process, releasing contents through a small opening and quickly resealing. Voltage-gated Ca2+ channels are located near the release sites at the active zones. When Ca2+ enters the presynaptic neuron, it triggers exocytosis of neurotransmitters within 200 μs. Transmitters must be released close to the postsynaptic receptors for effective communication. Synaptic organization is supported by neurexins (presynaptic cell-adhesion molecules) that bind to neuroligins on the postsynaptic membrane. Neurexin-neuroligin interactions help hold synapses together and create synaptic specificity, ensuring precise communication between neurons. Some deadly toxins are zinc endopeptidases that block neurotransmitter release by inactivating proteins in the fusion-exocytosis complex. Neurotoxins from the bacteria Clostridium tetani and Clostridium botulinum can disrupt neurotransmitter release in the central nervous system (CNS) or at the neuromuscular junction. Synaptic electrical transmission https://youtu.be/zHJ3h675nNk A single stimulus to sensory nerves usually doesn’t cause an action potential. Instead, it causes a brief depolarization (excitatory) or hyperpolarization (inhibitory). The depolarization (fast excitatory postsynaptic potential,EPSP) starts 0.5 ms after the stimulus, peaks in 1–1.5 ms, and makes the neuron more excitable. An inhibitory response (fast inhibitory postsynaptic potential (IPSP)) happens when Cl− ions enter the neuron. When Cl− enters, the neuron becomes more negative, which lowers the chances of firing an action potential. IPSPs are hyperpolarizations and can be caused by changes in different ion channels. Example: Opening K+ channels (K+ moves out of the cell) or closing Na+ or Ca2+ channels. Slow EPSPs and IPSPs occur in autonomic ganglia, cardiac and smooth muscle, and cortical neurons. These have a delay of 100–500 ms and can last several seconds. Slow EPSPs are caused by a decrease in K+ conductance. Slow IPSPs are caused by an increase in K+ conductance. Electrical synapses In electrical synapses, the presynaptic and postsynaptic neurons are closely connected. They form gap junctions, which allow ions to pass freely between the neurons through low-resistance pathways. This setup lets the EPSP in the postsynaptic neuron happen faster than in chemical synapses, due to the direct ion flow. Presynaptic inhibition and fascilitation Presynaptic inhibition lowers the amount of neurotransmitter released, while presynaptic facilitation increases it. In presynaptic inhibition, one neuron connects to another through a special link called an axoaxonal synapse. This opens Cl− channels, which weakens the signal (action potential). Because of this, less Ca2+ enters, so fewer neurotransmitters get released. K+ channels also open, letting K+ out, which further reduces the amount of Ca2+ and lowers neurotransmitter release. The first neurotransmitter found to cause presynaptic inhibition was GABA. GABAA receptors increase Cl− conductance to cause inhibition. GABAB receptors, found in the spinal cord, increase K+ conductance via a G- protein. Baclofen, a GABAB agonist, helps treat spasticity from spinal cord injuries and multiple sclerosis when delivered through an implanted pump. Other neurotransmitters also cause presynaptic inhibition by affecting Ca2+ and K+ channels via G-proteins. Presynaptic facilitation happens when the signal (action potential) lasts longer, keeping Ca2+ channels open longer, increasing neurotransmitter release. Neuromuscular junction The neuromuscular junction is where a motor nerve connects to a skeletal muscle fiber. As the nerve gets close to the muscle, it loses its myelin covering and splits into several endings (called terminal boutons). These endings contain tiny vesicles filled with acetylcholine, the chemical that sends signals to the muscle. The endings fit into small folds on the muscle’s motor endplate (a thickened part of the muscle membrane). Each muscle endplate is controlled by one nerve fiber. The space between the nerve ending and the muscle is like the synaptic cleft found between two neurons. Together, this structure is called the neuromuscular junction. Sequence of events during transmission https://youtu.be/CLS84OoHJnQ 1. When a nerve impulse reaches the motor neuron terminal, the permeability to Ca2+ increases. 2. Ca2+ enters the nerve endings, causing a large release of acetylcholine from synaptic vesicles via exocytosis. 3. The released acetylcholine diffuses across the synaptic cleft and binds to nicotinic receptors on the motor endplate. 4. When acetylcholine binds, it increases the flow of Na+ and K+ across the membrane. 5. The influx of Na+ causes a depolarizing potential, known as the endplate potential, which triggers muscle contraction. The local depolarization (endplate potential) spreads to the surrounding muscle membrane, reaching the firing threshold. Action potentials are generated on both sides of the endplate and travel along the muscle fiber in both directions. These action potentials trigger muscle contraction. Afterward, acetylcholine is broken down by acetylcholinesterase, an enzyme that is highly concentrated at the neuromuscular junction, clearing it from the synaptic cleft